1 Introduction
The kinetics and mechanism of oxidation of l-ascorbic acid (H2A) to dehydroascorbic acid (A) with different oxidizing agents [1–23] have been investigated. Also, an emphasis has been given to metal-ion catalyzed oxidations by molecular oxygen [13]. These oxidation studies of ascorbic acid are of immense importance both from biochemical [1–25] and biomedical [26–29] viewpoints, highlighting wider applications of the former as a reducing agent in chemical as well as biological systems [1–35]. Ascorbic acid is a water-soluble vitamin for which no microbiological test is known [36]. It has been employed in photo conversion reactions [37,38] and also as an anti-oxidant in food industry [39,40].
Much has been reported on the reactions of cobalt(III) complexes [30,31,41,42] with ascorbic acid despite the fact that these oxidants are substitution inert. Most of these reactions are reportedly outer-sphere and generally occur through an ion-pair as an intermediate, the latter undergoes redox-decomposition. A few reactions are also reported to be inner-sphere [43–46] or mixed inner-sphere and outer-sphere [47,48].
Ascorbic acid, being a lactone having 2,3-endiol group, has also been utilized in a number of synthesis.
These were the observations that prompted us to undertake the kinetics study of the title reaction from the following viewpoints:
- • First, ascorbic acid reportedly undergoes one-electron and two-electron transfer reduction. If it behaves as one-equivalent reductant [22,53], there is every possibility of the formation of the peroxomonosulphate radical which hitherto is least known in its redox reactions.
- • Secondly, transition metal-ion catalyzed oxidations of ascorbic acid by H2O2 have been reported without any agreement for specific rate; the title study becomes more interesting to probe further.
2 Experimental
2.1 Material/Methods
l-ascorbic acid (BDH AnalaR) was employed as received. The solutions of the acid were always prepared afresh as and when required and were kept in refrigerated brown colored glass vessels to minimize deterioration/oxidation. The concentration of the solution was checked iodimetrically [49,50] before use. Peroxomonosulphate (Aldrich) was employed as supplied. Other reagents were of analytical grade. Copper sulphate was employed as a catalyst.
Triply distilled water was employed in the preparation of the reagents and reaction mixtures, the third distillation was from edta of double distilled water in alkaline KMnO4 in an all glass apparatus. Such a distillation with edta removed traces of metal-ions known to catalyze the oxidation of ascorbic acid by molecular oxygen or peroxides.
2.2 Procedure
The reaction mixtures containing all other ingredients except peroxomonosulphate were taken in glass stoppered Erlenmeyer flasks painted black from the outside to check photodecomposition. These flasks were suspended in a water-bath thermostated at ± 0.1 °C unless specified otherwise. The reactions were initiated by adding requisite solution of the temperature pre-equilibrated peracid in to the reaction mixture. The time of initiation was recorded when half of the contents from the pipette were released into the reaction mixture. A known aliquot (5 or 10 cm3) of the reaction mixture was withdrawn periodically and then quenched in an ice-cold H2SO4 (∼1 mol dm−3), the remaining ascorbic acid at different time intervals was estimated ceremetrically employing n-phenyl anthranilic acid indicator.
Initial rates were computed employing plane-mirror method. Pseudo first order plots were also made wherever reaction conditions permitted and second order plots were made for comparable concentrations of the reactants. Rates in triplicate were reproducible to within ± 5%.
2.3 Stoichiometry
The reaction mixtures with [PMS] > [H2A] and [H2A] > [PMS] were allowed to react in a thermostated water-bath for 6 h, excess [PMS] was determined iodometrically (heretofore PMS was used for peroxomonosulphate). The reactions where excess [H2A] was taken over [PMS], the excess [H2A] was determined iodimetrically. These results subscribe to the reaction of one mole of PMS with one mole of ascorbic acid as represented by Eq. (1).
A similar stoiochiometry has been reported earlier in reactions of ascorbic acid with peroxodisulphate, peroxodiphosphate and peroxomonophosphate, respectively, in acid media.
3 Results
3.1 Uncatalyzed reaction
3.1.1 Peroxomonosulphate dependence
The concentration of peroxomonosulphate was varied in the range (2.0 to 10.0) × 10−3 mol dm−3 at fixed concentrations of other reaction ingredients viz. [H2A] = 2.0 × 10−3 mol dm−3 and [H+] = 0.02 mol dm−3 employing perchloric acid. Initial rates (ki, mol −3 s−1) were calculated and a plot of initial rate (ki) against the concentration of PMS yielded a straight line passing through the origin indicating first order with respect to PMS.
3.1.2 Ascorbic acid dependence
The concentration of ascorbic acid (H2A) was varied from (2.0 to 10.0) × 10−3 mol dm−3 at constant concentrations of other reaction components viz. [PMS] = 5.0 × 10−3 mol dm−3 and [H+] = 0.02 mol dm−3. The plot of initial rate (ki) against the concentration of ascorbic acid also yields a straight line passing through the origin indicating first order dependence with respect to organic acid. Second order plots of log of [PMS]t/[H2A]t or [H2A]t/[PMS]t were also made for comparable concentrations of the reactants. The second order rate constants calculated from these plots are in agreement with those calculated from initial rates (Table 1).
Initial rates (ki) and second order rate constants (k2) in l-ascorbic acid and peroxomonosulphate reaction. [HClO4] = 0.02 mol dm−3, 25 °C.
103 [PMS] | 103 [H2A] | 106 (ki) | 102 (k) |
mol dm−3 | mol dm−3 | mol dm−3s−1 | dm3 mol s−1 |
2.0 | 2.0 | 0.54 | 13.5 (13.3)a |
3.0 | 2.0 | 0.78 | 13.0 (13.2) |
4.0 | 2.0 | 1.10 | 13.7 (13.1) |
5.0 | 2.0 | 1.33 | 13.3 (13.0) |
6.0 | 2.0 | 1.73 | 14.4 (13.8) |
8.0 | 2.0 | 2.32 | 14.5 (14.4) |
10.0 | 2.0 | 2.90 | 14.5 (13.8) |
5.0 | 3.0 | 2.13 | 14.2 (14.4) |
5.0 | 4.0 | 2.66 | 13.3 (13.4) |
5.0 | 5.0 | 3.33 | 13.3 (13.5)a |
5.0 | 6.0 | 4.0 | 13.3 (13.4) |
5.0 | 8.0 | 5.83 | 14.5 (14.0) |
5.0 | 10.0 | 7.22 | 14.4 (14.5) |
2.0 | 2.0 | – | 13.3a |
3.0 | 3.0 | – | 13.3a |
4.0 | 4.0 | – | 13.3a |
5.0 | 5.0 | – | 13.3a |
6.0 | 6.0 | – | 13.8a |
8.0 | 8.0 | – | 13.3a |
10.0 | 10.0 | – | 13.5a |
a The rate constants marked () were calculated from stoichiometric plots.
Also, the second order nature of the reaction was further confirmed by undertaking kinetics of the reaction for stoichiometric concentrations of the reactants. A plot of [H2A]t−1 versus time (Fig. 1) was made that yielded a straight line, the second order rate constant calculated from the slope of such lines is in agreement to the rate constants calculated under varied experimental conditions as collected in Table 1.

Stoichiometric plot in the reaction of ascorbic acid and PMS. [H+] = 2.0 × 10-2 mol dm-3; [PMS] = [H2A] = 2 × 10-3, 3 × 10-3, 4 × 10-3, 5 × 10-3, 6 × 10-3, 8 × 10-3 and 1 × 10-2 mol dm-3, 25 °C.
3.1.3 Hydrogen ion effect
Hydrogen ion concentration was varied by employing perchloric acid from 0.02 to 0.2 mol dm−3 at I = 1.0 mol dm−3 (adjusted by employing lithium perchlorate) at fixed concentrations of other reaction components viz. [PMS] = 5.0 × 10−3 mol dm−3, [H2A] = 2.0 × 10−3 mol dm−3 and at 23, 25, 28 and 32 °C respectively. The rate decreases with increasing concentration of perchloric acid.
3.1.4 Effect of ionic strength
The effect of ionic strength was studied by employing lithium perchlorate from 0.02 to 0.2 mol dm−3 at fixed concentrations of other reaction ingredients viz. [PMS] = 5.0 × 10−3 mol dm−3, [H2A] = 2.0 × 10−3 mol dm−3 and [H+] = 0.02 mol dm−3. The rate increases with increasing ionic strength.
3.1.5 Activation parameters
The kinetics of the reaction keeping [PMS] = 5.0 × 10−3 mol dm−3, [H2A] = 2.0 × 10−3 mol dm−3 and [H+] = 0.02 mol dm−3 constant at 23, 25, 28 and 32 °C respectively and I = 1.0 mol dm−3 was also studied. A plot of log (k2, dm3 mol−1 s−1) versus 1/T yields a straight line. The energy of activation to be (57 ± 3.0) kJ mol−1 and entropy of activation to be (–71 ± 5) JK−1 mol−1 were calculated in a conventional manner.
3.1.6 Reaction catalyzed by copper (II)
The concentration of copper (II) was varied in the range (1.0 to 10.0) × 10−5 mol dm−3 at fixed concentration of other reaction components viz. [PMS] = 5.0 × 10−3 mol dm−3, [H2A] = 2.0 × 10−3 mol dm−3 and [H+] = 0.2 mol dm−3. The rate increases with increasing concentration of copper (II) (Fig. 2). Such a plot, however, shows occurrence of simultaneously uncatalyzed reaction to a significant extent.
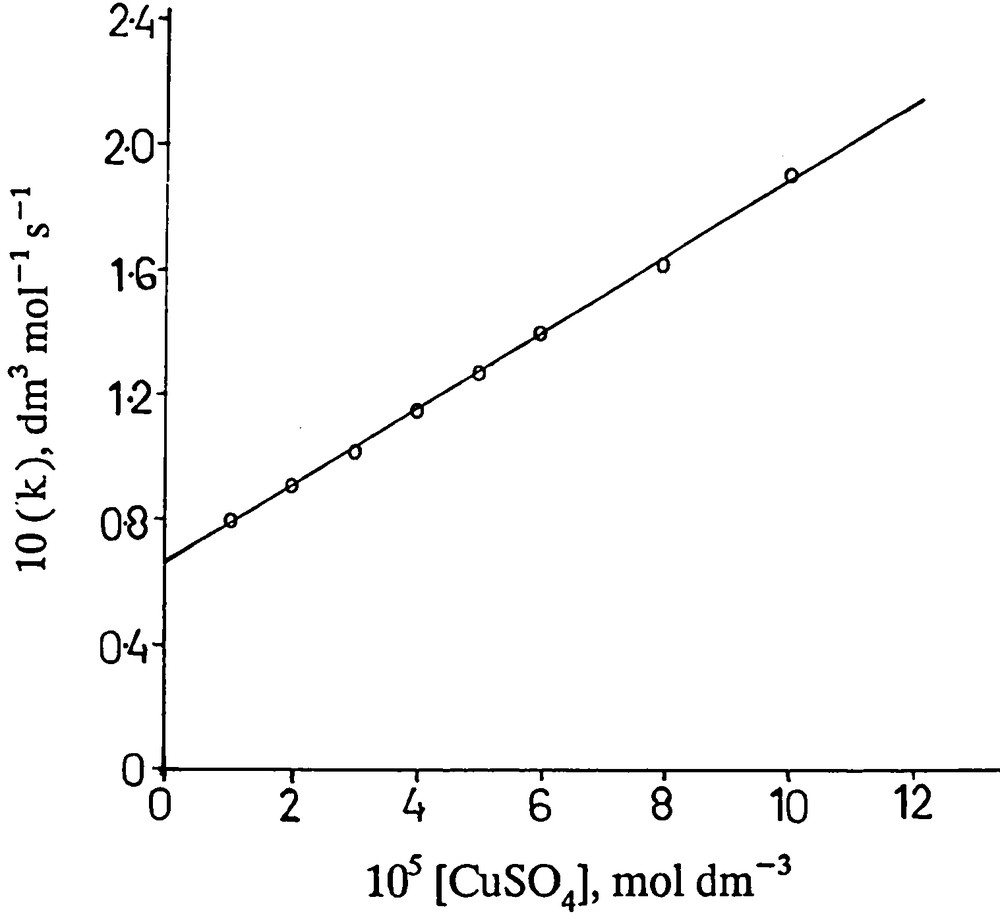
Variation of copper sulphate. [PMS] = 5.0 × 10-3 mol -3; [H2A] = 2.0 × 10-3 mol dm-3; I = 1.0 mol -3; 25 °C.
Hydrogen ion concentration was varied by employing perchloric acid from 0.08 to 0.3 mol dm−3 at [CuII] = 5.0 × 10−5 mol dm−3, [PMS] = 5.0 × 10−3 mol dm−3 and [H2A] = 2.0 × 10−3 mol dm−3. The rate decreases with increasing hydrogen ion concentration as in case of uncatalyzed reaction.
The orders with respect to [PMS] and [H2A] respectively were found to be one in catalyzed reaction (Table 2) as in case of uncatalyzed reaction (Table 1).
Second order rate constants in CuII-catalyzed oxidation of l-ascorbic acid by peroxomonosulphate.
103 [PMS] | 103 [H2A] | 102 (k2) |
mol dm−3 | mol dm−3 | dm3 mol−1s−1 |
5.0 | 2.0 | 12.7 |
5.0 | 3.0 | 12.7 |
5.0 | 4.0 | 12.8 |
5.0 | 6.0 | 12.5 |
5.0 | 8.0 | 12.7 |
5.0 | 10.0 | 12.0 |
3.0 | 2.0 | 12.4 |
4.0 | 2.0 | 12.5 |
6.0 | 2.0 | 12.0 |
8.0 | 2.0 | 12.2 |
10.0 | 2.0 | 12.5 |
4 Discussion
Since oxidations of ascorbic acid [H2A] are pH dependent, probably it is this reason that the reducing properties of ascorbic acid significantly differ from those of the conjugate base species such as HA−and A2−. The pKa's of ascorbic acid [51] at 25 °C and I = 1.0 mol dm−3 are ∼ 3.99 and ∼ 11.28 respectively. This shows that the major species of the acid in strongly acid medium (0 < pH < 1) is its molecular form (H2A), monoanionic species HA− is significant only in the range 2.5 < pH < 5.5 and dianion A2− is reactive only at pH > 6.0. Kimura et al. [30] considered H2A and HA− species for the studies in the pH region 3.2–4.7. If the hydrogen ion concentration employed in the uncatalyzed study is any guide, the major reactive species of ascorbic acid are H2A and HA− whereas the concentration of A2− species is insignificant. In view of the hydrogen ion effect on the rate, HA−, appears to be the predominantly reactive species of ascorbic acid.
Thus if all these kinetic observations are taken into account, the following mechanism consisting of steps (2) to (5) can be envisaged.
(2) |
(3) |
(4) |
(5) |
Since intermediate complex is indicated neither kinetically nor spectrally, the reaction should, therefore, occur in an outer-sphere mode as in case of other reactions.
Thus the loss of ascorbic acid, leads to the rate law (6):
(6) |
where [PMS]T and [H2A]T are the gross analytical concentrations of peroxomonosulphate and ascorbic acid respectively. The rate law (6) is further reduced to Eq. (7)
(7) |
where k is an observed second order rate constant (Table 1). A plot of k versus [H+]−1 was made from Eq. (7) that yielded a straight line passing through the origin with zero intercept (Fig. 3). k2K1 was calculated from the gradients to be 5.7, 8.0, 11.0 and 13.5 × 10−3 s−1 at 23, 25, 28 and 32 °C respectively at I = 1.0 mol dm−3. Thus HA−species is predominantly reactive form of ascorbic acid and the contribution to the overall rate of (H2A + PMS) reaction compared to (HA− + PMS) reaction is negligible. The rate enhancement in due to ionic strength also justifies interaction of like charged species of the reactants in the rate-controlling step of the reaction mechanism.
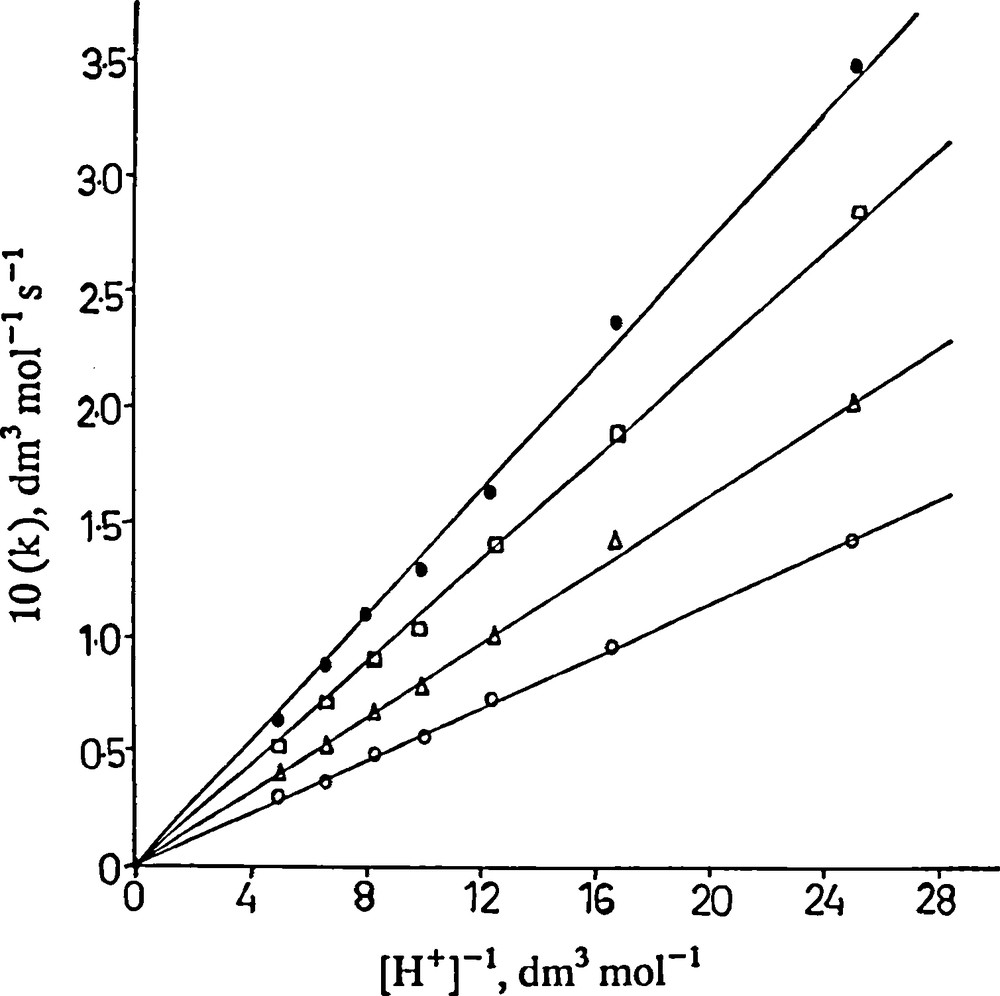
A plot of (k) versus [H+]-1. [PMS] = 5.0 × 10-3 mol-3; [H2A] = 2.0 × 10-3 mol dm-3; I = 1.0 mol-3. : 23; : 25; : 28 and : 32 °C.
The reactivity of the monoanion species of ascorbic acid is further strengthened if the effect of ionic-strength (I < 0.2 mol dm−3) is taken into account in the light of Bronsted-Bjerrum-Christiansen [52] Eq. (8).
(8) |
where βa is a constant which is related to the size of the ion. A is another constant which is generally given by the relationship (9)
(9) |
where D is the dielectric constant of the medium, T is temperature in Kelvin and k0 is the rate constant at infinite dilution. Z+ and Z−are the charges on the two reactant species. This Eq. (8) is further reduced to Eq. (10) at 25 °C at which A = 0.509 and βa = 1,
(10) |
A plot of log k versus was made from the Eq. (10) that yielded a straight line with a slope of ∼ 0.85, a value which is close to unity. This confirms HSO5−and HA− to be the reactive species. Alternatively, the rate dependence on inverse hydrogen ion concentration can also be explained if all the three species of ascorbic acid viz. H2A, HA− and A2− are considered with the assumption that A2− being in small concentration is highly reactive. Considering steps (2) and (3) along with step (11) to be the rate controlling, the rate law (13) is obtained from such a mechanism.
(11) |
Such a mechanism leads to the rate law (12) or (13)
(12) |
or
(13) |
This rate law (13) is further reduced to Eq. (14) assuming the valid assumption:
K1 [H+] >> [H+]2 + K1K2
(14) |
Eq. (14) is similar to Eq. (7) with only difference that K2 replaces K1 in the numerator.
Such a proposal is, however, contrary to the following facts.
- • If pK1 and pK2 are any guide the species A2− is reported in solutions of pH > 6. Hydrogen ion concentration being significantly high rules out the reactivity A2− species.
- • An observed value of Z+ Z−to be ∼ 0.85 does not match the theoretical slope to be ∼ 2.0 expected for the species A2−.
4.1 Cu(II) catalyzed oxidation of ascorbic acid
In CuII-catalyzed oxidation of ascorbic acid by various oxidants such as peroxydisulphate [53], hydrogen peroxide [54] and oxygen [43], the kinetic orders are one and half respectively. Moreover, rate is independent of peroxodiphosphate concentration in oxidation of ascorbic acid by peroxodiphosphate. Peroxomonophosphate -H2A reaction in the presence of CuII as a catalyst conforms to second order kinetics viz first order with respect to the oxidant and substrate, respectively. Also, order with respect to the catalyst is one.
Copper (II) is known to form complex(s) with ascorbic acid and the stability constant to be 42 ± 6 dm3 mol−1 is reported both kinetically [55] and spectrally. If experimental observations in this study of Cu(II) catalyzed oxidation of ascorbic acid by peroxomonosulphate are taken into account, the following mechanism can be envisaged.
(15) |
(16) |
(17) |
(18) |
It is also recognized [56] that peroxo co-ordinated adducts are readily formed and the reduction of such an adduct proceeds through a transient species with properties similar to that of a peroxo-bridged precursor complex.
Such a mechanism leads to the rate law (19)
(19) |
where [PMS], [H2A] and [CuII] are the gross analytical concentrations of peroxomonosulphate, ascorbic acid and copper(II) respectively.
This rate law (19) is further reduced to the form (20)
(20) |
where k” is an observed second order rate constant. If uncatalyzed reaction path which occurs significantly is also taken into account, the rate law accounting for this path can be given by the Eq. (21) or (22)
(21) |
(22) |
A plot of (k”–kun’) versus [H+]−1 was made from Eq. (22) that yielded a straight line with zero intercept (Fig. 4). The slope yielded the values of k2”K2′K1 to be 1.2, 1.7, 2.2 and 2.7 × 10−2 dm3 mol−1 s−1 at 23, 25, 28 and 32 °C, respectively at I = 1.0 mol dm−3.
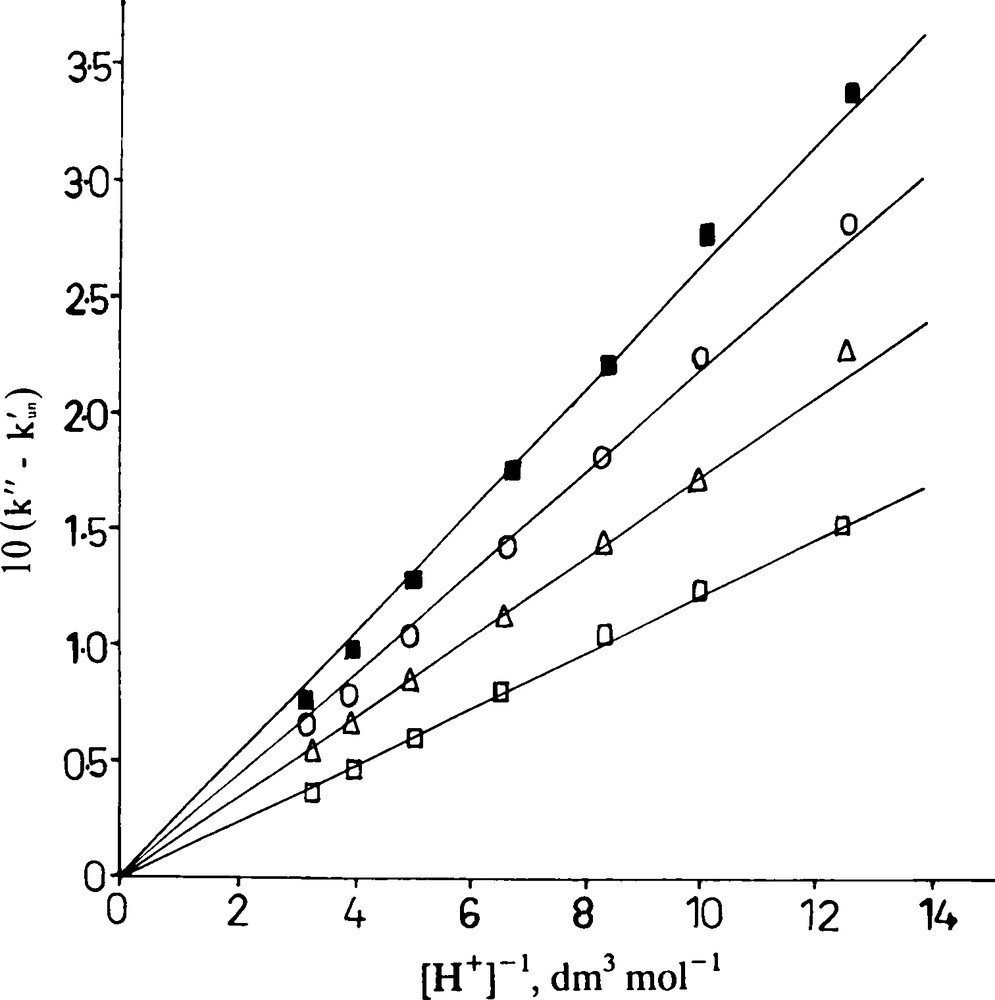
A plot of (k” – kun’) versus [H+]-1. [PMS] = 5.0 × 10-3 mol dm-3; [H2A] = 2.0 × 10-3 mol dm-3; [Cu(II)] = 5.0 × 10-5 mol dm-3; I = 1.0 mol dm-3; Temp. = : 23; : 25; : 28 and : 32 °C.
The values of k2” are significantly higher than those of k1” which is invariably true in oxidation reactions of ascorbic acid and not only in CuII-catalyzed oxidation of this acid.
It is also reported [55] that uncatalyzed path in copper(II)-catalyzed oxidation of ascorbic acid by either dissolved oxygen or air is due to the presence of CuII or FeII as impurities in traces in the reagents. The real uncatalyzed path is not significant.
Hydrogen ion dependence can also be explained alternatively provided all the species such as H2A, HA−and A2− of ascorbic acid are equally reactive with two species of copper(II). If hydrolysis of CuII as in Eq. (23) is taken into account (∼ 10−7), the following mechanism giving the rate law (28) can be proposed:
(23) |
(24) |
(25) |
(26) |
(27) |
(28) |
Ascorbic acid, no doubt, forms reactive free-radical intermediates as the monomer acrylic acid in the reaction mixture polymerizes just on addition into reaction mixture. However, t-butyl alcohol does not affect the rate of the reaction eliminating the possibility of formation of reactive sulphate radicals (SO4−).
In conclusion, it is established that peroxomonosulphate with ascorbic acid does not yield sulphate free radicals. Also, the stoichiometric kinetics further establishes two-equivalent nature of ascorbic acid. Hydrogen ion dependence is of usual nature as has been observed in other reactions. The reaction is catalyzed by traces of CuII ions but not tremendously.