1 Introduction
Organic reactions in aqueous media have attracted much attention recently not only because water is the most abundant, environmentally benign, and cheapest solvent but also because unique selectivity and reactivity can be expected from this solvent [1].
Multicomponent reactions (MCR) are very efficient synthetic methods. The strategy offers significant advantages over classical stepwise approaches allowing the formation of several bonds and the construction of complex molecular architectures from simple precursors in a single synthetic operation without the need for isolation of intermediates [2]. MCRs, particularly those performed in aqueous media, have become increasingly useful tools for the synthesis of chemically and biologically important compounds because of their environmentally friendly atom economy and green characteristics [3]. Furthermore, the discovery of novel MCRs can be considered an interesting topic for academic research that also satisfies the practical interest of applied science [2,3].
The organic nitriles are of much importance in organic synthesis as these are versatile intermediates in the synthesis of amines, carboxylic acids, esters and ketones which are useful raw materials for pharmaceuticals, agrochemicals and dyes [4]. The cyano moiety is also present in numerous bioactive molecules [5]. The classical method for the synthesis of alkyl cyanide involves nucleophilic substitution of an alkyl halide with metal cyanides [4a].
Furthermore, the introduction of nitrile into organic compounds can be achieved using different routes including: the direct conversion of aldehydes [6], oxime ethers [7], thioamides [8], carboxylic acid and their esters [9], dehydration of aldoximes [10] and amides [11] with various reagents, oxidation of amines [12], and reduction of primary aliphatic nitro compounds [13], enzymatic methods [14] and application of organoborane/diazoacetonitrile [15]. Unfortunately, most of the reported methods suffer from serious drawbacks such as the difficulty of available reagents, low yields, drastic reaction conditions and tedious work-up procedures. Thus, the development of a convenient and efficient methodology for the synthesis of β-cyanocarbonyl compounds is an interesting target for investigation.
One of the best methods for the introduction of a cyanide group involves the Michael addition of cyanide anion to an α,β-unsaturated carbonyl derivatives in the presence of strong bases or Lewis acid catalysts [16]. As part of our ongoing research aimed at developing synthesis of alkyl nitriles [17], herein we report a one-pot, three-component reaction of Meldrum's acid 1, an aldehyde 2 and sodium cyanide 3, for the preparation of alkyl nitrile or β-cyanocarbonyl 4 without using any catalyst at room temperature in water (Scheme 1).

Synthesis of β-cyanocarbonyls.
2 Results and discussion
We compared the reaction rate in different solvents by measuring the isolated yield using identical amounts of reactants for a fixed reaction time of 6 h at room temperature. The desired product was obtained in protic solvents such as water, ethanol, and methanol but water can afford the product in good yield even better than ethanol and methanol. The desired product was scarcely obtained in dichloromethane, benzene, and toluene. We also studied the reaction at different temperatures. The reaction rate decreased as the reaction temperature was raised. Because at high temperature reaction aldol-Michael two equivalents of Meldrum's acid with aldehyde is a competing reaction; the product of this reaction was separated as a by-product in high temperature [18].
With the optimal conditions in hand, the range of the substrates was checked. The Knoevenagel condensation and Michael addition reaction were carried out using a series of different aldehydes with Meldrum's acid and sodium cyanide. The results are summarized in Table 1. The structures of the products were established by different spectroscopic methods (for details see Experimental section). In all cases, excellent yields were obtained, whatever the nature of the substituent present on the aldehyde (electron-donating or electron-withdrawing). This confirms the reliability of the synthetic method.
Reaction of Meldrum's acid and aldehyde with sodium cyanide in water at room temperature.
Entry | R1 | Product | Yield (%)a |
1 | H | 4a | 99 |
2 | 4-Cl | 4b | 98 |
3 | 4-OH | 4c | 98 |
4 | 4-NO2 | 4d | 99 |
5 | 4-OMe | 4e | 99 |
6 | 4-Me | 4f | 99 |
7 | 3-OH | 4g | 98 |
8 | 3-NO2 | 4h | 98 |
a Isolated yield.
For the scope and limitation of the method, we investigated the reaction of 2-naphthyl carbaldehyde with Meldrum's acid and sodium cyanide under the above conditions led to the formation of the corresponding alkyl nitriles in 99% yields (Scheme 2).
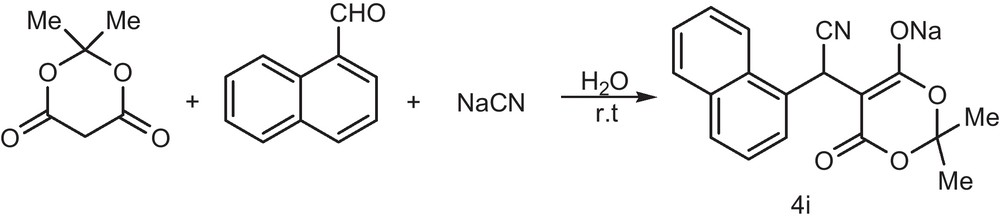
Synthesis of alkyl nitrile derivative.
Mechanistically, it is conceivable that the reaction involves the initial formation of activated alkene or α,β-unsaturated carbonyl 5 through a Knoevenagel condensation reaction of Meldrum's acid and aldehyde. Afterwards the intermediate 5 undergoes Michael addition reaction with cyanide to afford β-cyanocarbonyls 4 (mechanism A). Another mechanism possible for this reaction involves the initial formation of cyanohydrin 6 through a cyanohydrin formation of sodium cyanide with aldehyde. Afterwards nucleophilic substitution of the cyanohdrin 6 with sodium cyanide affords to β-cyanocarbonyls 4 (mechanism B) (Scheme 3). To clarify the proposed mechanisms, first, the Michael acceptor 5 was synthesized from condensation of Meldrum's acid and benzaldehyde, subsequently reaction of 5 with sodium cyanide afforded corresponding β-cyanocarbonyl 4. Also for further clarification, cyanohdrin 6 was synthesized from reaction aldehyde with sodium cyanide in water; subsequently cyanohdrin 6 did not undergo reaction with Meldrum's acid under the reaction conditions. Therefore mechanism A is reasonable.

Proposal mechanisms.
3 Conclusion
In conclusion, the efficient synthesis of alkyl nitrile in water, without employing catalyst has been described. The three-component reaction involving Meldrum's acid, an aromatic aldehyde and sodium cyanide afforded the desired products with excellent yields. A wide range of aromatic aldehydes were tested, bearing either electron-donating or electron-withdrawing substituent's, suggesting that this method can be used to synthesize a large variety of compounds. It has therefore been demonstrated that using water as the solvent is ideally suited for the synthesis of alkyl nitriles.
4 Experimental
4.1 Materials and techniques
Melting points were taken on an Electrothermal 9100 apparatus and left uncorrected. IR spectra were obtained on a Shimadzu IR-470 spectrometer. 1H and 13C NMR spectra were recorded on a Bruker DRX-200 Avance spectrometer at 200 and 50 MHz. NMR spectra were obtained on solutions in DMSO using tetramethylsilane as internal standard. All of the chemicals were purchased from Fluka, Merck and Aldrich and used without purification.
4.2 Typical procedure: preparation of 2-(sodium 2,2-dimethyl-4-oxo-4H-1,3-dioxin-5-yl-6-oxide)-2-phenylacetonitrile (4a)
A solution of Meldrum's acid (0.14 g, 1 mmol), benzaldehyde (0.11 g, 1 mmol) and sodium cyanide (0.05 g, 1 mmol) in H2O (10 mL) was stirred for 6 h at room temperature. After completion of the reaction as indicated by TLC, the solvent was evaporated, and the residue was washed with ether and recrystallized in ether/n-hexane (50/50) to give the pure product 4a. White powder (0.28 g, yield 99%). Mp 218 °C. IR (KBr) (νmax /cm−1): 3183, 2272, 1720. MS, (m/z): 232 (M+-NaCN). 1H NMR (200 MHz, DMSO-d6): δH (ppm) 1.44 (6H, s, CH3-C-CH3), 5.09 (1H, s, CH-CN), 7.16–7.35 (5H, m, H-Ar). 13C NMR (50 MHz, DMSO-d6): δC (ppm) 26.2 (CH3-C-CH3), 32.4 (CH-CN), 71.7 (C=CC-ONa), 100.4 (CH3-C-CH3), 121.7, 126.8, 127.2, 128.4, 139.2 (C-Ar and CN), 164.6 (C=CO). Anal. Calcd for C14H12NNaO4: C, 59.79; H, 4.30; N, 4.98. Found: C, 59.83; H, 4.27; N, 5.11.
4.3 2-(4-chlorophenyl)-2-(sodium-2,2-dimethyl-4-oxo-4H-1,3-dioxin-5-yl-6-oxide) acetonitrile (4b)
Yellow powder (0.29 g, yield 98%). Mp 197 °C. IR (KBr) (νmax /cm−1): 3182, 2271, 1725. MS, (m/z): 266 (M+-NaCN). 1H NMR (200 MHz, DMSO-d6): δH (ppm) 1.42 (6H, s, CH3-C-CH3), 5.07 (1H, s, CH-CN), 7.30–7.32 (4H, m, H-Ar). 13C NMR (50 MHz, DMSO-d6): δC (ppm) 26.1 (CH3-C-CH3), 31.9 (CH-CN), 71.8 (C=C-ONa), 100.7 (CH3-C-CH3), 121.3, 128.4, 129.1, 131.5, 138.2 (C-Ar and CN), 164.7 (C=O). Anal. Calcd for C14H12ClNO4: C, 57.25; H, 4.12; Cl, 12.07; N. Found: C, 57.33; H, 4.17; N, 12.11.
4.4 2-(sodium-2,2-dimethyl-4-oxo-4H-1,3-dioxin-5-yl-6-oxide)-2-(4-hydroxyphenyl) acetonitrile (4c)
Red powder (0.29 g, yield 98%). Mp 193 °C. IR (KBr) (νmax /cm−1): 3184, 2273, 1727. MS, (m/z): 248 (M+-NaCN). 1H NMR (200 MHz, DMSO-d6): δH (ppm) 1.49 (6H, s, CH3-C-CH3), 3.47 (OH exchanged with water of DMSO-d6), 5.02 (1H, s, CH-CN), 6.70 (2H, d, 3JHH = 8.4 Hz, H-Ar), 7.20 (2H, d, 3JHH = 8.4 Hz, H-Ar). 13C NMR (50 MHz, DMSO-d6): δC (ppm) 26.3 (CH3-C-CH3), 31.6 (CH-CN), 71.7 (C=C-ONa), 100.1 (CH3-C-CH3), 115.1, 122.1, 128.3, 129.7, 156.2 (C-Ar and CN), 164.4 (C=O). Anal. Calcd for C14H12NNaO5: C, 56.57; H, 4.07; N, 4.71. Found: C, 56.62; H, 4.17; N, 4.79.
4.5 2-(sodium-2,2-dimethyl-4-oxo-4H-1,3-dioxin-5-yl-6-oxide)-2-(4-nitrophenyl) acetonitrile (4d)
Brown powder (0.32 g, yield 99%). Mp 178 °C. IR (KBr) (νmax /cm−1): 3187, 2270, 1726. MS, (m/z): 248 (M+-NaCN). 1H NMR (200 MHz, DMSO-d6): δH (ppm) 1.48 (6H, s, CH3-C-CH3), 5.29 (1H, s, CH-CN), 7.63 (2H, d, 3JHH = 8.5 Hz, H-Ar), 8.22 (2H, d, 3JHH = 8.5 Hz, H-Ar). 13C NMR (50 MHz, DMSO-d6): δC (ppm) 26.2 (CH3-C-CH3), 31.8 (CH-CN), 71.10 (C=C-ONa), 100.1 (CH3-C-CH3), 123.0, 123.7, 128.4, 130.4, 147.5 (C-Ar and CN), 164.4 (C=O). Anal. Calcd for C14H11N2NaO6: C, 51.54; H, 3.40; N, 8.59. Found: C, 51.60; H, 3.38; N, 8.60.
4.6 2-(sodium-2,2-dimethyl-4-oxo-4H-1,3-dioxin-5-yl-6-oxide)-2-(4-methoxyphenyl) acetonitrile (4e)
Cream powder (0.31 g, yield 99%). Mp 195 °C. IR (KBr) (νmax /cm−1): 3183, 2277, 1730. MS, (m/z): 262 (M+-NaCN). 1H NMR (200 MHz, DMSO-d6): δH (ppm) 1.40 (6H, s, CH3-C-CH3), 3.67 (3H, s, OCH3), 5.10 (1H, s, CH-CN), 7.80 (2H, d, 3JHH = 8.0 Hz, H-Ar), 7.22 (2H, d, 3JHH = 8.0 Hz, H-Ar). 13C NMR (50 MHz, DMSO-d6): δC (ppm) 26.3 (CH3-C-CH3), 31.6 (CH-CN), 55.5 (OCH3), 71.7 (C=C-ONa), 100.2 (CH3-C-CH3), 113.8, 122.0, 128.4, 131.4, 158.2 (C-Ar and CN), 164.4 (C=CO). Anal. Calcd for C15H14NNaO5: C, 57.88; H, 4.53; N, 4.50. Found: C, 57.78; H, 4.59; N, 4.42.
4.7 2-(sodium-2,2-dimethyl-4-oxo-4H-1,3-dioxin-5-yl-6-oxide)-2-p-tolylacetonitrile (4f)
White powder (0.29 g, yield 99%). Mp 160 °C. IR (KBr) (νmax /cm−1): 3182, 2271, 1731. MS, (m/z): 268 (M+-NaCN). 1H NMR (200 MHz, DMSO-d6): δH (ppm) 1.65 (6H, s, CH3-C-CH3), 2.30 (3H, s, CH3), 5.15 (1H, s, CH-CN), 7.19 (2H, d, 3JHH = 8.1 Hz, H-Ar), 7.33 (2H, d, 3JHH = 8.1 Hz, H-Ar). 13C NMR (50 MHz, DMSO-d6): δC (ppm) 21.0 (CH3), 26.3 (CH3-C-CH3), 32.1 (CH-CN), 71.6 (C=CC-ONa), 100.2 (CH3-C-CH3), 121.8, 127.2, 128.9, 135.7, 136.5 (C-Ar and CN), 164.4 (C=CO). Anal. Calcd for C15H14NNaO4: C, 61.02; H, 4.78; N, 4.74. Found: C, 61.12; H, 4.83; N, 4.64.
4.8 2-(sodium-2,2-dimethyl-4-oxo-4H-1,3-dioxin-5-yl-6-oxide)-2-(3-hydroxyphenyl) acetonitrile (4g)
Cream powder (0.29 g, yield 98%). Mp 192 °C. IR (KBr) (νmax /cm−1): 3183, 2271, 1726. MS, (m/z): 248 (M+-NaCN). 1H NMR (200 MHz, DMSO-d6): δH (ppm) 1.36 (6H, s, CH3-C-CH3), 3.67 (OH exchanged with water of DMSO-d6), 4.91 (1H, s, CH-CN), 6.48–7.01 (4H, m, H-Ar). 13C NMR (50 MHz, DMSO-d6): δC (ppm) 26.4 (CH3-C-CH3), 32.3 (CH-CN), 71.3 (C=CC-ONa), 100.1 (CH3-C-CH3), 113.7, 114.3, 117.9, 121.7, 129.2, 140.8, 157.5 (C-Ar and CN), 164.4 (C=CO). Anal. Calcd for C14H12NNaO5: C, 56.57; H, 4.07; N, 4.71. Found: C, 56.64; H, 4.27; N, 4.73.
4.9 2-(sodium-2,2-dimethyl-4-oxo-4H-1,3-dioxin-5-yl-6-oxide)-2-(3-nitrophenyl) acetonitrile (4h)
Cream powder (0.32 g, yield 98%). Mp 185 °C. IR (KBr) (νmax /cm−1): 3183, 2271, 1729. MS, (m/z): 248 (M+-NaCN). 1H NMR (200 MHz, DMSO-d6): δH (ppm) 1.43 (6H, s, CH3-C-CH3), 5.23 (1H, s, CH-CN), 7.50–8.13 (4H, m, H-Ar). 13C NMR (50 MHz, DMSO-d6): δC (ppm) 26.1 (CH3-C-CH3), 32.4 (CH-CN), 71.50 (C=CC-ONa), 100.8 (CH3-C-CH3), 120.8, 121.7, 122.1, 130.1, 134.0, 141.5, 147.5 (C-Ar and CN), 164.7 (C=CO). Anal. Calcd for C14H11N2NaO6: C, 51.54; H, 3.40; N, 8.59. Found: C, 51.44; H, 3.38; N, 8.51.
4.10 2-(sodium-2,2-dimethyl-4-oxo-4H-1,3-dioxin-5-yl-6-oxide)-2-(naphthalen-3-yl) acetonitrile (4i)
Cream powder (0.33 g, yield 98%). Mp 188 °C. IR (KBr) (νmax /cm−1): 3184, 2270, 1726. MS, (m/z): 304 (M+-NaCN). 1H NMR (200 MHz, DMSO-d6): δH (ppm) 1.50 (6H, s, CH3-C-CH3), 5.32 (1H, s, CH-CN), 7.50–7.91 (7H, m, H-Ar). 13C NMR (50 MHz, DMSO-d6): δC (ppm) 26.2 (CH3-C-CH3), 32.6 (CH-CN), 71.80 (C=CC-ONa), 100.5 (CH3-C-CH3), 120.6, 125.1, 126.0, 126.1, 126.6, 127.8, 128.0, 128.1, 132.2, 133.0, 136.9 (C-Ar and CN), 164.6 (C=CO). Anal. Calcd for C18H14NNaO4: C, 65.26; H, 4.26; N, 4.23. Found: C, 65.34; H, 4.22; N, 4.32.
Acknowledgements
We gratefully acknowledge financial support from the Research Council of Razi University.