1 Introduction
Squaraines are a class of strongly colored dyes bearing a central electron deficient four-membered ring core surrounded by electron rich aromatic moieties. These dyes are interesting compounds in chemical synthesis mainly because their species show strong absorption bands (ɛ ∼ 105 L.mol−1.cm−1) in the visible region [1], intense fluorescence emission [2] and good photoconductivity [3]. These features make them useful in a variety of applications in the area of imaging [4,5], nonlinear optics [6–8], photodynamic therapy [9–12], photovoltaic devices [13–15], ion sensing [16], as well as in organic synthesis [17]. Furthermore, squaraine dyes are suitable for fluorescence detection of proteins at long-wavelength excitation and labels in biological assay [18] due their unique physicochemical and photophysical properties namely light absorption in the visible red and near-infrared regions [19].
Squaraine dyes are generally prepared by the condensation of electron rich aromatic, heteroaromatic or olefinic compounds with squaric acid or its esters in a one-step reaction [20]. In a typical procedure, symmetrical squaraines 3 are synthesized by the reaction of dialkyl esters derived from squaric acid, e.g. 3,4-dibutoxy-3-cyclobutene-1,2-dione 1 with two equivalents of a quaternized methylene base, e.g. 1,3,3-trimethyl-2-methyleneindoline 2 (Scheme 1) [21].
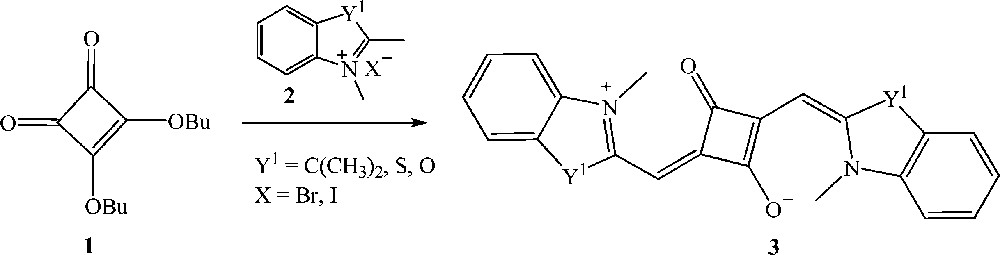
Unsymmetrical squaraines are synthesized via a multi-step procedure (Scheme 2) [22]. In the first step, the reaction of squaric ester 1 with an equimolar amount of heterocyclic quaternary ammonium salt 2, in the presence of triethylamine as catalyst and n-butanol as solvent, leads to the intermediate mono-squaraine 4. Subsequent reaction of 4 with a second equimolar amount of a methylene base affords the corresponding unsymmetrical squaraine 6.

Although similar structures have been presented in the literature, this work presents the synthesis of new dicyanomethylene-substituted squaraine dyes with good yields and fluorescence emission in infrared region. Additionally, we believe that this manuscript can contribute not only in the field of fluorescence sensors, but as well as new dyes with potential application as dye sensitized solar cells [23,24].
2 Results and discussion
2.1 Synthesis of squaraine dyes
Symmetrical dicyanomethylene-substituted squaraine dye 11 was synthesized in four steps from squaric acid as shown in Scheme 3. The synthetic route includes initially the reaction of squaric acid (7) and excess of n-butanol to afford the dibutyl ester 1 [25], which was subsequently treated with 1.0 equivalent of malononitrile in the presence of triethylamine to give triethylammonium-2-butoxy-3-dicyanomethylene-4-oxocyclobut-1-en-1-olate (8) in 85% yield [26]. Next, commercially available 2,3,3-trimethylindol (9) was converted in the heterocyclic quaternary ammonium salt 10 by coupling of 9 with excess of iodooctane (5.0 equiv.) in 75% yield after chromatography [27]. The subsequent base-catalyzed condensation of 2.0 equivalents of 3H-indolium salt 10 and 1.0 equivalent of 8 under reflux in an n-butanol:pyridine mixture [28], afforded the symmetrical dye 11 in 39% yield.

The synthesis of the unsymmetrical squaraine dye 16 was performed as depicted in Scheme 4. In the first step, 2-aminothiophenol (12) was treated with 1.0 equivalent of acetyl chloride at room temperature using toluene as solvent to afford 2-methylbenzothiazole (13) in 85% yield after purification by chromatography [29]. Subsequent quaternization of 13 using excess of iodooctane led to the N-octyl-2-methylbenzothiazolium iodide (14) in satisfactory yield (68% after purification). Condensation of 1.0 equivalent of 10 with 1.0 equivalent of squarate 8, in the presence of triethylamine and ethanol as solvent, afforded the intermediate mono-squaraine 15 [30]. Reflux of crude product containing mainly 15 with benzothiazolium salt 10 in an n-butanol:benzene mixture led to the unsymmetrical dye 16 in 25% yield from quaternized indolenine 10.
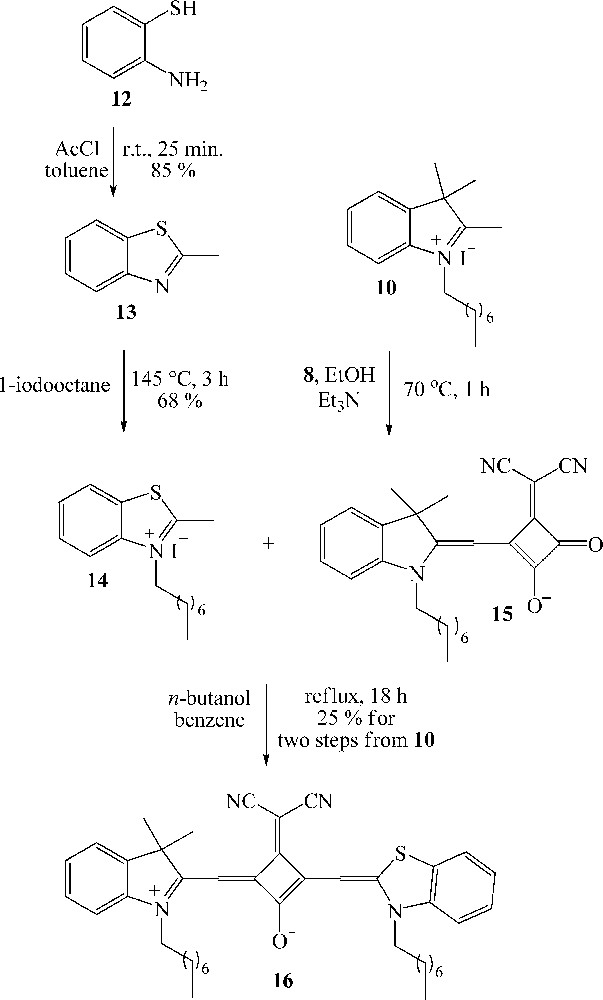
The squaraine dye 17 was obtained in 42% yield in a unique step by condensation of 1.0 equivalent of the 3-dicyanomethylene squarate 8 with 2.0 equivalents of benzothiazolium 14 under reflux in an n-butanol:pyridine mixture (Scheme 5).
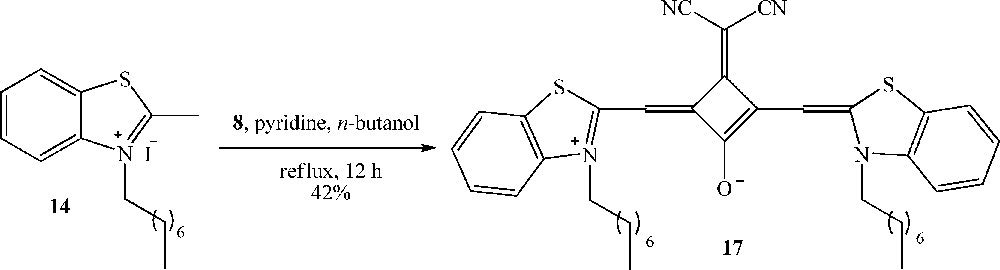
2.2 Photophysical characterization of free dyes in organic solvents
In Figs. 1–3 are presented the normalized UV–Vis absorption and fluorescence emission spectra of the squaraine dyes 11, 16 and 17 in 1,4-dioxane, chloroform, ethanol, dimethylformamide and dimethylsulfoxide. The spectral shapes of the dyes are almost the same as expected [31], and the relevant data are summarized in Table 1.

Normalized absorption and fluorescence emission spectra of the squaraine dye 11.

Normalized absorption and fluorescence emission spectra of the squaraine dye 16.

Normalized absorption and fluorescence emission spectra of the squaraine dye 17.
Relevant photophysical data from UV–Vis absorption and fluorescence emission of the squaraine dyes 11, 16 and 17.
Dye | Solvent | λabs (nm) | ɛ × 105 (M−1·cm−1) | λem (nm) | λex (nm) | Stokes’ shift (nm/cm−1) | Φfl |
11 | 1,4-Dioxane | 689 | 1.90 | 702 | 686 | 13/321 | 0.19 |
CHCl3 | 685 | 0.99 | 695 | 686 | 10/247 | 0.18 | |
EtOH | 673 | 0.73 | 687 | 671 | 14/345 | 0.13 | |
DMF | 681 | 1.94 | 691 | 681 | 10/247 | 0.08 | |
16 | 1,4-Dioxane | 694 | 1.46 | 709 | 691 | 15/370 | 0.16 |
CHCl3 | 690 | 1.42 | 709 | 688 | 19/462 | 0.17 | |
DMF | 681 | 6.52 | 699 | 681 | 18/438 | 0.15 | |
DMSO | 681 | 1.39 | 702 | 683 | 21/511 | 0.16 | |
17 | 1,4-Dioxane | 709 | 1.61 | 723 | 710 | 14/340 | 0.09 |
CHCl3 | 704 | 1.78 | 724 | 688 | 20/487 | 0.13 | |
DMF | 696 | 1.28 | 711 | 688 | 15/365 | 0.14 | |
DMSO | 696 | 1.37 | 717 | 688 | 21/511 | 0.14 |
An absorption band maxima located around 681, 687 and 702 nm, with high molar extinction coefficient values (ɛ) in agreement with π−π* transitions, could be observed in the squaraines 11, 16 and 17, respectively. It can also be seen that the electronic structure plays a fundamental role in the absorption spectra of the squaraine dyes. The symmetrical indolium derivative 11 presents the lower absorption maxima in all studied solvents despite of the dyes 16 and 17, which present a bathochromic absorption, indicating more delocalized electronic structure due to the sulfur atom in the dye structure. For all dyes studied, a small solvatochromic effect could be observed (13–16 nm) in the non-protic environments, which can be due to solvent effects determined by the electronic polarizability of the solvent and the molecular polarizability resulting from reorientation of solvent dipoles. The latter property is a function of the static dielectric constant, ɛ. However, the ethanol (to dye 11), dimethylformamide and dimethylsulfoxide (to dyes 16 and 17) present a blue-shifted band in relation to the other solvents, which probably indicates that specific interactions are produced by one or a few neighboring molecules, and are determined by the specific chemical properties of both the fluorophore and solvent, such as hydrogen bonding or preferential solvation [32].
The curves of fluorescence were obtained using the absorption maxima as the excitation wavelengths. One main emission band located around 694, 703 and 717 nm could be observed in the squaraines 11, 16 and 17, respectively, where no aggregation in the studied organic solvents was detected [31]. The similar solvatochromic effect and the red shift depending on the electronic structure was also observed in the excited state in despite of the absorption data. Concerning similar structures presented in the literature, the dyes 11 and 16 presented higher quantum yields in all studied solvents (Φfl = 0.19 to dye 11 and Φfl = 0.17 to dye 16, Table 1). The excitation spectra were also obtained and are quite similar to the UV–Vis absorption spectra, which indicate that no significative conformational or electronic changes took place in the excited state (Figs. S20–S22, Supporting information).
2.3 Photophysical characterization of free dyes in buffer
Proteins can be covalently or non-covalently labeled with different fluorophores. The latter is applied in some biochemical and biomedical applications for investigations of conformational changes in proteins or determination of drug-binding constants [18]. In this way, several works have been suggesting that squaraine dyes can occupy common hydrophobic binding sites on the protein to form stable conjugates, which can enhance the squaraine fluorescence emission and be used for the protein determination [31,33]. Herein, the spectral properties of squaraines 11, 16 and 17 upon binding to bovine serum albumin (BSA) as a model protein were investigated. The fluorescence spectra of squaraine dyes associated with different concentration of BSA are shown in Figs. 4–6. In the absence of BSA, it was observed that the squaraine dyes are almost nonfluorescent. On the other hand, the fluorescence intensity increases by addition of BSA in the phosphate buffer solution (PBS)/squaraine dye solution (BSAIf/If) by a factor from 6 up to 49 (Table 2). An additional emission band could be observed, located at 750 nm in low BSA content. It is well known that the self-association of dyes in solution is a usual phenomenon in dye chemistry owing to strong intermolecular van der Waals-like attractive forces between the molecules, such as cyanines and squaraines [34], which allows fluorescence emission with spectral shifts when compared to the monomeric species. However, a red shift emission band located at the same position could be detected to the PBS solution without the squaraine dyes (control solution). In this way, we are not able to attribute this emission band as being due to the squaraine dye aggregates in solution.
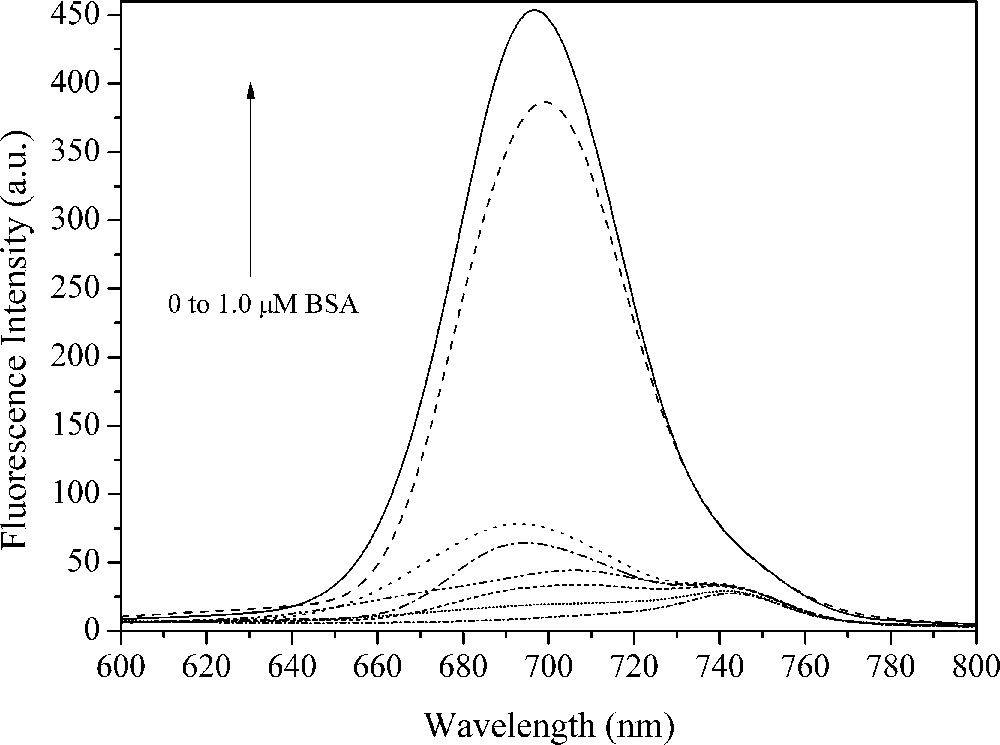
Fluorescence spectra of 2 μM squaraine dye 11 associated with different concentrations of bovine serum albumin (BSA) in pH = 7.0 (0, 0.02, 0.04, 0.06, 0.14, 0.20, 0.80 and 1.0 μM BSA) at room temperature.

Fluorescence spectra of 2 μM squaraine dye 16 associated with different concentrations of bovine serum albumin (BSA) in pH = 7.0 (0, 0.02, 0.04, 0.06, 0.10, 0.12, 0.16, 0.20, 0.40 and 1.0 μM BSA) at room temperature.
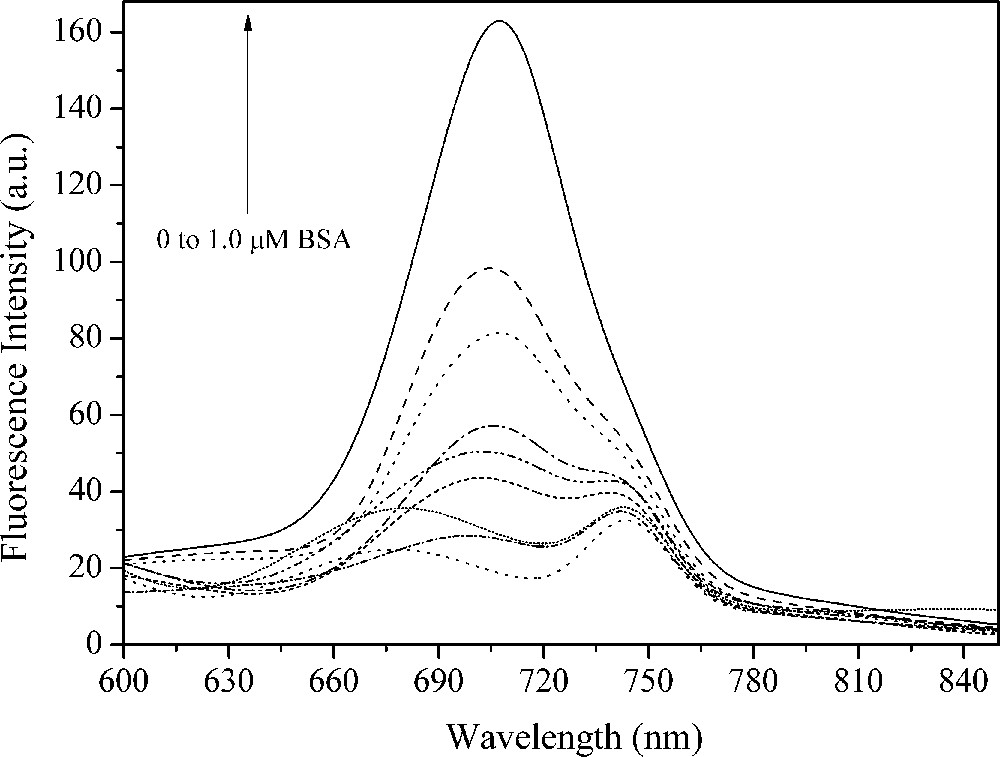
Fluorescence spectra of 2 μM squaraine dye 17 associated with different concentrations of bovine serum albumin (BSA) in pH = 7.0 (0, 0.04, 0.06, 0.10, 0.12, 0.16, 0.20, 0.60 and 1.0 μM BSA) at room temperature.
Relevant photophysical data of the squaraine dyes 8, 13 and 14 in phosphate buffer solution (PBS) buffer.
In bovine serum albumin (BSA) absence | In bovine serum albumin (BSA) presencea | ||||
Dye | λem (nm) | If (a.u.) | BSAλem (nm) | BSAIf (a.u.) | BSAIf/If |
11 | 689 | 13 | 697 | 463 | 36 |
16 | 689 | 13 | 698 | 634 | 49 |
17 | 696 | 27 | 707 | 167 | 6 |
a BSA concentration of 1 μM.
3 Conclusions
In summary, we have successfully synthesized new symmetrical and unsymmetrical squaraine dyes based on indolium and benzothiazolium moieties. Symmetrical squaraine dyes 11 and 17 were prepared by base-catalyzed condensation reaction between 2.0 equivalents of 3H-indolium or benzothiazolium salts, respectively, with 1.0 equivalent of dicyanomethylene squarate 8, derived from squaric acid (7). Unsymmetrical squaraine dye 16 was prepared in two steps via mono-squaraine intermediate 15, obtained from condensation reaction between 1.0 equivalent of 3H-indolium salt 10 and 1.0 equivalent of dicyanomethylene squarate 8. The dyes presented strong absorption and fluorescence emission between 680 nm and 724 nm with high molar extinction coefficient values (ɛ > 105) and fluorescence quantum yields between 0.08 and 0.19. In addition, no aggregation could be observed in solution of organic solvents. Additionally, the squaraines displayed a considerable increase in fluorescence emission by a factor of up 6 to 49 in the presence of albumin from bovine serum.
4 Experimental
4.1 General
1H and 13C NMR spectra were recorded on a Varian Inova 300 MHz spectrometer. The chemical shifts are expressed as δ (ppm) relative to tetramethylsilane as an internal standard and J standard values are given in Hz. IR spectra were measured on a Mattson Galaxy Series FT-IR 3000 (model 3020). The UV–Vis absorption and fluorescence emission spectra were obtained on a Shimadzu UV-2450 spectrophotometer and a Shimadzu RF-5301PC spectrofluorometer, respectively. Spectroscopic grade solvents (Merck or Aldrich) were used in fluorescence emission and UV–Vis absorption spectroscopy measurements. The quantum yield of fluorescence (Φfl) was measured at 25 °C in spectroscopic grade solvents with a solution with absorbance intensity lower than 0.05. Zinc phthalocyanine (ZnPc) was synthesized according to the literature [35] and used as quantum yield standard (Φfl = 0.20 in dimethylsulfoxide [DMSO]) [36]. Purification by column chromatography was carried out on silica gel 60 (70–230 mesh). Analytical thin-layer chromatography (TLC) was conducted on Merck aluminum plates with 0.2 mm of silica gel 60F-254. 3,4-dibutoxycyclobut-3-ene-1,2-dione (1), triethylammonium-2-butoxy-3-dicyanomethylene-4-oxocyclobut-1-en-1-olate (8), 1-octyl-2,3,3-trimethyl-3H-indolium iodide (10), 2-methylbenzothiazole (13) and N-octyl-2-methylbenzothiazolium iodide (14) were prepared according to the procedures described in this work. Squaric acid (7), 2,3,3-trimethylindol (9), 2-aminothiophenol (12), 1-iodooctane, malononitrile, phthalonitrile, zinc acetate and nitrobenzene were purchased from Sigma Aldrich and used without purification. BSA fraction V was purchased from Alamar Tecno-Científica LTDA.
4.1.1 3,4-Dibutoxycyclobut-3-ene-1,2-dione (1)
In a 100 mL round bottom flask with reflux condenser, a mixture of squaric acid (7) (1.0 g, 8.77 mmol) and dry n-butanol (30 mL) was heated at reflux for 24 h using a Dean Stark trap. After solvent removal under reduced pressure, the product was purified by column chromatography using silica gel and hexane:ethyl acetate as eluent mixture (8:2) to give 1.39 g (6.17 mmol, 70% yield) of 3,4-dibutoxycyclobut-3-ene-1,2-dione (1). The spectroscopic data of 1 (1H NMR and 13C NMR) were in agreement with those reported previously in literature [25].
4.1.2 Triethylammonium-2-butoxy-3-dicyanomethylene-4-oxocyclobut-1-en-1-olate (8)
To a solution of 3,4-dibutoxycyclobut-3-ene-1,2-dione (1) (320 mg, 1.42 mmol) and malononitrile (92 mg, 1.39 mmol) in 6.0 mL of benzene was added, dropwise with stirring, 0.19 mL of triethylamine. The mixture was stirred at room temperature for 20 min. The solvent was removed under reduced pressure to afford the dicyanomethylene 8, which was used without any further purification in the next step. The spectroscopic data of 8 (1H NMR and 13C NMR) were in agreement with those reported previously in literature [26].
4.1.3 1-Octyl-2,3,3-trimethyl-3H-indolium iodide (10)
A mixture of 1-iodooctane (1.2 g, 5.0 mmol) and 2,3,3-trimethylindol (9) (0.16 g, 1.0 mmol) was heated at 145 °C during 3 h. After cooling, the resultant mixture was purified by column chromatography using silica gel and CH2Cl2:MeOH as eluent mixture (8:2) to give 0.2 g (0.75 mmol, 75% yield) of 1-octyl-2,3,3-trimethyl-3H-indolium iodide (10). The spectroscopic data of 10 (1H NMR and 13C NMR) were in agreement with those reported previously in literature [37].
4.1.4 Squaraine dye (11)
A mixture of 8 (233 mg, 0.73 mmol) and 10 (400 mg, 1.46 mmol) was refluxed in 16 mL of an n-butanol:pyridine mixture (1:1, v/v) for 12 h. The solvent was removed under reduced pressure and the residue was purified by column chromatography using silica gel and CHCl3:ethyl acetate as eluent mixture (95:5) to afford 191 mg (0.29 mmol, 39% yield) of dye 11 as green solid; 1H NMR (300 MHz, CDCl3) δ 7.36 (4H, m), 7.20 (2H, t, J = 7.2 Hz), 7.06 (2H, d, J = 8.1 Hz), 6.53 (2H, s), 4.02 (4H, t, J = 7.2 Hz), 1.79 (4H, m), 1.78 (12H, m), 1.46 (4H, m), 1.27 (20H, m), 0.87 (6H, t, J = 6.9 Hz); 13C NMR (75 MHz, CDCl3) δ 173.3, 171.9, 167.9, 166.7, 142.6, 142.2, 128.2, 124.7, 122.4, 119.2, 110.3, 89.3, 49.5, 44.6, 31.9, 29.5, 29.3, 27.5, 26.9, 26.8, 22.8, 14.3; IR (KBr) 2925, 2855, 2193, 1492, 1454, 1359, 1285, 1111 cm−1; Analysis: Calcd. for C45H56N4O: C, 80.80; H, 8.44; N, 8.38. Found: C, 80.75; H, 8.40; N, 8.36.
4.1.5 2-Methylbenzothiazole (13)
To a solution of 2-aminothiophenol (12), (0.31 g, 2.5 mmol) in 2.5 mL of toluene was added dropwise 0.18 mL of acetyl chloride (0.2 g, 2.5 mmol). The mixture was kept under stirring at room temperature during 25 minutes. Next, the mixture was diluted with ethyl acetate (10 mL) and sat. aq. NaHCO3 (5 mL). The organic layer was separated and the aqueous layer extracted with ethyl acetate (3 × 5 mL). The combined extracts were washed with H2O (3 × 5 mL), dried (Na2SO4) and concentrated under reduced pressure. The crude product was purified by column chromatography using silica gel and hexane:ethyl acetate as eluent mixture (8:2) to give 0.32 g (2.12 mmol, 85% yield) of 2-methylbenzothiazole (13). The spectroscopic data of 13 (1H NMR and 13C NMR) were in agreement with those reported previously in literature [29].
4.1.6 N-Octyl-2-methylbenzothiazolium iodide (14)
A mixture of 1-iodooctane (1.2 g, 5.0 mmol) and 2-methylbenzothiazole (13) (0.15 g, 1 mmol) was heated at 145 °C during 3 h. After cooling, the resultant mixture was purified by column chromatography using silica gel and CH2Cl2:MeOH as eluent mixture (8:2) to give 0.18 g (0.68 mmol, 68% yield) of N-octyl-2-methylbenzothiazolium iodide (14). The spectroscopic data of 14 (1H NMR and 13C NMR) were in agreement with those reported previously in literature [37].
4.1.7 Mono-squaraine (15)
A solution of 8 (229 mg, 0.72 mmol) and 10 (0.15 g, 0.55 mmol) in 2 mL of EtOH, in presence of 0.1 mL of Et3N, was heated at 70 °C for 1 h. After removal of solvent, the crude product containing mainly the mono-squaraine 15 was used in the next step without further purification.
4.1.8 Unsymmetrical squaraine dye (16)
A mixture of 14 (204 mg, 0.77 mmol) and mono-squaraine 15 (400 mg, 0.77 mmol) was refluxed in 30 mL of n-butanol:benzene mixture (1:1, v/v) for 18 h using Dean-Stark trap. The solvent was removed under reduced pressure and the residue was purified by column chromatography using silica gel and CHCl3:ethyl acetate as eluent mixture (8:2) to afford 91 mg (0.14 mmol, 25% yield from 10) of dye 16 as green solid; 1H NMR (300 MHz, CDCl3) δ 7.64 (1H, d, J = 7.8 Hz), 7.49 (1H, t, J = 8.4 Hz), 7.32 (4H, m), 7.15 (1H, t, J = 7.5 Hz), 6.99 (1H, d, J = 7.8 Hz), 6.54 (1H, s), 6.31 (1H, s), 4.21 (2H, t, J = 7.2 Hz), 3.94 (2H, t, J = 7.2 Hz), 1.78 (4H, m), 1.75 (6H, m), 1.42 (4H, m), 1.27 (16H, m), 0.87 (6H, t, J = 6.9 Hz); 13C NMR (75 MHz, CDCl3) δ 174.0, 169.8, 166.4, 165.4, 163.6, 162.3, 142.5, 142.2, 140.8, 128.8, 128.0, 127.9, 125.4, 123.8, 122.7, 122.3, 119.2, 118.9, 112.7, 109.6, 48.9, 47.4, 44.2, 41.1, 31.9, 31.9, 29.6, 29.4, 29.3, 29.2, 27.9, 27.3, 27.1, 26.9, 22.8, 22.7, 14.3; IR (KBr) 2925, 2854, 2192, 1506, 1457, 1268, 1115 cm−1; Analysis: Calcd. for C42H50N4OS: C, 76.55; H, 7.65; N, 8.50. Found: C, 76.03; H, 7.85; N, 8.12.
4.1.9 Squaraine dye (17)
A mixture of 8 (243 mg, 0.76 mmol) and 14 (400 mg, 1.52 mmol) was refluxed in 17 mL of n-butanol:pyridine mixture (1:1, v/v) for 12 h. The solvent was removed under reduced pressure and the residue was purified by column chromatography using silica gel and CHCl3:ethyl acetate as eluent mixture (9:1) to afford 208 mg (0.32 mmol, 42% yield) of dye 17 as dark solid; 1H NMR (300 MHz, CDCl3) δ 7.55 (2H, d, J = 7.8 Hz), 7.41 (2H, t, J = 7.8), 7.24 (4H, m), 6.25 (2H, s), 4.11 (4H, t, J = 7.2 Hz), 1.82 (4H, m), 1.46 (4H, m), 1.27 (16H, m), 0.87 (6H, t, J = 6.9 Hz); 13C NMR (75 MHz, CDCl3) δ 174.2, 163.9, 162.6, 160.6, 141.0, 128.5, 127.6, 124.7, 122.4, 118.9, 112.1, 87.0, 47.0, 31.9, 29.4, 29.2, 27.7, 27.0, 22.7, 14.2; IR (KBr) 2926, 2852, 2185, 1456, 1434, 1257, 1154, 1130 cm−1; Analysis: Calcd. for C39H44N4OS2: C, 72.18; H, 6.83; N, 8.63. Found: C, 73.19; H, 6.58; N, 8.45.
4.2 Synthesis of the zinc phthalocyanine (ZnPc)
A mixture of phthalonitrile (4.3 g, 33 mmol) and zinc acetate (2.5 g, 114 mmol) in nitrobenzene was refluxed during 5 h. After cooling, the resulting mixture was filtered and washed with ethanol and acetone to give a violet powder. Next, the solid was purified by Soxhlet extraction with toluene, ethanol and acetone. The resulting solid was treated with hot aqueous solution of 10% aq. HCl and subsequently hot aqueous solution of 10% aq. NaOH to give 4,0 g (83% yield) of ZnPc. The expected structure is in agreement with the literature (λabs = 673 nm and λem = 678 nm in DMSO) [35].
4.3 Bovine serum albumin association
Different BSA concentrations (BSA fraction V) were labeled with the squaraine dyes in a concentration of 2 μM. In a previous solution of the dyes, in PBS, was added different volumes of BSA solution, also in PBS. The final solution was gentle stirred for 1 h. The fluorescence spectra were recorded with increasing the BSA concentration until concentration where the fluorescence intensity saturates.
Acknowledgements
The authors thank the Conselho Nacional de Desenvolvimento Científico e Tecnológico (CNPq) and the Instituto Nacional de Inovação em Diagnósticos para Saúde Pública (INDI-Saúde) for financial support.