1 Introduction
Riboflavin is a type of flavin, a group of compounds that take part in biological processes such as photosynthesis or phototropism. It can be found in dietary products, enzymes and photoreceptors [1,2]. Some research has shown that riboflavin derivatives may have anticancer properties and may also be useful in treating other diseases [3–5]. The most prominent feature of the riboflavin molecule, as exemplified by its biological role as an electron shuttle, is its ability to undergo redox reactions [6]. Research on the photophysical and photochemical properties of riboflavin showed that, under UV–vis, riboflavin becomes activated (triplet state), producing a superoxide anion-radical [2,7].
Natural antioxidants are used to remove harmful compounds in the form of radicals from organisms or other media by a natural, biological process. Riboflavin (vitamin B2), discovered in 1932 by Warburg and Christian, is important for reduction and oxidation processes. Its antioxidant properties depend on the reaction conditions, time of reaction, concentration and pH of the reaction medium [8]. It is important to examine the reduction and oxidation reactions of riboflavin and its antioxidant properties using different electrochemical methods [9–13] to determine the potential use of riboflavin as an antioxidant in polymers. Oxidative ageing of the polymer is one of common problems in polymer technology. The result of the oxidation reaction occurs as loss of mechanical properties, molecular mass, chalking and surface cracks. Fundamentally, there are approaches to prevent the oxidation reaction [14–18]. Presently, the anti-ageing agents added to elastomers are derivatives of aromatic amines and phosphate derivatives. These substances are definitely not environmentally friendly, and some of them are carcinogenic or corrosive [19–21]. It seems that natural antioxidants with oxygen, such as phenol derivatives that can be extracted from plants, are a more appropriate solution.
2 Materials and methods
2.1 Chemicals
The examined substrate, riboflavin (vitamin B2), was obtained from Sigma Aldrich.
The following reagents were used:
- • acetonitrile (CH3CN) pure p.a. from POCh Gliwice, Poland;
- • N,N-dimethylformamide (DMF) pure p.a. from Chempur, Poland;
- • methanol (CH3OH) pure p.a. from Chempur, Poland;
- • tetrabutylammonium perchlorate (C4H9)4NClO4) from Fluka, which was used as a supporting electrolyte in organic solvent;
- • sodium perchlorate (NaClO4·H2O) pure p.a. from Fluka, which was used as a supporting electrolyte in aqueous solvent.
Ethylene-propylene rubber (EPM, Dutral CO - 054) was obtained from Montedison Ferrara–Italy. Dicumyl peroxide (DCP, Fluka) was used as a cross-linking agent, 1,3,5-triallyl-1,3,5-triazine-2,4,6(1H,3H,5H)-trione (Sigma Aldrich Chemie GmbH) was used as a co-agent of cross-linking and hexadecyltrimethylammonium bromide (CTBA, Sigma Aldrich Chemie GmbH) was used as a dispersing agent. Areosil 380 silica (Degussa) was used as a filler.
The anti-ageing substances used included the following flavonoid derivatives: riboflavin (Sigma Aldrich). The compositions of elastomer blends are given in Table 1.
Composition of EPM elastomer blends containing riboflavin.
Composition | (phr) |
EPM | 100 |
DCP | 2.00 |
CTAB | 2.00 |
1,3,5-Triallyl-1,3,5-triazine-2,4,6 (1H, 3H, 5H) | 0 0.5 |
A380 | 0 30 |
Riboflavin | 1.25 |
2.2 Methods of experimental investigation
Cyclic voltammetry (CV) and differential pulse voltammetry (DPV) measurements were performed using an Autolab analytical unit (Eco Chemie, Holland). A three-electrode system with platinum test and auxiliary electrodes was used for the measurements. The potential of the tested electrode was measured versus a ferricinium/ferrocene reference electrode (Fc+/Fc), the standard potential of which is defined as zero, independent of the solvent used. In aqueous solvent the potential of the tested electrode was measured versus a saturated calomel electrode (SCE).
Prior to taking the measurements, all solutions were degassed with argon. During the measurements, an argon atmosphere was maintained over the solution. The effect of the polarisation rate on the electro-oxidation of riboflavin in an anhydrous medium was assessed.
Rubber blends were prepared using a laboratory mixing mill with rolls having a length (L) = 330 mm and a diameter (D) = 140 mm. The rotation speed of the front roll (Vp) was 20 rpm, the friction was 1.1 the average temperature of rolls was about 40 °C.
The vulcanisation of rubber blends was performed using steel vulcanisation moulds placed between the shelves of an electrically heated hydraulic press. Teflon films were used as spacers to prevent the adherence of blends to the press plates. Samples were vulcanised at 160 °C and 15 MPa for 30 min.
The density of crosslinks in the vulcanizate network was determined by the equilibrium swelling method. The vulcanised were subjected to equilibrium swelling in toluene for 48 h at room temperature. The swollen samples were then weighed on a torsion balance, dried in a dryer at 60 °C to a constant weight and reweighed after 48 h. The cross-linking density was determined using Flory-Rehner's equation:
(1) |
Using the elastomer-solvent interactions, the cross-linking density was μ = 0.501 + 0.273 Vr, where Vr is the volume fraction of the elastomer in the swollen gel.
The tensile strength of the vulcanizates was tested according to PN-ISO 37:1998 with a ZWICK tester (model 1435) for dumbbell w-3.
Ageing characteristics were determined according to PN-82/C-04216. Samples were subjected to circulated air at an elevated temperature (353 K) for 7 days in a dryer with thermo-circulation. UV ageing was performed using an UV 2000 apparatus (Atlas). The measurement lasted for 120 h and consisted of two alternately repeating segments: the daily segment (radiation intensity of 0.7 W/m2, temperature of 60 °C, duration of 8 h) and the night segment (no UV radiation, temperature of 50 °C, duration of 4 h). The ageing coefficient was calculated according to the relationship S = [TS × EB]after ageing/[TS × EB]before ageing, where TS is the tensile strength, EB is the elongation at break and TS and EB are the corresponding values after ageing. The colour of the vulcanizates was measured with a CM-3600d spectrophotometer. The radiation source consisted of four impulse xenon tubes.
The oxygen induction time (OIT) test were performed on a Mettler Toledo DSC instrument. Samples of 4 mg were heated from room temperature up to the test temperature, 220 °C, at 20 °C/min under nitrogen atmosphere. After 5 min at 220 °C, the gas was switched from nitrogen to air at a flow rate of 60 ml/min. When all antioxidants are consumed the sample starts to oxidize producing a deviation in the baseline. The OIT was measured as the time between the gas switch and an intersection with a tangent from the maximum derivate after oxidation has started. Two analyzes on each sample were performed to ensure the accuracy of the result.
3 Results and discussion
3.1 Electrochemical behaviour of riboflavin
The antioxidant properties of riboflavin were examined in different solvents using different electroanalytical methods. Cyclic voltammetry is frequently used for the characterization of electroactive systems [22,23]. The potential at the electrode influences the current, allowing data on the electrochemical reactions at the examined electrode to be obtained. The cyclic voltammograms of riboflavin oxidation are presented in Fig. 1.
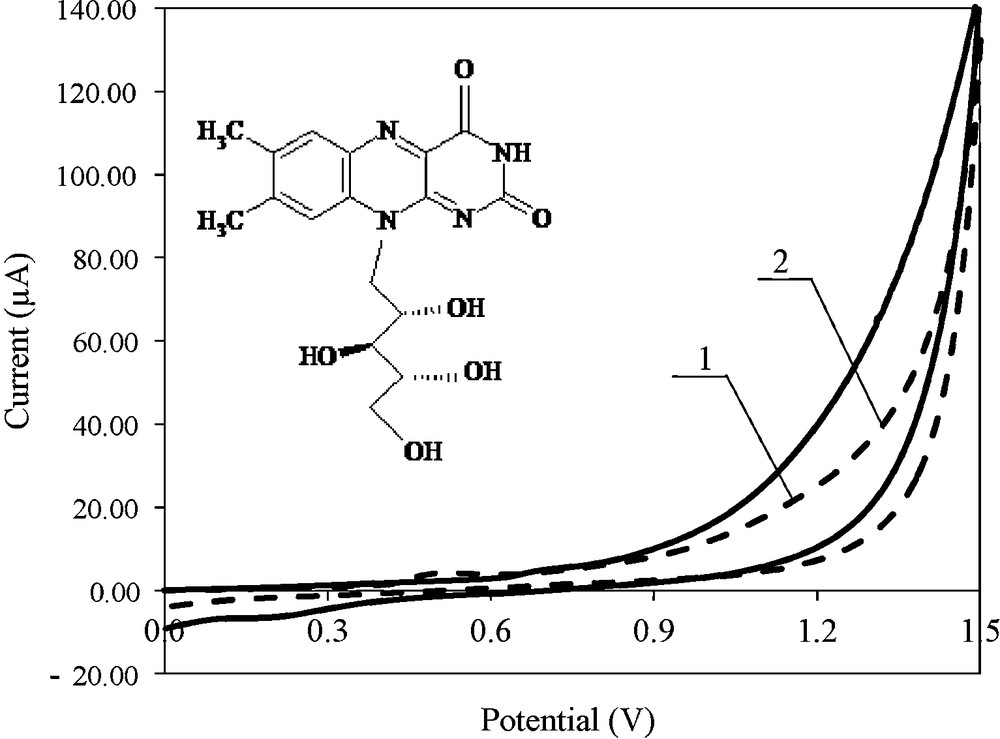
Cyclic voltammograms of riboflavin oxidation at the Pt electrode, v = 0.01 V s−1; c = 1.0 × 10−3 mol L−1 in 0.1 mol L−1 (C4H9)4NClO4 in DMF – curve 1, supporting electrolyte (0.1 mol L−1 (C4H9)4NClO4 in DMF)–2.
Within the range of potentials tested, the basic electrolyte showed no peaks, except for loading the electrical double layer. Only low capacity current was observed (Fig. 1, curve 1). The relationship between the reaction current and the electrode potential suggests that the riboflavin oxidation threshold is 0.9 V. The reaction current has a higher value than the basic electrolyte. However, no electro-oxidation peak was observed for the examined compound.
The half-wave potential (E1/2) of the examined electrochemical reaction in cyclic voltammetry corresponds to the peak potential (Ep) in differential pulse voltammetry.
The cyclic voltammograms shown in Fig. 2 and the differential pulse voltammeograms (higher resolution) shown in Fig. 3 indicate that riboflavin in DMF (curve 1), acetonitrile (curve 2) and methanol (curve 3) is reduced in the course of at least two electrode reactions before reaching the decomposition potential of the electrolyte; at least one electrode reaction was observed in water (curve 4). In DMF and acetonitrile, the examined riboflavin reduction reactions are practically irreversible. In the reverse cycle of the cathode polarisation, a weak oxidation peak was observed. However, in methanol and water, a riboflavin oxidation peak was observed in the reverse cycle of the cathode polarisation, and the examined reactions were reversible. In the reaction medium with protons, riboflavin underwent reduction and oxidation reactions.

Cyclic voltammograms of riboflavin reduction and oxidation at the Pt electrode; c = 1.0 × 10−3 mol L−1 in 0.1 mol L−1 (C4H9)4NClO4 in DMF (curve 1), acetonitrile (curve 2) and methanol (cure 3) and in 0.1 mol L−1 NaClO4 in water (curve 4); v = 0.01 V s−1.
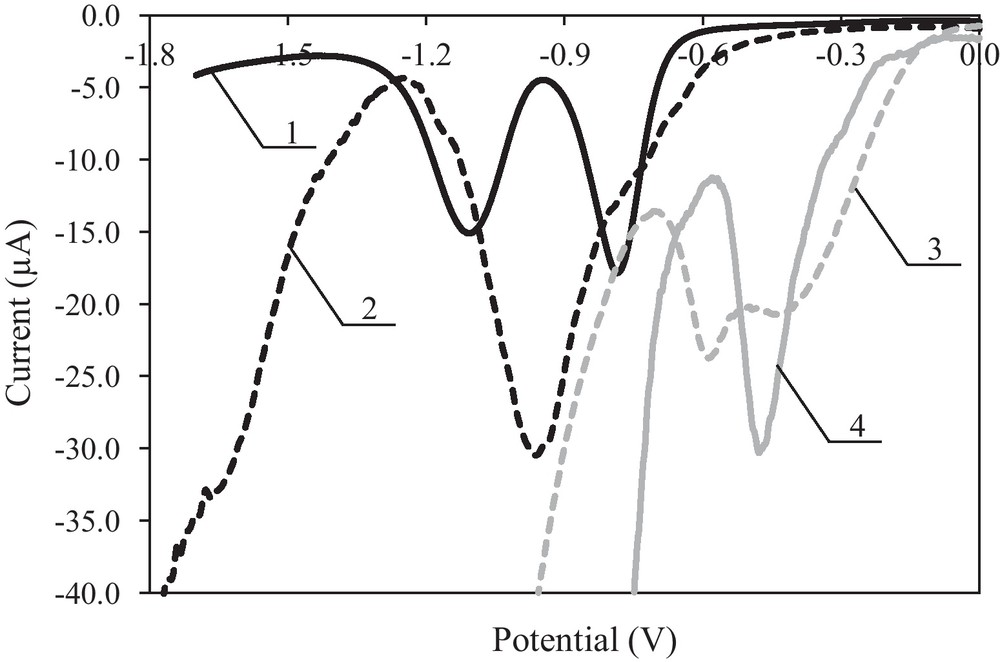
Differential pulse voltammograms differential pulse of riboflavin reduction at the Pt electrode c = 1.0 × 10−3 mol L−1 in 0.1 mol L−1 (C4H9)4NClO4 in DMF (curve 1), acetonitrile (curve 2) and methanol (curve 3) and in 0.1 mol L−1 NaClO4 in water (curve 40; v = 0.01 V s−1.
Cyclic voltammetry (CV) (Fig. 2) and differential pulse voltammetry (DPV) (Fig. 3) was used in the determination the E1/2 and the Ep, respectively (Table 2).
Values of half-wave potential (E1/2) of the first electrode step in riboflavin electroreduction and electrooxidation at the Pt electrode; c = 1.0 × 10−3 mol L−1 in 0.1 mol L−1 (C4H9)4NClO4 in DMF, acetonitrile and methanol and in 0.1 mol L−1 NaClO4 in water.
Solvent | Cyclic voltammetry (CV) | Differential pulse voltammetry (DPV) | ||||
reduction | oxidation | reduction | ||||
EI1/2 (V) | EII1/2 (V) | EI1/2 (V) | EII1/2 (V) | EIp (V) | EIIp (V) | |
DMF | −0.80 | −1.15 | −0.78 | −1.13 | −0.77 | −1.09 |
CH3CN | −0.95 | −1.44 | −0.84 | −1.30 | −0.92 | −1.56 |
CH3OH | −0.43 | −0.59 | −0.15 | −0.76 | −0.41 | −0.56 |
H2O | −0.48 | – | −0.47 | – | −0.45 | – |
The results presented in Table 1 show that riboflavin is most easily reduced and oxidised reversibly in methanol or water; the half-wave potentials of the first electrode reaction were −0.43 V and −0.48 V, respectively, and the electro-oxidation potentials were −0.41 V and −0.45 V, respectively. Riboflavin reduction is more difficult and practically irreversible in an aprotic medium (without water); the half-wave potentials were −0.80 V for DMF and −0.95 V for acetonitrile. In the reverse cycle of the cathode polarisation, weak oxidation peaks were observed; the potentials were −0.78 V and −0.84 V. The antioxidant properties of riboflavin depend on the reaction medium (pH). The influence of polarisation rate on the riboflavin reduction and oxidation reactions in DMF was examined (Fig. 4).

Cyclic voltammograms of riboflavin reduction and oxidation at the Pt electrode; c = 1.0 × 10−3 mol L−1 in 0.1 mol L−1 (C4H9)4NClO4 in DMF for various scan rates; curve 1–v = 0.01 V s−1, 2 – v = 0.05 V s−1, 3 – v = 0.1 V s−1, 4 – v = 0.2 V s−1.
The potential of the first and second electroreduction steps became slightly more negative with increasing polarisation rates. According to this research and the literature data, the following mechanism of riboflavin reduction and oxidation reactions was proposed (Scheme 1) [24,25].
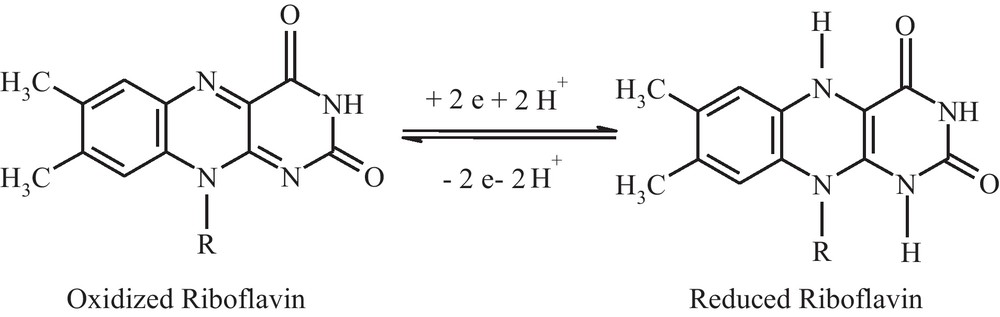
Reduction and oxidation of riboflavin.
3.2 Influence of riboflavin on the stability of a natural rubber EPM vulcanizate
Adding riboflavin had a noticeable effect on the cross-linking density of the ethylene-propylene rubber. After high temperature, natural climate ageing and outdoor weathering, the ve did not change significantly. The ve of the standard vulcanizate decreased by as much as a 48% after ultraviolet ageing, and the concentration of lattice points after heat and natural climate ageing increased by 52 and 37%, respectively (Table 3).
Influence of riboflavin on the cross-linking density of a natural rubber EPM vulcanizate.
Vulcanizate | Before | UV ageing 60 h | Thermal ageing 7 days/70 °C | Weathering 60 h |
νe × 10−5 [mol cm−3] | ||||
EPM | 6.10 | 2.92 | 9.30 | 8.38 |
EPM/riboflavin | 6.47 | 6.35 | 6.92 | 6.55 |
The vulcanizate with vitamin B had a tensile strength 3.8 MPa lower than the reference sample, but no differences were observed in the elongation at break values. The most significant influence on the mechanical properties of the vulcanizates was observed after UV ageing. Riboflavin showed high antioxidant performance both in simulated weathering (ageing index of 0.47) and under focused UV ray (ageing index of 0.63). In case of heat ageing, the observed cross-linking effect increased the tensile strength both in the reference vulcanizate and in the vulcanizate with riboflavin. These results suggest that the riboflavin molecules were activated by radiation during UV ageing and during simulated weathering when sunlight was emulated (Table 4).
Influence of riboflavin on the mechanical properties of a natural rubber EPM vulcanizate.
Vulcanisate | SE100 MPa | TS MPa | Eb % | Ageing parameter | |
Before ageing | EPM | 3.30 | 21.10 | 623 | – |
EPM/riboflavin | 2.55 | 17.30 | 658 | – | |
UV ageing 120h | EPM | 7.19 | 8.37 | 338 | 0.22 |
EPM/riboflavin | 3.25 | 8.81 | 610 | 0.47 | |
Thermal ageing | |||||
7days/70°C | EPM | 4.44 | 22.40 | 617 | 1.05 |
EPM/riboflavin | 2.93 | 21.00 | 676 | 1.24 | |
Weathering | |||||
120h | EPM | 6.61 | 13.50 | 435 | 0.45 |
EPM/riboflavin | 3.13 | 11.10 | 651 | 0.63 |
The OIT test was performed using differential scanning calorimeter (DSC). The test was terminated after an exothermal peak was detected. The exothermal peak correspond to the oxidation of the polymer and the complete consumption of antioxidants in polymer.
The OIT value of EPM vulcanizate was 2.88 min and of EPM/riboflavin was 10.68 min.
The oxidation time of EPM with riboflavin was about three times longer in the comparison with the standard sample ethylene-propylene elastomer. Therefore riboflavin improves considerably the stabilisation of vulcanizate to oxidation processes Fig. 5.
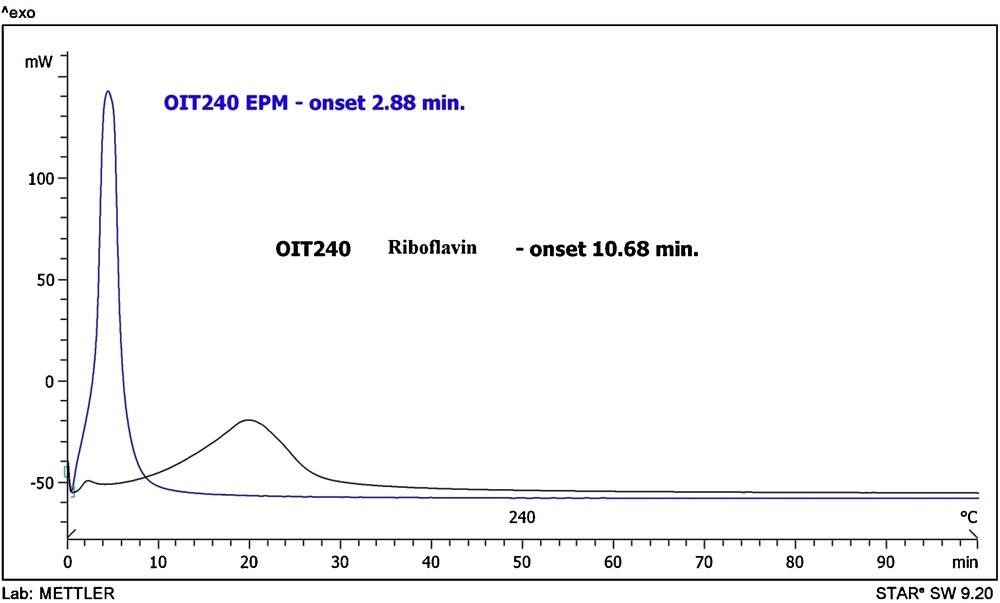
OIT (oxygen induction time) values of EPM vulcanizate.
On the base electrochemistry and other methods applied in this paper we prepare the Scheme 2 of stabilization polymer by riboflavin. The riboflavin quenching active reaction form, which cause the degradation of polymer.

Polymer stabilization polymer by riboflavin.
4 Conclusions
The riboflavin exhibited very high reduction activity in the oxidation process. Riboflavine lengthens oxidative induction time of EPM vulcanizate about 7.8 minutes. Therefore this antioxidant improve resintance of EPM against ageing Natural antioxidants may be a new, environmentally friendly alternative to the aromatic amines commonly used in elastomers. Riboflavin shows antioxidant properties under radiation; therefore, it protects the elastomeric material against sunlight, including UV. Our investigation require extension of research, but we introduce in this paper only preliminary result.
Acknowledgement
This study was supported by Ministry of Science of Higher Education.