1 Introduction
Heterocyclic compounds bearing nitrogen and sulphur have long been the prime focus of synthetic chemistry research due to their broad spectrum of applications in biological, pharmaceutical, and material areas [1,2]. Mainly, thiazolidines containing ketonic group have attracted much attention due to their interesting and unique properties such as protein-nucleic acid interactions, antiviral activity and antidiabetic activity. However, to our knowledge, a few methods were developed to construct polysubstituted thiazolones. Here over, some of these protocols have not been entirely satisfactory because of drawbacks such as low yields, long reaction time and cumbersome experimental processes [3,4]. One pot syntheses are emerging as useful tools for synthesizing small drug-like molecules with several degrees of structural diversity [5–10]. Pioneering work by several research groups in this area has already established the versatility and uniqueness of one pot multicomponent coupling protocols as a powerful methodology for the synthesis of diverse structure scaffolds required in the search of novel therapeutic molecules [11–14].
Thiazolidinone derivatives have been extensively used in drug discovery. They are reported to have anticonvulsant, antibacterial, antiviral and antidiabetic properties. For example, pioglitazone and rosiglitazone were launched recently for type-II diabetes mellitus [15–19]. Presence of these moieties in organic molecules imparts them with the extensive range of biological and pharmacological properties, such as antidiabetic, anticancerous and antimicrobial activities. Moreover, these compounds are also useful as conducting materials. Many of the methods reported for the synthesis of organic compounds are associated with the use of hazardous organic solvents, long reaction time, and lack of general applicability. Thus, we developed a simple one pot synthesis of novel 7-phenyl-1,4,6,7-tetrahydro-thiazolo[5,4-d]pyrimidine-2,5-diones under catalyst free conditions.
2 Experimental
In a typical experiment, thiazolidine-2, 4-dione (10 mmol), aromatic aldehydes (10 mmol) and thiourea (11 mmol) in ionic liquid {[bmim]Br} (15 mL) was stirred at room temperature (80 °C) for the appropriate time. The completion of the reaction was monitored by thin layer chromatography (ethyl acetate: n-hexane: 20: 80). After the completion of reactions, the compound was isolated from the reaction mixture by the addition of ethyl ether (three times × 30 mL) and further washed with brine solution (three times × 30 mL). Then ethyl ether was evaporated under reduced pressure to afford the corresponding product. Structural assignments of the products are based on their 1H NMR, 13C NMR, FT-IR and mass analysis. The analysis of complete spectral and compositional data revealed the formation of corresponding derivatives in 85–95% yield. The ionic liquid used for the transformation was recovered and used for the further reactions.
3 Result and discussion
Thiazolidine-2,4-dione (1) reacts with benzaldehyde (2a) and thiourea (3a) to afford the product 7-Phenyl-1,4,6,7-tetrahydro-thiazolo[5,4-d]pyrimidine-2,5-dione (4a) in excellent yield (Scheme 1, 94%). The reaction of thiazolidine-2,4-dione (1) with benzaldehyde (2a) and thiourea could rapidly form (A) after dehydration. Further dehydration of A will occur and coupling processes to afford the target compound (4a). Screening of the reaction conditions was established suitable solvents, the mole ratio of reactants as well as temperature for the desired MCRs (Table 1). It was exciting that the chosen solvents such as dioxane, N,N–dimethylformamide (DMF), acetonitrile (CH3CN), dimethylsulfoxide (DMSO), toluene, etc. were suitable for the MCRs (Table 1, entries 1–10). Ionic liquid {[bmim]Br} proved to be the best among them (Table 1, entry 10), while under solvent free conditions, a complex result was obtained (Table 1, entry 5). To modulate the ratio of reactants and improve the yield, we examined various ratios of thiazolidine-2, 4-dione (1), benzaldehyde (2a) and thiourea (3a) by using ionic liquid {[bmim]Br} as a solvent (Table 1, entries 10–15). The best result obtained when the ratio of thiazolidine-2, 4-diones (1), benzaldehyde (2a) and phenol (3a) is 1:1:1.1 to afford the product 4a i.e. entry 12. Further, optimization of temperature was done (Table 1, entries 16–18) and we found the best yield was obtained at 80 °C (entry 17).
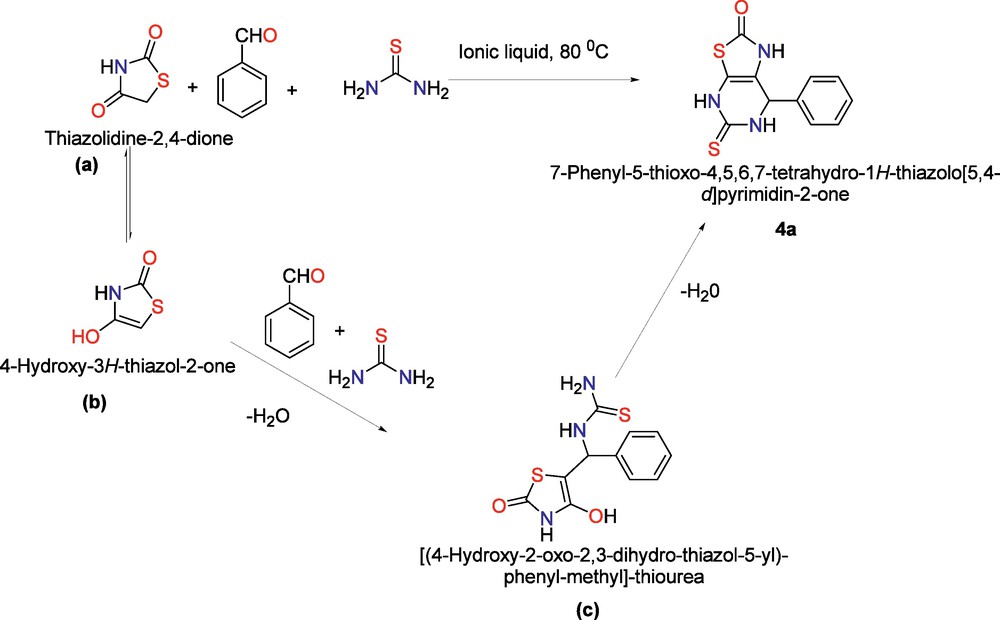
Mechanism for the synthesis of 7-phenyl-1,4,6,7-tetrahydro-thiazolo[5,4-d]pyrimidine-2,5-diones.
Optimization of reaction conditions (solvent, mole ratio and temperature of the reactants) for the catalyst free multicomponent reactionsa.
S. no. | Solvent | Mole ratio (1:2a:3a) | t (h) | Temp (°C) | Yield (%) |
1 | Dioxane | 1:1:1 | 6 | 30 | 65 |
2 | CH3CN | 1:1:1 | 7 | 30 | 64 |
3 | DMF | 1:1:1 | 14 | 30 | 65 |
4 | Toluene | 1:1:1 | 8 | 30 | 50 |
5 | None | 1:1:1 | 18 | 30 | Complex |
6 | DMSO | 1:1:1 | 16 | 30 | 55 |
7 | THF | 1:1:1 | 8 | 30 | 70 |
8 | [NEt3][Ac] | 1:1:1 | 4 | 30 | 80 |
9 | [bmim][Cl] | 1:1:1 | 4 | 30 | 78 |
10 | [bmim][Br] | 1:1:1 | 2.5 | 30 | 80 |
11 | [bmim][Br] | 1:1:1.1 | 2.5 | 30 | 84 |
12 | [bmim][Br] | 1:1:1.2 | 2.5 | 30 | 80 |
13 | [bmim][Br] | 1:1:1.3 | 2.5 | 30 | 75 |
14 | [bmim][Br] | 1:1:0.9 | 2.5 | 30 | 72 |
15 | [bmim][Br] | 1:1:0.8 | 2.5 | 30 | 62 |
16 | [bmim][Br] | 1:1:1.1 | 2.5 | 45 | 85 |
17 | [bmim][Br] | 1:1:1.1 | 2.5 | 60 | 90 |
18 | [bmim][Br] | 1:1:1.1 | 2.5 | 80 | 94 |
With the optimized condition in hand, we examine the scope of the multicomponent reaction (Table 2, entries 1–16). We are pleased to find that the reaction proceeded smoothly, and the desired products were afforded in excellent yields. Meaningfully, the substituted group on the thiazolidine ring could not be selectively induced by changing the addition order of the aromatic aldehyde and urea/thiourea under the same employed conditions. The ionic liquid used for the transformation was recovered and used for further reactions and gave good yield for further reactions as shown in Table 3. The mechanism has been justifiesd through a semi-empirical calculation on the basis of their energy and the same have incorporated in the manuscript as Table 4.
Catalyst free one pot synthesis of 9-phenyl-3,9-dihydro-chromeno [2,3-d] thiazol-2-one via MCRs between thiazolidine-2,4-dione, aromatic aldehyde, urea derivative.
S. no. | Reactant2 | Reactant3 | Product | Compound number | t (h) | Yield (%) |
1 | 4a | 2.5 | 94 | |||
2 | 4b | 3.0 | 90 | |||
3 | 4c | 2.0 | 92 | |||
4 | 4d | 2.0 | 92 | |||
5 | 4e | 3.0 | 95 | |||
6 | 4f | 3.5 | 93 | |||
7 | 4g | 4.5 | 88 | |||
8 | 4h | 5.0 | 84 | |||
9 | 4i | 6.0 | 86 | |||
10 | 4j | 7.5 | 82 | |||
11 | 4k | 6.0 | 94 | |||
12 | 4l | 6.0 | 92 | |||
13 | 4m | 6.0 | 90 | |||
14 | 4n | 6.0 | 90 | |||
15 | 4o | 6.5 | 88 | |||
16 | 4p | 6.5 | 84 |
Optimization of the activity of ionic liquid for the synthesis of 4a after reuse.
S. no. | No. of cycle | Yield (%) |
1 | I | 95 |
2 | II | 94 |
3 | III | 94 |
4 | IV | 92 |
5 | V | 92 |
Optimization and various parameters of the synthesized compounds.
Thermodynamic properties | ||||||
Compound | Single point energy (kcal/mol) | Optimization energy (kcal/mol) | Total E (Kcal/mol) | Entropy (Kcal/mol/deg) | Total Free E (Kcal/mol) | H (Kcal/mol/deg) |
1 | 6604.986 | 12.668 | 44.367 | 0.0716 | 22.879 | 0.0137 |
2 | 6681.347 | 17.283 | 50.640 | 0.069 | 29.936 | 0.0120 |
3 | 18193.481 | 36.564 | 129.907 | 0.095 | 101.392 | 0.0326 |
4 | 16901.238 | 21.288 | 113.479 | 0.099 | 83.722 | 0.0338 |
4 Conclusion
In conclusion, a series of biologically and pharmacologically 7-phenyl-1,4,6,7-tetrahydro-thiazolo[5,4-d]pyrimidine-2,5-diones have been synthesized via one pot three component condensation of aromatic aldehyde, urea/thiourea, and thiazolidine-2,4-dione in excellent yields within a short reaction time. The advantages offered by ionic liquid as a solvent versus known organic solvent are:
- • the ionic liquid has high vapor pressure;
- • highly stable;
- • reusable;
- • environmentally benign.
The exploration of ionic liquid for other multicomponent reactions leading to biologically active compounds is underway.
Analytical data of the few selected compounds.
C. no. | Data |
4a | IR (ν in cm−1) 3154.21, 2946.72, 2864.15, 1742.20, 1695.46, 1461.08, 1356.26, 1245.70, 1179.92, 807.37, 652.48; 1H NMR (300 MHz, d-DMSO) δ 8.45 (s, 1H of NH), 7.00–7.19 (m, 5H), δ 4.58 (s, 1H of benzylic proton), 2.54 (s, 1H of NH), 1.98 (s, 1H of NH); 13C NMR (75 MHz, d-DMSO) δ for thiourea carbon (184.6), carbonyl carbon (165.1), aromatic carbon (129.3, 125.7, 120.1), δ for alkenic carbon (113.0, 110.5), benzylic carbon (65.8), HRMS (M+ ion peak) 263.0154. |
4b | IR (ν in cm−1) 3165.43, 2932.45, 2874.32, 1741.05, 1699.46, 1459.52, 1368.06, 1246.98, 1162.01, 816.09, 665.97; 1H NMR (300 MHz, d-DMSO) δ 8.14 (s, 1H of NH), 6.98–7.20 (m, 5H), δ 4.46 (s, 1H of benzylic proton), 2.79 (s, 1H of NH), 1.95 (s, 1H of NH); 13C NMR (75 MHz, d-DMSO) δ for thiourea carbon (182.0), carbonyl carbon (163.4), aromatic carbon (130.1, 123.0, 119.1), δ for alkenic carbon (113.9, 111.6), benzylic carbon (66.0), HRMS (M+ ion peak) 247.3248. |
4c | IR (ν in cm−1) 3165.48, 2932.45, 2872.45, 1746.89, 1699.05, 1469.15, 1378.65, 1260.65, 1170.05, 812.65, 666.01; 1H NMR (300 MHz, d-DMSO) δ 8.65 (s, 1H of NH), 7.01-7.29 (m, 4H), δ 4.65 (s, 1H of benzylic proton), 2.36 (s, 1H of NH), 2.09 (s, 1H of NH); 13C NMR (75 MHz, d-DMSO) δ for thiourea carbon (179.3), carbonyl carbon (162.3), aromatic carbon (131.4, 126.8, 121.7), δ for alkenic carbon (116.5, 111.0), benzylic carbon (62.2), HRMS (M+ ion peak) 308.4565. |
4d | IR (ν in cm−1) 3189.13, 2945.65, 2879.03, 1726.78, 1679.09, 1446.46, 1362.19, 1239.62, 1180.49, 812.36, 666.03; 1H NMR (300 MHz, d-DMSO) δ 8.49 (s, 1H of NH), 7.05–7.35 (m, 4H), δ 4.62 (s, 1H of benzylic proton), 2.63 (s, 1H of NH), 2.00 (s, 1H of NH); 13C NMR (75 MHz, d-DMSO) δ for thiourea carbon (180.4), carbonyl carbon (161.0), aromatic carbon (131.0, 124.9, 121.8), δ for alkenic carbon (114.7, 111.7), benzylic carbon (66.9), HRMS (M+ ion peak) 292.8706. |
4e | IR (ν in cm−1) 3123.35, 2922.33, 2846.72, 1716.29, 1666.13, 1476.43, 1365.08, 1246.35, 1182.69, 832.35; 1H NMR (300 MHz, d-DMSO) δ 8.26 (s, 1H of NH), 6.79–7.33 (m, 4H), δ 4.46 (s, 1H of benzylic proton), 2.60 (s, 1H of NH), 2.32 (s, 3H of methyl), 1.89 (s, 1H of NH); 13C NMR (75 MHz, d-DMSO) δ for thiourea carbon (185.6), carbonyl carbon (167.0), aromatic carbon (130.0, 125.0, 121.7), δ for alkenic carbon (112.6, 111.7), benzylic carbon (63.1), HRMS (M+ ion peak) 239.5987. |
4f | IR (ν in cm−1) 3102.32, 2978.29, 2878.45, 1732.49, 1702.23, 1465.78, 1321.22, 1246.08, 1145.65, 845.38, 615.95; 1H NMR (300 MHz, d-DMSO) δ 8.11 (s, 1H of NH), 6.83–7.29 (m, 4H), δ 4.62 (s, 1H of benzylic proton), 2.46 (s, 1H of NH), 2.12 (s, 3H of methyl), 1.86 (s, 1H of NH); 13C NMR (75 MHz, d-DMSO) δ for thiourea carbon (188.0), carbonyl carbon (164.9), aromatic carbon (131.0, 126.4, 124.4), δ for alkenic carbon (115.7, 113.9), benzylic carbon (60.6), HRMS (M+ ion peak) 277.6598. |
4g | IR (ν in cm−1) 3202.15, 2938.46, 2873.09, 1739.15, 1738.45, 1489.15, 1375.17, 1260.19, 1154.28, 836.97, 666.67; 1H NMR (300 MHz, d-DMSO) δ 8.23 (s, 1H of NH), 6.88–7.10 (m, 4H), δ 4.32 (s, 1H of benzylic proton), 2.35 (s, 1H of NH), 1.89 (s, 1H of NH); 13C NMR (75 MHz, d-DMSO) δ for thiourea carbon (181.6), carbonyl carbon (166.5), aromatic carbon (128.4, 126.3, 122.7), δ for alkenic carbon (115.1, 111.2), benzylic carbon (61.0), HRMS (M+ ion peak) 297.8977. |
4h | IR (ν in cm−1) 3208.65, 2945.68, 2879.46, 1719.18, 1689.39, 1461.08, 1354.72, 1298.15, 1183.36, 835.79, 679.15; 1H NMR (300 MHz, d-DMSO) δ 8.32 (s, 1H of NH), 7.00–7.19 (m, 4H), δ 4.18 (s, 1H of benzylic proton), 2.24 (s, 1H of NH), 1.84 (s, 1H of NH); 13C NMR (75 MHz, d-DMSO) δ for thiourea carbon (186.0), carbonyl carbon (160.9), aromatic carbon (124.7, 122.0, 119.3), δ for alkenic carbon (111.7, 108.7), benzylic carbon (61.1), HRMS (M+ ion peak) 281.0350. |
Acknowledgement
We gratefully acknowledge financial support from University Grant Commission (UGC) and Department of Science and Technology, India.