1 Introduction
Organic molecules, which are not biologically degradable, constitute one of the classes of contaminants, responsible for pollution of water and groundwater. Adsorption technologies are used extensively for the removal of organic and inorganic micropollutants from aqueous solutions. Activated carbons are the most widely used to achieve this operation, but the disadvantage associated with their high regeneration cost increases the request to find other efficient materials, which are easily regenerable [1,2]. Indeed, zeolites, which are inorganic crystalline polymers, can replace activated carbon since these solids have a great capacity of adsorption. They can be modulated easily (variation of pores size, hydrophobicity, acidity, …) and especially are easily regenerable under air. Many investigations were effectuated on natural zeolites for an eventual application like adsorbents. However, they are hydrophilic in nature due to their high content on aluminum; therefore, they are often modified by chemical treatments. Cation exchange with surfactants is by far the most tested on natural zeolites [3–7]. Synthetic zeolites are also very attractive because they offer several possibilities in term of pore size, Si/Al ratio and they can be easily tailored. We find in the literature many works related to adsorption by synthetic zeolites [8–14]. Zeolite Y (FAU) has very interesting adsorption proprieties. This structure is very ventilated (porous volume accounts for 50% of total volume) with pores in form of cages with large openings (7.4 Å) and consequently a good accessibility to the internal sites. Koubaissy et al. [15] studied the adsorption of four nitrophenols (NP) in aqueous solution by three zeolites: BEA (Si/Al ∞), MFI (Si/Al = 500) and FAU (Si/Al = 100). They found that zeolite BEA has the highest adsorption capacity because it is more hydrophobic but the adsorption capacity of FAU is higher than that of MFI, which is more hydrophobic, but it has smaller pore size. The same observation was made by Roostaei et al. [16] in their study; they found that zeolite FAU (Si/Al = 20) had higher adsorption capacity than MFI of Si/Al ratio of ∞. Tsai et al. [17] studied adsorption of bisphenol by zeolite Y (FAU) of Si/Al > 100 at 25 °C in batch reactor. They noticed that the equilibrium time was very short (< 10 min) and this zeolite has a good capacity of adsorption. Kamble et al. [4] synthesized a zeolite Y from fly-ashes with Si/Al ratio of 2.5 which they then modified with the HDTMA. They found that this one was more effective (3.24 times) than a commercial zeolite Y for the elimination of chloro-phenol in aqueous medium. Khalid et al. [18] studied the effect of the Si/Al ratio in the case of the same structure and effect of structure on adsorption capacity of phenol in a concentration domain varying from 0.1 to 1.6 g/L. They found that for the same Faujasite structure, the capacity of adsorption increases with Si/Al ratio, and that for the same Si/Al ratio this structure is more effective than BEA and Mordenite, which have comparable pore size. Braschi et al. [19] and Martucci et al. [20] made the same observations in adsorption of pharmaceuticals from aqueous solution by different zeolitic structures with the same Si/Al ratio. They found that zeolite Y structure had the highest adsorption capacity compared to MFI and MOR.
Adsorption capacities of organic compounds in aqueous solution by zeolite Y are lower than those of activated carbon and other zeolites, which have higher Si/Al ratio and comparable pore size. In order to increase their adsorption capacity, we must increase their hydrophobic character by different chemical modification.
The aim of this work is to study adsorption of organic molecules from aqueous solutions by zeolites Y modified by silylation to improve their hydrophobic character, in order to increase their affinity toward organic molecules, and consequently their capacity of adsorption.
2 Experimental
2.1 Materials
All reagents used in this study are of analytical grade. Zeolites are in protonic form supplied by Zeolyst International (characteristics are listed in Table 1). Modification was carried out by trimethylchlorosilane (TMCS) 99% from Aldrich, Germany. The pollutant used is phenol (99% Aldrich, Germany), phenol solutions was prepared using distillated water. The zeolites were placed in drying oven at 105 °C to remove physisorbed water before their use.
Textural proprieties of the adsorbent.
Zeolite | Si/Al | SBET (m2/g) | Sext (m2/g) | Vt (cm3/g) | Vmic (cm3/g) | Vmes (cm3/g) |
HFAU (5) | 5 | 690 | 25 | 0.357 | 0.267 | 0.09 |
HFAU (5) modified | 670 | 56 | 0.360 | 0.261 | 0.100 | |
HFAU (60) | 60 | 906 | 99 | 0.485 | 0.364 | 0.121 |
HFAU (60) modified | 821 | 109 | 0.473 | 0.331 | 0.142 |
2.2 Characterization methods
Porosity was probed by physisorption of nitrogen at 77 K by a gas adsorption system ASAP 2000 (Micromeritics). The specific zeolite area was determined by the Brunauer–Emmett–Teller approach using 0.10 as the value of maximum relative pressure. The microporous volume was determined by the Dubinin-Raduskevich (D-R) equation and external surface area was obtained by the t-plot method. The adsorbent before and after modification was also characterized using a Shimadzu IR Affinity FT-IR spectrometer with KBr pellets.
2.3 Silylation experiments
Zeolite (10 g) was suspended in 300 mL of anhydrous acetone and was heated under reflux with stirring. Five milliliters of TMCS were added into the mixture after each 1 h and the reaction was carried out for 4 h under reflux at 120 °C. The separation was effectuated by filtration and the sample was collected, washed with acetone, then with distillated water. After that, the zeolite was dried in drying oven at 105 °C before its utilization.
2.4 Adsorption experiments
Adsorption was carried out at room temperature and under atmospheric pressure in batch conditions. It was performed by introducing a mass of zeolite into a glass erlenmeyer of 100 mL, containing 50 mL of an aqueous phenol solution of determined concentration. The mixture was stirred with a magnetic agitation at 150 rpm for the desired period. Then, the separation was effectuated by centrifugation at 6000 rpm during 5 min and the suspension was analyzed by UV–vis spectroscopy at wave length of 270 nm to determine the residual concentration of phenol.
The amount of phenol adsorbed by the adsorbate qads (mg/g) was calculated according to:
(1) |
The adsorption percentage is calculated according to:
(2) |
2.5 Adsorption isotherms
The adsorption equilibrium was evaluated by the models of Langmuir, Freundlich and Dubinin-Raduskevich (D-R). The parameters of each model were calculated by non-linear fitting using Origin 6.0 software.
The simplest model for adsorption events corresponds to the Langmuir isotherm which is used to describe adsorption by homogenous surface is given by:
(3) |
Another simple equation for representing adsorption data is the Freundlich isotherm used to describe adsorption by heterogeneous surface:
(4) |
The model of Dubinin-Raduskevich (D-R) is used to estimate the apparent free energy of adsorption as well as to make a difference between physical and chemical adsorption process. The D-R equation was given by the following relationship:
(5) |
(6) |
The D-R constant β can give the valuable information regarding the mean energy of adsorption (E) by the following equation:
(7) |
The magnitude of E gives the information about the type of adsorption process: physical (1–8 kJ/mol), ion exchange (9–16 kJ/mol) and chemical (> 16 kJ/mol) [23,24].
3 Results and discussion
3.1 Characterization
The FT-IR (Fig. 1) analyses show that after modification, new peaks appear. The first is situated at 2962 cm−1 corresponding to stretching vibration of C–H bonds of the methyl groups, two other peaks are located at 866 and 757 cm−1 due to bending vibrations of the Si–C bonds. This indicates that there is grafting of new groups on the external surface of zeolite by substitution of hydrogen of the external silanols groups with trimethylsilyl groups (Si (CH3)3). One of the consequences of this modification is the decrease in the intensity of the large peak situated between 3000 and 3800 cm−1 corresponding to stretching vibration of hydroxyl groups of physisorbed water and intensity of the peak at 1627 cm−1 characteristic of bending vibration these groups, indicating that there is a diminution of the number of water molecules physisorbed. These results are in agreement with those found in the literature [25–35].

FT-IR spectra of the HFAU (60) before and after modification; (a): before modification, (b): after modification.
Porosity was characterized by adsorption-desorption of nitrogen at 77 K. Textural proprieties of the zeolites are summarized in Table 1. The mesoporous fraction constitutes approximately 30% of total porous volume, which facilitates the diffusion of the molecules towards the internal sites. External surface is very smaller than total surface (BET surface); therefore, only small portion of adsorption surface is easily accessible and that the majority of the sites of adsorption are located inside crystallites. Thus, these zeolites have a greater effectiveness for the adsorption of the molecules of kinetic diameter lower than the openings of the pores (< 7.4 Å). These results also show that the modification has negligible effects on the texture of zeolites (surface and porosity), which is positive for adsorption.
3.2 Effect of the modification on the percentage of adsorption
For an initial concentration of 50 mg/L and an amount of the adsorbent of 10 g/L we found that silylation improves the percentage of adsorption by 31.67% for HFAU (60) and 26.16% for HFAU (5) (Fig. 2). The capacity of adsorption passes from 1.06 to 3.03 mg/g for HFAU (60) and from 0.43 to 0.88 mg/g after modification. This increase in the percentage of adsorption (and the capacity of adsorption) is attributed to the increase in the hydrophobic character of zeolites due to the substitution of the H of the silanols groups by the trimethylsilyl groups ((CH3)3Si–), having for consequence the reduction in the effect of competition between water and phenol for the occupation of the active sites.

Effect of silylation on the rate of adsorption. C0= 50 mg/L; agitation = 150 rpm; pH = 6; adsorbent dosage = 10 g/L and contact time 1 h.
We also found that HFAU (60) has a greater capacity of adsorption than HFAU (5), which is due to the difference in the Si/Al ratio (the greater is the Si/Al ratio the greater is hydrophobic character of the zeolite).
3.3 Kinetic of adsorption
The effect of the contact time is carried out with aim of determining the equilibrium time by fixing the other parameters (agitation: 150 rpm, adsorbent dosage: 10 g/L, pH = 6 which is the pH of distilled water, initial concentration of phenol: 50 mg/L). Fig. 3 shows that adsorption is very fast and that equilibrium is reached after the first minute. The same observations were made by Braschi et al. [19] for the adsorption of sulfonamide antibiotics from water by Y zeolite. This is due to the fact that phenol possesses a kinetic diameter is of 0.6 nm can penetrates easily inside the micropores which have a larger diameter (0.74 nm). The effect of contact time on phenol adsorption onto modified zeolite is illustrated in Fig. 4. It was seen from this figure that the silylation does not affect the kinetics of adsorption. This is obvious because the silylation did not affect the texture of these zeolites.

Effect of contact time on phenol adsorption onto HFAU (60).

Effect of modification on the kinetic on adsorption of HFAU (60).
3.4 Effect of pH on adsorption equilibrium
The study of the effect of the pH on the adsorption equilibrium was carried out for values of pH ranging between 3 and 11. The pH is adjusted by solutions of NaOH and HCl 0.1 M. From Fig. 5, we see that the pH does not affect adsorption in the field ranging between 3 and 9 for the two zeolites. However, adsorbed amount decreases brutally when the pH is higher than 9 especially for the unmodified zeolite. This diminution is caused by the increase in the concentration of phenolates resulting from the more marked dissociation of phenol at basic values of pH. These phenolates are not adsorbed by the zeolite due to their negative charge (Fig. 5).

Effect of the pH on the adsorption equilibrium. Adsorbent dosage = 10 g/L; agitation = 150 prm, contact time = 1 h and C0= 50 mg/L.
3.5 Effect of ionic strength
We also studied the effect of the ionic strength on the adsorption equilibrium of zeolite before and after modification (Fig. 6). For that we used phenol solutions with different NaCl concentrations (0.001, 0.01, 0.1 mol/L). The results obtained in Fig. 7 show that adsorption equilibrium is not affected by ionic strength. On the contrary, we observe a slight increase in the adsorption with the increase in the NaCl concentration. Dong et al. [8], observed the same phenomenon in their study of adsorption of bisphenol-A on a modified zeolite by cation exchange. They attributed this increase to the reduction in the solubility of phenol caused by increase in the concentration of the electrolyte, which is more soluble, leading to reduction in intensity of the interactions adsorbate-solvent. Koubaissy et al. [15], observed that adsorption increases with the reduction in solubility of nitropheonls on zeolite Y with Si/Al ratio of 100.
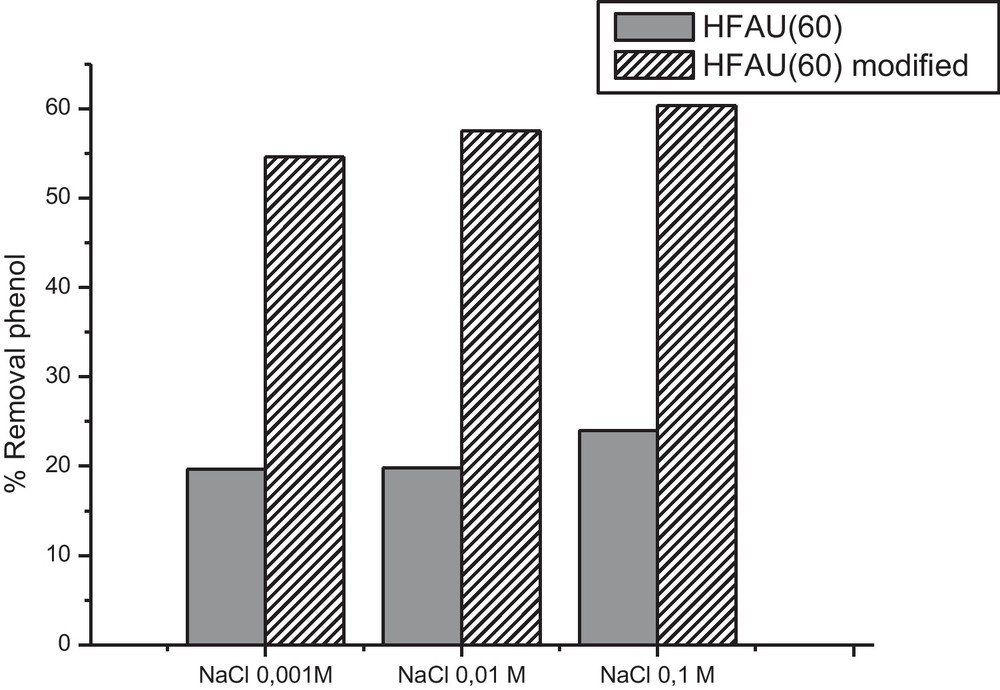
Effect of the ionic strength on the adsorption equilibrium.
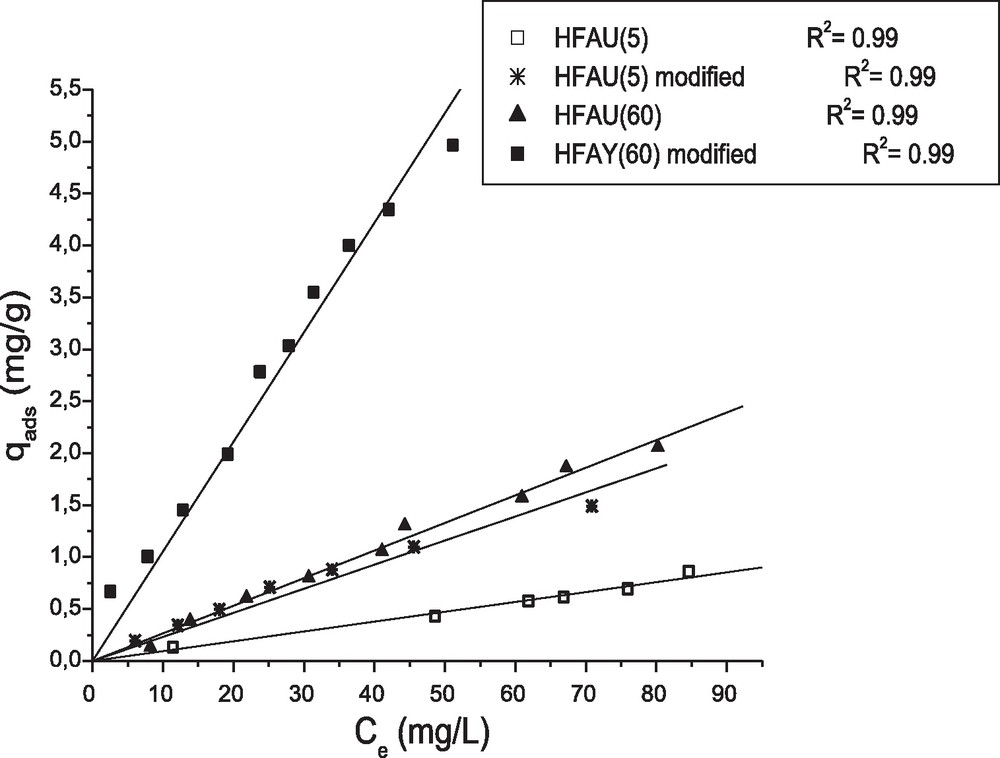
Adsorption isotherms of phenol onto modified and unmodified HFAU (60) and HFAU (5). Agitation = 150 rpm, adsorbent dosage = 10 g/L, pH = 6, contact time = 1 h and T = 20 ± 1 °C.
3.6 Isotherms of adsorption
Adsorption isotherms were carried out by varying the initial concentration of the adsorbate from 10 to 100 mg/L. Obtained curves (Fig. 7) show clearly that isotherms of adsorption for HFAU (60) and HFAU (5) modified or not, are of type C according to the classification of Giles et al. [36] (linear). The same type of isotherms was obtained by Roostaei et al. [16] and Khalid et al. [18]. This linearity illustrates a constant distribution between the two phases, characterized by a coefficient called the distribution coefficient Kd (Kd = 0ads/Ce).
3.7 Modelisation of the adsorption equilibrium
The adsorption equilibrium was modeled by three parameters models: Langmuir, Freundlich and Dubinin-Raduskevich (D-R). Parameters of these models were calculated by the non-linear regression method. The calculations were effectuated by Origin 6.0 software with the function non-linear curve of the software. The results are illustrated on Table 2. As can be seen from Fig. 8, experimental data are well fitted by the three models with a coefficient correlation of approximately 0.99 for each model. The values of E calculated by the D-R model were less than 8 kJ/mol for the two zeolites modified and unmodified, indicating that adsorption of phenol is by physisorption. Values of parameter n of the model of Freundlich are close to 1 which resulting from linearity of isotherms. We also noted that values of the Langmuir coefficient (KL) and Freundlich (KF) coefficient increase with the Si/Al ratio and after modification of the zeolite, indicating that adsorption become more favorable with increase in the hydrophobic character of the adsorbent.
Parameter values of adsorption models.
Zeolite | Model | |||||||||
Langmuir | Freundlich | D-R | ||||||||
qmax (mg/g) | KL(L/mg) | R2 | KF | 1/n | n | R2 | qmax (mg/g) | E (kJ/mol) | R2 | |
HFAU (5) | 13.610 | 0.0007 | 0.995 | 0.010 | 0.935 | 1.017 | 0.995 | 9.546 | 7.001 | 0.992 |
HFAU (5) modified | 4.239 | 0.007 | 0.998 | 0.053 | 0.784 | 1.275 | 0.995 | 18.786 | 7.905 | 0.998 |
HFAU (60) | 24.512 | 0.001 | 0.989 | 0.032 | 0.955 | 1.047 | 0.989 | 45.087 | 6.835 | 0.989 |
HFAU (60) modified | 21.958 | 0.005 | 0.991 | 0.174 | 0.859 | 1.164 | 0.988 | 97.989 | 7.453 | 0.991 |

Modelization of the adsorption equilibrium.
4 Conclusion
The results obtained in this study show that zeolite Y has very interesting properties of adsorption and that the increase in the hydrophobic character increases capacity of adsorption of phenol in aqueous medium. Modification by silylation increases rate of adsorption by approximately 30%, indicating that the hydrophobic character supports the adsorption of phenol to the detriment of water. Analysis carried out by the adsorption of N2 at 77 K showed that the modification does not affect the texture of zeolite. Isotherms of adsorption obtained are all linear and D-R model shows that adsorbent–adsorbate interactions are of physical nature (E < 8 kJ/mol).