1 Introduction
Lectins are proteins of non-immune origin that bind in specific and reversible ways to carbohydrates without modifying them [1,2] and often display the property of agglutinating cells. Lectins are widely distributed in nature and they have been identified in all part of living reign, from microorganisms to fungi, plants and animals. Due to their interactions with glycoproteins, glycolipids and oligosaccharides, lectins are involved in cell-cell communication and they play the crucial role of deciphering the glycocode [3]. Their biological functions are very diverse. Lectins are important players in the innate immunity system of many invertebrates since they are able to differentiate between self and non-self carbohydrates [4]. Lectins are particularly abundant in plants, where they are proposed to be involved in protection against pathogens or feeders [5]. The biological roles of animal lectins have been the most studied; they include among others, glycoprotein trafficking and clearance, development, immune defence, fertility and others [6].
In pathogenic microorganisms, lectins are often involved in host recognition and tissue adhesion [7]. Indeed, all known organisms are covered with arrays of free or covalently attached glycans that interact with receptors, cells and microorganisms. These glycans present a wide range of structural diversity, correlated with variability of possible configurations of monosaccharides and variability of linkages between monosaccharide units, including branching points [8]. The infection strategies of pathogens involve interactions characterized by their high specificity to host glycans and most of the time by multivalency. Interfering with synthetic compounds that mimic the natural oligosaccharides and effectively compete with the attachment site is an attractive anti-infectious strategy [9] that is now developed in many laboratories of glycochemistry.
The present review focuses on the interactions occurring between opportunistic lung pathogens and their host as mediated by soluble lectin and glycoconjugates. The involvement of fucosylated histo-blood group oligosaccharides is discussed as well as the structural basis for the interaction. The knowledge accumulated in the recent years is now utilized for structure-based design of high affinity fucosylated compounds that can compete with the binding of receptors and therefore act as anti-adhesive compounds that can play a role in anti-infectious strategy.
2 Pathogen receptors and human glycans – an evolutionary point of view
Pathogenic organisms such as viruses, bacteria, fungi or parasites express different glycan binding proteins such as toxins, soluble lectins, and lectin domains attached for example on the tip of bacterial pili and flagella or to the surface of viral capsids and envelops [7,10–12]. Evolution that resulted in this variety of receptors has been mirrored by selection of glycans on the host tissue. Although, in the absence of fossils, it is not possible to obtain information from extinct pathogens, it is now accepted that a significant portion of the overall diversity in vertebrate cell-surface glycan structure reflects pathogen-mediated selection processes [13]. The glycans that are present on epithelia as part of mucins, N-glycoproteins or glycolipids can be the target for microbial adhesion, providing convenient points of invasion. However, they can also act as protection against pathogens, and mucins secretions have been shown to adsorb and trap intruding pathogens before they can invade the underlying tissues [14].
In this context, the individual variation of glycans present at the surface of human tissues reflects the pressure of past epidemic or endemic pathogens. Such variations are observed in blood group phenotypes since A, B and O blood groups and Lewis histo-blood groups (Fig. 1) are distinguished by oligosaccharides on the surface of cells. The A, B, and H(O) antigens are complex fucosylated oligosaccharides present on endothelial cells and erythrocytes of all individuals [15]. The ABH antigens are also expressed in saliva, tears, and mucus secretions in the digestive tract of individuals who display the secretor phenotype (80% of the Caucasian population). The biological role of the ABO blood group system remains mysterious but in the 1980s, anthropological surveys suggested that the geographic and racial distribution of human blood groups reflects susceptibility of populations with specific blood types to the plague, cholera, smallpox, malaria and other infectious diseases [16,17]. The biochemical and structural evidences for the direct role of pathogen receptors interaction with ABH(O) oligosaccharides have been however obtained only very recently. Cholera toxin of the El Tor biotype of Vibrio cholera is captured by A and B blood group before entering epithelial cells, resulting in more severe infections for people with blood group O [18,19]. The ABO group also influences susceptibility to severe Plasmodium falciparum malaria due to an A/B oligosaccharides specific adhesin domains that promote rosetting, i.e. transmission of parasites from infected red cells to non-infected ones [20]. Susceptibility to viral gastroenteritis is also ABO phenotype-dependant, with variations of specificity between rotaviruses and the different strains of noroviruses [21].
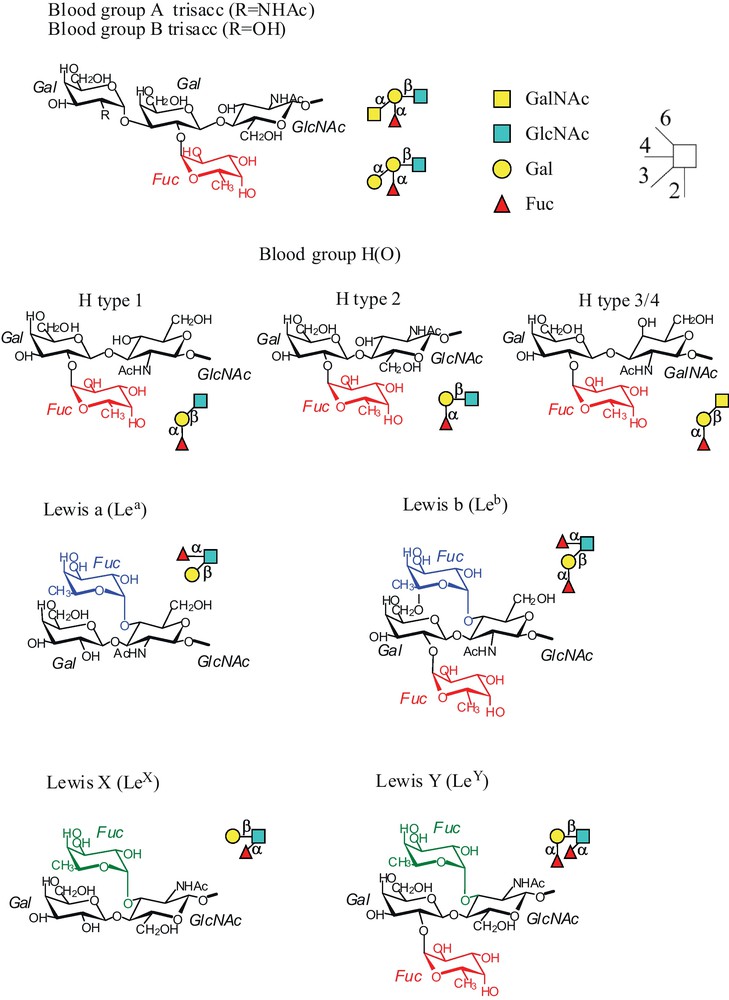
Fucosylated histo-blood group oligosaccharides of interest with fucose residues colored in red when α1-2 linked to galactose, and in green and blue when α1-3 and α1-4 linked to GlcNAc, respectively. The schematic international representation is also given.
3 Lectins from lung pathogens – convergence for binding to fucose
As described above, the histo-blood group correlation with susceptibility of infection has mainly been characterized for pathogens colonizing blood and gastro-intestinal tract. Lung pathogens are in contact with epithelia and mucins that are potential adhesion points. In the recent years, soluble lectins from opportunistic bacteria responsible for acute pneumonia in cystic fibrosis patients or in hospital environment have been described (Table 1).
Different families of bacterial soluble lectins from opportunistic pathogens and their specificity towards oligosaccharides.
Bacteria | Lectin | Higher affinity ligand | Oligomer (number of sites) | Reference |
Pseudomonas aeruginosa | PA-IL (LecA) | αGal4 Gal | Tetramer | [58] |
Pseudomonas aeruginosa | PA-IIL (LecB) | H-type 2/Lewis a | Tetramer | [26] |
Chromobacterium violaceum | CV-IIL | H-type 2 | Tetramer | [24] |
Burkholderia cenocepacia | BC2L-A | Oligomannose | Dimer | [59] |
Burkholderia cenocepacia | BC2L-C N-ter dom | H-type 1/Lewis B | Trimer | [27] |
Burkholderia ambifaria | BambL | H-type 2/Lewis Y | Trimer (6 sites) | [25] |
Interestingly, most of these soluble lectins are fucose-specific and exhibit strong affinity towards human fucosylated ABO or Lewis oligosaccharides. Three different families of soluble fucose-binding bacterial lectins have been identified in Pseudomonas aeruginosa and bacteria from the Burkholderia cepacia complex such as Burkholderia cenocepacia and Burkholderia ambifaria (Fig. 2). LecB from P. aeruginosa is a tetrameric protein that displays an unusually strong micromolar affinity to l-fucose in a tight binding site which requires two Ca2+ ions [22,23]. CV-IIL from the tropical pathogen Chromobacterium violaceum has strong sequence and structure similarities to LecB [24]. BambL from B. ambifaria is a trimeric lectin arranged in a β-propeller fold with two similar binding sites per monomer, resulting in an hexameric arrangement of fucose-binding sites [25]. Previous specificity studies indicate that both lectins bind to a large variety of fucosylated oligosaccharides, with LecB having higher affinity to Lewis a epitope [26] and BambL to H-type 2 epitopes [25]. A third fucose-binding bacterial lectin of interest is the N-terminal domain of Bc2L-C from B. cenocepacia. Bc2L-C is an hexameric lectin, each of the monomer containing two domains with different specificity and oligomerisation state [27,28]. The N-terminal domain assembles as a TNFα-like trimer with strong specificity for H-type1 and Lewis b oligosaccharides [27] while the C-terminal domain is a dimeric mannose-binding lectin [28].
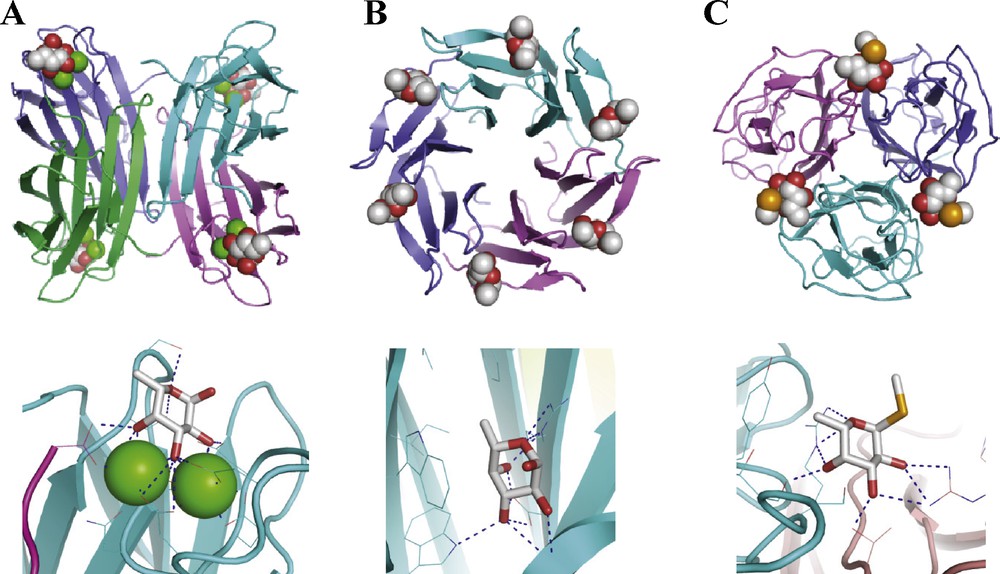
Crystal structure of three bacterial lectins complexed with fucose or fucoside with overview of the oligomeric state with ligand represented as spheres (top) and details of the binding site with ligand represented as stick (bottom) A. LecB/fucose (PDB code 1GZT) [23]. B. BambL/fucose (PDB code 3ZW0) [25]. C. BC2L-C-nt/Me-Se-fucoside (PDB code 2WQ4).[27] Graphics have been prepared with PyMol program.
Although all of the herein described bacterial lectins recognize fucose, their binding modes present strong differences. In LecB, as well as in the related CV-IIL, the specificity towards fucose is due to the presence of two calcium ions that bridges the protein acidic amino acids and the carbohydrate hydroxyl groups. Only monosaccharides presenting hydroxyl groups suite having axial-equatorial-equatorial arrangement, i.e. d-manno or l-galacto configuration, can fit in the coordination scheme. Fucose, that has the requested l-galacto configuration and a methyl group at C6, is the highest affinity ligand but other monosaccharides with this configuration such as l-galactose, d-mannose or d-arabinose can still bind to LecB and CV-IIL, albeit with lower affinity [24,29]. The fucose-binding sites of BambL and BC2L-C-nt are again different but they display one common feature. In both cases, an arginine residue is located directly under the fucose ring, establishing bifurcated hydrogen bonds with both the ring oxygen, and the axial O4 hydroxyl group, therefore imposing the specificity for l-galacto configuration.
4 Fine specificities of fucose-binding lectins from lung pathogens
The fine specificity of lectins can be assessed with the use of “glycochips”, i.e. glycan array with more than 500 different human glycans that are available from the “Consortium for Functional Glycomics”. The four fucose-binding lectins of interest described above have been produced recombinantly in Escherichia coli, then chemically labeled with Alexa Fluor and tested on these glycan arrays. The complete results are available from the Consortium web site (http://www.functionalglycomics.org). Fig. 3 summarizes their binding to ABO and Lewis epitopes. The structurally related lectins LecB and CV-IIL both bind to blood group O epitope H-type 2, although LecB also binds to Lewis a. BambL has a broader specificity and binds to blood group A, B, O and to Lewis oligosaccharides. On the opposite, Bc2L-C-nt is highly specific for Lewis b tetrasaccharide.

Selected data for binding of Alexa fluor-labelled lectin to glycan array. Fluorescence intensities have been averaged over the different oligosaccharides that present the indicated epitopes in terminal position in a single copy. Complete glycan array data are available from http://www.functionalglycomics.org.
5 Bacterial strategy for attaining high affinity – a thermodynamic point of view
Titration calorimetry is a method of choice for quantification of protein/carbohydrate interactions. The large number of hydrogen bonds involved results in strongly exothermic interactions that are easily measured by isothermal titration calorimetry (ITC). Furthermore, the range of affinity, with dissociation constants generally between 10−4 to 10−7 M, is well suited for this method. Microcalorimetry experiments allow direct determination of affinity (association or dissociation constant) and stochiometry of binding, and also of the contribution of enthalpy and entropy of binding.
Lectin-carbohydrate interactions are generally characterized by a favorable enthalpy term, due to the large number of hydrogen bonds, that is offset by an unfavorable entropy contribution that has been attributed either to solvent rearrangement or to loss of ligand conformational flexibility [30]. Our work on soluble bacterial lectins has been accompanied by thermodynamic characterization that identified much higher affinity than that observed for plant or animal lectins (Table 2) with dissociation constants lower than micromolar for LecB and BambL for example. The detailed analysis of the binding energy data provides a way to evaluate the role played by the so-called enthalpy-entropy compensation that characterizes the interaction between protein and carbohydrate in solution. The analysis of the soluble bacterial lectins (Table 2 and Fig. 4) shows that only BambL follows these trends with a large favorable enthalpy of binding compensated by large unfavorable entropy of binding. LecB and BC2L-C-nt present slightly favorable entropy of binding. For LecB, this has been attributed to the unique involvement of two calcium ions in the binding site: In the native structure [31], three water molecules are tightly bound to the calcium, and binding of sugar releases them in the bulk of the solvent, resulting in a gain in entropy. This gain is even larger when binding to Lewis trisaccharides since these epitopes are branched oligosaccharides with very limited conformational variations in solution. CV-IIL, that also includes two calcium ions in the binding site, displays the stronger favorable entropy contribution [24]. Bacterial lectins have therefore been demonstrated to present higher affinity to glycan targets than classically observed, and to be able to use unusual entropy-driven strategies for reaching strong binding [32].
Isothermal titration microcalorimetry data for the interaction between lectins of interest and the ligands fucose, α-methyl-fucoside (αMeFuc) and fucosylated oligosaccharides.
Lectin | Ligand | n | Kd (μM) | −ΔG (kJ/mol) | −ΔH (kJ/mol) | TΔS (kJ/mol) | Reference |
LecB | Fuc | 0.96 | 2.9 | 31.5 | 31.2 | 0.3 | [26] |
αMeFuc | 1.05 | 0.38 | 36.6 | 35.5 | 1.1 | [41] | |
Lewis a | 1.08 | 0.21 | 38.1 | 34.9 | 3.1 | [26] | |
Lewis x | 0.95 | 3.4 | 31.1 | 22.3 | 8.8 | [26] | |
CV-IIL | αMeFuc | 0.95 | 1.68 | 32.9 | 21.8 | 11.1 | [24] |
BambL | αMeFuc | 2.02 | 0.96 | 34.3 | 47.8 | –13.5 | [25] |
A-tri | 1.96 | 0.46 | 36.0 | 53.1 | –16.9 | [25] | |
H-type 2 | 1.96 | 7.5 | 29.3 | 44.4 | –15.2 | [25] | |
Lewis Y | 2.00 | 11.1 | 28.3 | 38.6 | –10.3 | [25] | |
BC2L-C-nt | αMeFuc | 1a | 1236 | 14.7 | 20.9 | –6.2 | [27] |
H-type 1 | 0.93 | 77.2 | 23.5 | 23 | 0.5 | [27] | |
Lewis Y | 0.98 | 53.9 | 24.4 | 34.9 | –10.5 | [27] | |
Lewis b | 1.11 | 213 | 21.0 | 19.8 | 1.15 | [27] |
a Value fixed during the fitting procedure.
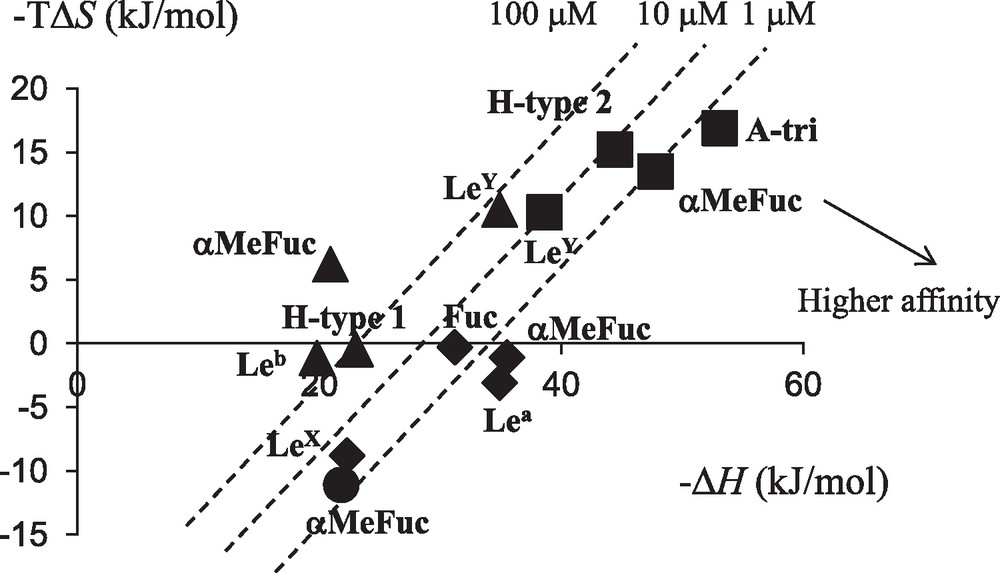
Enthalpy/entropy plot for the interaction of bacterial lectins with fucose or fucosylated oligosaccharides. Code for lectins is as follows: LecB: ; CV-IIL: ; BambL: ; BC2L-C-nt: .
6 Structure-based design of carbohydrate-derived anti-infectious compounds
The LecB lectin from P. aeruginosa is an attractive target for anti-infectious developments since it is involved in biofilm formation [33], a phase of bacterial development that is specially dangerous in lung infection for cystic fibrosis patients. Recent data on related BC2L-A from B. cenocepacia points to possible role in formation of microcolonies by binding to heptose residues present on bacterial cell wall [34]. LecB has also been demonstrated to adhere to human airway cilia in respiratory cell culture and to lower the ciliary beat frequency, an effect that can be inhibited by fucose [35]. P. aeruginosa strain lacking LecB displays reduced cytotoxicity and adhesion on A549 cells compared to parental strain and cause less pronounced lung damages in a murine model of acute pneumonia [36]. In this animal model, co-administration of α-methyl-fucoside markedly reduced lung injury and mortality. Inhalation of a mixture of fucose and galactose resulted in a decrease of the bacterial load in P. aeruginosa pneumonia after treatment of one immune-compromised patient [37], and of a group of cystic fibrosis patients [38].
As a consequence of these promising preliminary data, the search for high affinity ligands for LecB has been initiated by several teams. Since the Lewis a epitope has been identified as the best natural ligand with Kd of 210 nM [26], a first generation of glycomimetics was designed in order to avoid the laborious synthesis of this trisaccharide [39]. The disaccharide αFuc1-4GlcNAc was conserved with addition of several triazole groups on GlcNAc (1 in Fig. 5) and such compounds were as good ligand as Lewis a (Table 3). Comparison of crystal structures of LecB complexed to 1 and to Lewis a (Fig. 6) demonstrates that the αFuc1-4GlcNAc has a slightly different conformation in the two structures. The fucose maintains its coordination with the calcium ions, but the O6 hydroxyl group of the GlcNAc residue establishes different hydrogen bonds. A second generation of compounds containing two such glycomimetics connected through different linkers were then prepared [40]. They were able to bind LecB with the expected stochiometry and compound 2 and 3 proved to be excellent ligands with Kd of 170 and 90 nM, respectively. No additional affinity was obtained for the trimeric compound 4. Attempts to replace the αFuc1-4GlcNAc disaccharide by a fucosyl amide also resulted in high affinity ligands for both monovalent and divalent glycocompounds (4) with an additional gain of simple synthesis and chemical and biological stability [41].
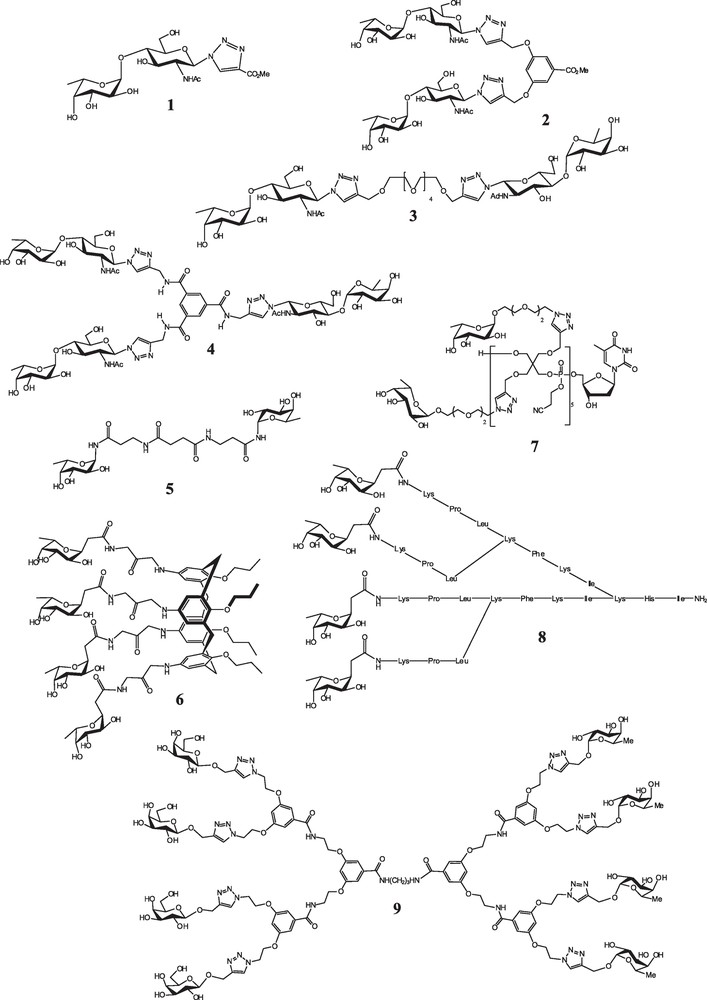
Selection of glycocompounds designed and synthesized for their high affinity towards LecB lectin.
Binding efficiency, competition assays and other activity for a selection of multivalent compounds targeted to LecB. Kd values are obtained by ITC and IC50 values are obtained by competition against binding to polymeric a-fucoside (ELLA test).
Compound | Kd (μM) | IC50 (μM) | Other activity | Reference |
αMeFuc | 0.38 | [41] | ||
Lewis a | 0.21 | 0.60 | [26,39] | |
1 | 0.31 | 0.95 | [39] | |
2 | 0.17 | 0.22 | [40] | |
3 | 0.09 | 0.35 | [40] | |
4 | 0.1 | 0.44 | [40] | |
5 | 0.62 | [41] | ||
6 | Inhibition of 80% biofilm formation at 200 μM | [42] | ||
7 | 0.25 | [43] | ||
8 | 0.14 | Strong biofilm inhibition | [49] | |
9 | Strong precipitation | [51] |
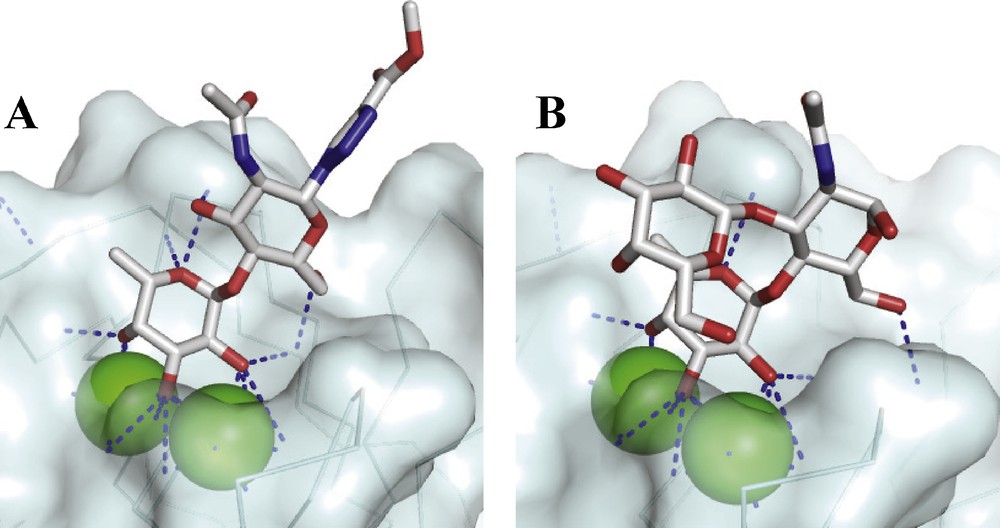
Comparison of binding sites in the crystal structure of LecB complexed with (1) (PDB code 2JDK) [39] and with Lewis a (PDB code 1W8H) [26].
In order to reach higher valency, that is often correlated to higher avidity to lectins, other scaffolds have been utilized. A calix[4]arene platform was used to present four α-l-C-fucosyl units on the same side of the molecule 6 [42]. Glycocompounds based on propargylated pentaerythrityl phosphodiester oligomers were prepared with four to 10 fucose residues (7) [43]. The antenna-like scaffold was preferred by LecB compared to linear or crown-like glycoclusters synthesized by the same team [44]. Glycopeptide ligands were identified by screening combinatorial libraries of peptide dendrimers [45–47] functionalized with N-terminal C-fucoside residues at the end of the branches and some tetravalent compounds such as 8 turned out to be potent ligands for LecB [48–50]. Glycodendrimers were also prepared bearing either eight fucose residues, or both galactosides and fucosides (9) in order to tackle the two lectins from P. aeruginosa [51].
Since the development of therapeutic agents having activity towards bacterial biofilm is of first importance, several of the compounds presented in Fig. 5 have been tested for their ability to inhibit biofilm formation. Compound 6 displayed a dose-dependent biofilm inhibiton effect on P. aeruginosa PAO1 strain [42]. Some of the C-fucosylpeptide dendrimers such as compound 8 not only inhibit biofilm formation but are also able to efficiently disperse established biofilms of wild type and hospital strains of P. aeruginosa [48,49]. This anti-biofilm effect is of a strong importance for further development of therapeutics to control P. aeruginosa infections.
In order to design the next generation of LecB competitors, it is essential to understand the thermodynamic basis of the high affinity obtained with some of the glycomimetics and glycodendrimers described here. The enthalpy-entropy plot displayed in Fig. 7 has been derived from titration microcalorimetry data available for LecB. Some compounds such as bivalent 2 and 3 are clearly highly flexible, resulting in a strong entropy cost in the binding procedure. On the opposite, trivalent compound 4 is sufficiently rigid to avoid the entropy cost but displays a poor enthalpy of binding, indicative of steric limitations. The divalent fucosyl amide compound 5 also displays poor enthalpy of binding, indicating that the NH group at the anomeric position is not as favorable as the anomeric oxygen.
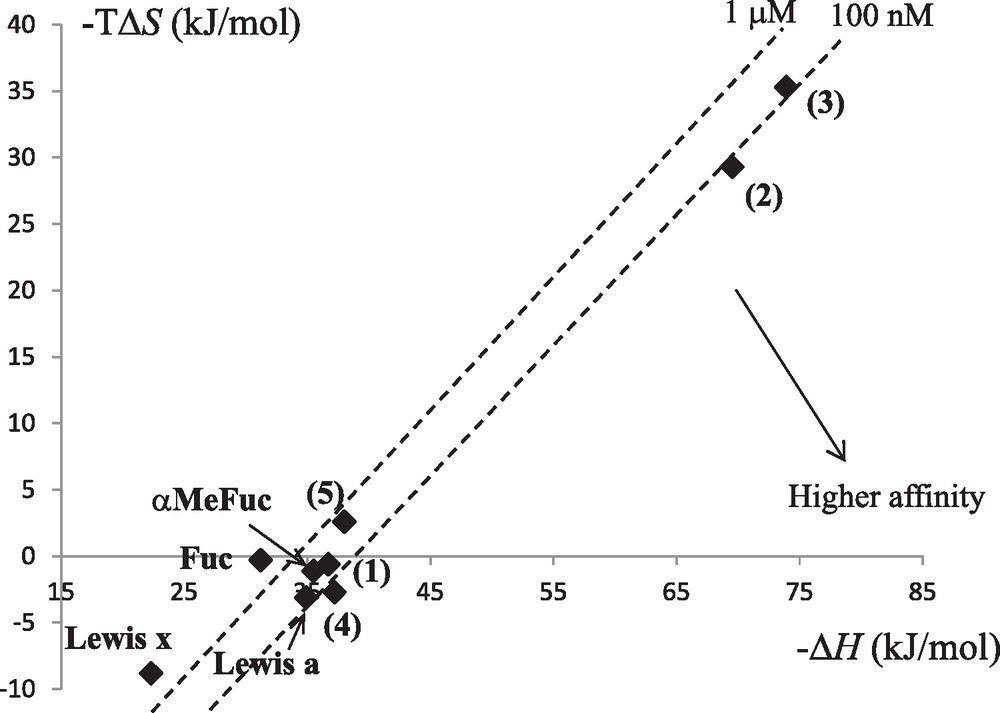
Enthalpy/entropy plot for the interaction of LecB with natural compounds and with high affinity synthetic glycocompounds.
7 Perspectives
The design of glycomimetics and glycodendrimes as anti-adhesive compounds for fighting microbial infection is now a very active field of research [52]. Multivalency is an interesting approach for manipulation of protein-carbohydrate interactions and the “glycoside cluster effect” can induce aggregation of several protein receptors, or chelation of several binding sites on the same protein [53–55]. The galactose-specific lectin LecA from P. aeruginosa received much attention. The close localization of two of the four sugar binding sites of the tetramer allows the design of glycocompounds with very efficient chelate effect. This results in improvement factor of 800 for tetravalent compounds compared to monovalent ones [56,57]. Clearly, the situation is different in LecB that has a ball shape with fucose-binding sites not oriented in a way that would favor chelating effect. Glycomimetics approach with search for aglycone groups that would enhance the affinity of monovalent fucosylated ligand/or even replacement of fucose by non-carbohydrate group should be envisaged.
On the opposite, the fucose-binding lectins from different Bukholderia species present all their carbohydrate-binding sites on the same face of the proteins, i.e. three sites for BC2L-C-nt and six sites for BambL as displayed in Fig. 2. These lectins should therefore be good candidate for inhibition by glycoclusters and glycodendrimers.
Acknowledgments
These studies on bacterial lectins received financial support from CNRS, the Association Vaincre la Mucoviscidose, the GDR Pseudomonas, the COST Actions BM1003 and CM1102 and the Agence Nationale de la Recherche (grants GlycoAsterix 08-PCVI-0028, PA-antiadh 09-JCJC-0047, NeoLect 11-BSV5-002-01 and Labex Arcane ANR-11-LABX-0003).