1 Introduction
The widespread use of synthetic polymers as catalyst supports has started to be looked at as an alternative to typical inorganic carriers. The popularity of employing macromolecular compounds results mainly from their availability and wide range of occurrence in the form of membranes, beads, fibers, films or powders. An unquestionable advantage of these organic supports is the ability to control the cross-linking density, the degree of crystallinity and the pore size. The chemical structure of the polymeric matrix influences the catalytic properties of the metal complex immobilized on it. A properly carried out synthesis of the carrier allows us to control the mechanical properties and thermal stability as well.
A homogeneous catalyst deposited on the support can be easily separated from the reagents and solvents at the same time preserving its specific properties such as activity and selectivity while working under mild pressure and temperature conditions. The procedure of heterogenization of metal complex catalysts on the polymeric carriers generally involves the displacement of ligands attached to the metal and their substitution by groups chemically bonded to the polymer chain [1]. Styrene-divinylbenzene copolymers, equipped with appropriate functional groups, are commonly used as supports for the immobilization of many types of complex catalysts. Their porosity, surface area and solution characteristics can be altered by varying the degree of cross-linking. The main disadvantage of this group of polymers are poor heat transfer ability, insufficient heat resistance and in many cases their poor mechanical properties which prevent them from being used in stirred reactors in which they are pulverized.
Epoxy resins are the main materials used as coating materials, adhesives and composites. Although the polymerization process using the opening of the epoxide ring creates many possibilities for easy functionalization, as well as direct input of metal complexes into the structure of the cured matrix, there have been only few reports on the use of epoxy resins as carriers of metal complex catalysts in organic synthesis. One of the not numerous examples of their use in heterogeneous catalysis are the reports of obtaining molybdenum-doped epoxy resin [2,3]. Such a procedure involves the use of the metal complex as the polymerization initiator and the precursor of the catalytic centres in the cured resin. Epoxy resins doped with Mo, Pd, and Rh complexes manifest high catalytic stability in the processes of epoxidation, hydrogenation and hydroformylation [4]. The use of epoxy resins as carriers of catalysts has been limited so far to the application of various matrices functionalized with epoxy groups for the immobilization of proteins and enzymes to improve their biocatalytic properties [5]. The Sepabeads-EP epoxide carrier used in industry allows for the highly efficient immobilization of proteins via the multipoint covalent bonding with the carrier. This carrier is produced in the process of curing in the presence of expanding agents and provides both suitable properties for the multipoint immobilization and the mechanical characteristics necessary in the usage of the final biocatalyst [6,7]. In 2005, a report was published which describes the obtaining of bi-functional epoxy-thiol carriers, in which the thiol groups are designed to speed up the process of physical adsorption and the epoxy groups are involved in the multipoint bonding of proteins [8].
The increasing usage of ionic liquids in synthesis and catalysis is reflected in a number of monographs dedicated to application of ionic liquids as solvents in many chemical reactions and as substances with catalytic properties [9,10]. Immobilization of organometallic complexes in thin films of heterogenized ionic liquids has been proposed as a new concept combining a high diversity of homogeneous catalysis with an easy separation of heterogeneous catalysts [11]. Ionic liquids fixed onto solid organic or inorganic carriers such as polymers or silica, are heterogeneous catalysts exhibiting the properties of ionic liquids found free in nature. Such a structure of catalytic systems allows for easier separation, recovery, and recycling of the catalysts from reaction mixtures [12].
In the nineties, a new catalyst containing the N-methylimidazole-based chloroaluminate ionic liquid impregnated on silica was patented and it proved to be more active than the free chloroaluminate ionic liquids [13,14]. The research on this catalytic system showed many defects in the structure of the carriers. This resulted in loss of activity during the process due to loss of the active ionic liquid. This disadvantage was removed during the immobilization by introducing the alkyl bridge between the carrier and the ionic liquid [15–17].
In the present study, we describe our approach to prepare the polymer-supported catalysts by immobilizing the palladium complex on the epoxy resin cured with ammonium ionic liquids. These catalysts were evaluated for their performance in the Heck reaction. Our aim was to study the influence of the type of ionic liquid on the reaction activity. Tests of catalysts stability during their prolonged use have also been described. Special emphasis has been stressed on the characterization of metal distribution on the surface of polymer matrix and its leaching during subsequent cycles of the reaction. The X-ray photoelectron spectroscopy (XPS) and IR spectroscopy were used to gain more information about the mode of bonding between the metal atom and the functional groups of the cured epoxy resin.
2 Experimental
2.1 Materials
Epoxy resin bisphenol A epichlorohydrin medium molecular weight ≤ 700 Epidian 5 (EP5), epoxy equivalent 200, (viscosity 20000–30000 mPas at 23 °C), Organika Sarzyna, Poland and epoxy resin a high purity bisphenol A diglycidylether D.E.R.™332 (DER), epoxy equivalent 170, (viscosity 4000–6000 mPas at 25 °C), the Dow Chemical Company, USA, were used. Iodobenzene (Aldrich), triethylamine (Organika Sarzyna), methyl acrylate (Aldrich) and N-metylhylpyrrolidone (ABCR) were used for the Heck reaction. Tetrafluoroboric acid, theophylline, theobromine, caffeine, potassium hydroxide, didecyldimethylammonium chloride as well as all solvents were purchased from commercial suppliers (Sigma-Aldrich, Fluka, Lonza) and used for synthesis of ionic liquids without further purification. The ionic liquids applied are collected in Table 1.
Description of ionic liquids used.
Cation | Anion | Acronym |
[d2m2am][thb] | ||
[d2m2am][thp] | ||
BF4− | [d2m2am][BF4] | |
BF4− | [mthb][BF4] |
2.2 Synthesis of ionic liquids
2.2.1 Synthesis of didecyldimethylammonium and 1-methyltheobromine (caffeine) salts
Method I – Didecyldimethylammonium chloride (0.05 mol) was dissolved in methanol and a stoichiometric amount of saturated methanolic solution of KOH was added. The solutions were stirred at room temperature for 5 min, after which the partially precipitated KCl was filtrated. Then a stoichiometric amount of an appropriate theophylline or theobromine or tetrafluoroboric acid was added. The solutions were stirred again at room temperature for 15 min and after evaporation of the solvent at a temperature of 40 °C, the product was dissolved in 20 ml of anhydrous acetone. The inorganic salt was separated by filtering and acetone was evaporated to give the product, which was finally dried under reduced pressure at 80 °C for 24 h (Scheme 1).
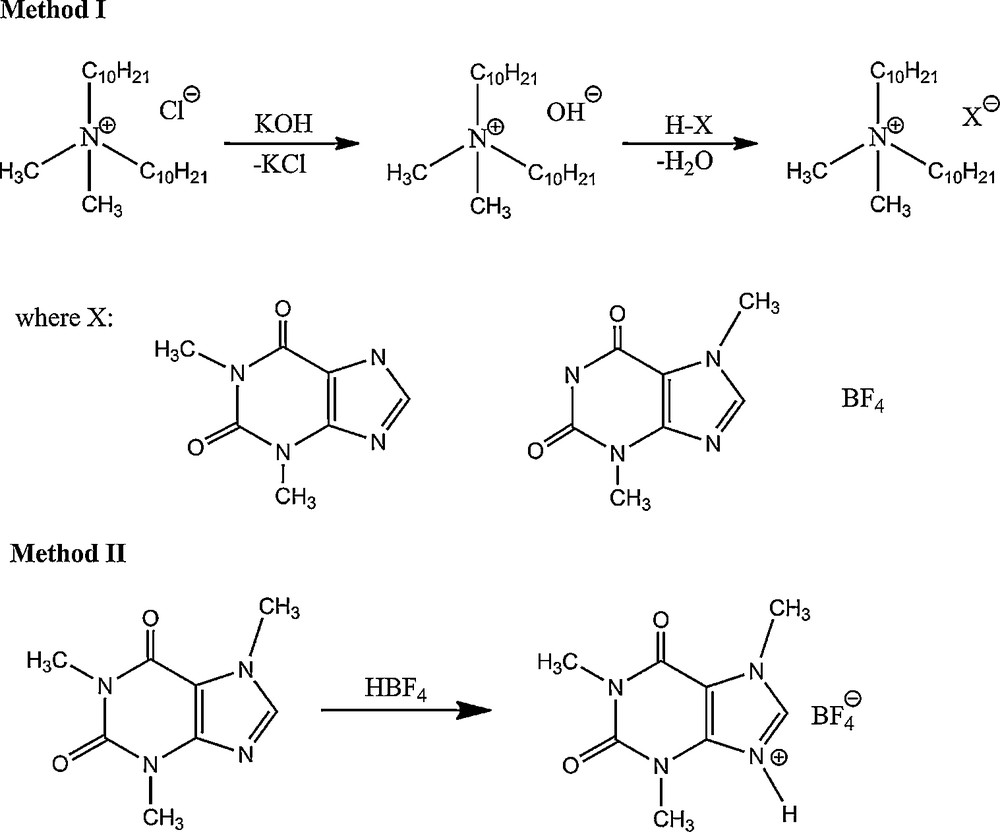
Synthesis of ionic liquids.
Method II – 1-methyltheobromine (caffeine) (0.1 mol) was dissolved in water at 40 °C. Then a stoichiometric amount of an appropriate tetrafluoroboric acid was added. The solutions were stirred at 40 °C for 24 h and after evaporation of the solvent at temperature of 40 °C, the product was finally dried under reduced pressure at 60 °C for 24 h (Scheme 1).
2.2.2 Didecyldimethylammonium theophyllinate [d2m2am][thp]
1H NMR (DMSO-d6) δ (ppm) 0.86 (t, J = 13.6 Hz, 6H), 1.25 (s, 28H), 1.61 (t, J = 11.3 Hz, 4H), 3.02 (s, 6H), 3.19 (s, 4H), 3.38 (s, 6H), 7.13 (s, 1H). 13C NMR (DMSO-d6) δ (ppm) 13.9, 21.7, 22.1, 25.8, 27.3, 28.5, 28.7, 28.9, 29.8, 31.3, 49.9, 62.7, 114.8, 146.3, 149.7, 151.8, 157.4.
Elemental analysis calc. (%) for C29H55N5O2 (505.78): C 68.87, H 10.96, N 13.85. Found: C 68.75, H 10.81, N 13.68.
2.2.3 Didecyldimethylammonium tetrafluoroborate [d2m2am][BF4]
1H NMR (DMSO-d6) δ (ppm) 0.86 (t, J = 7 Hz, 6H) 1.26 (m, 28H), 1.63 (m, 4H), 2.98 (s, 6H), 3.22 (m, 4H); 13C NMR (DMSO-d6) δ (ppm) 13.8, 21.5, 22.0, 25.7, 28.4, 28.6, 28.7, 28.8, 31.2, 49.9, 62.7.
Elemental analysis calc. (%) for C22H48BF4N (413.4): C 63.91, H 11.70, N 3.39. Found: C 64.15, H 12.07, N 3.13.
2.2.4 Didecyldimethylammonium theobrominate [d2m2am][thb]
1H NMR (DMSO-d6) δ (ppm) 0.86 (t, J = 11.6 Hz, 6H), 1.26 (s, 28H), 1.62 (t, J = 11,3 Hz, 4H), 3.01 (s, 6H), 3.18 (s, 4H), 3.35 (s, 3H), 3.85 (s, 3H) 7.9 (s, 1H). 13C NMR (DMSO-d6) δ (ppm) 13.9, 21.66, 22.1, 25.75, 28.48, 28.7, 28.92, 29.15, 31.3, 34.05, 49.9, 62.74, 108.75, 143.11, 149.9, 152.07, 155.4.
Elemental analysis calc. (%) for C29H55N5O2 (505.78): C 68.87, H 10.96, N 13.85. Found: C 68.95, H 10.84, N 13,78.
2.2.5 1-methyltheobromine (caffeine) tetrafluoroborate [mthb][BF4]
1H NMR (DMSO-d6) δ (ppm) 3.39 (s, 3H), 3.57 (s, 3H), 3.98 (s, 3H), 7.58 (s, 1H); 13C NMR δ (ppm) 27.82, 29.63, 33.57, 107.49, 141.42, 148.51, 151.56, 155.28.
Elemental analysis calc. (%) for C8H11BF4N4O2 (282.00): C 34.07, H 3.93, N 19.87. Found: C 34.16, H 4.05, N 19.73.
2.3 Preparation of the carriers
The carrier was prepared by mixing (3 g) the Epidian 5 or D.E.R™322 epoxy resin with (1.2 g) the relevant ionic liquid ([d2m2am][thb], [d2m2am][thp], [d2m2am][BF4], [mthb][BF4]). After obtaining homogenous consistency, the mixture was transferred onto a Teflon® mold and cured at a temperature of 298 to 423 K over a period of 1 hour to 48 hours. The cured resin was then frozen by immersion in liquid nitrogen and mechanically ground to a powder. Average particle size of crushed resins was measured by sieve analysis. These measurements yield a median size ranging from 0.5 to 1.0 mm.
2.4 Catalyst preparation
A typical procedure was followed. To a known amount of polymer matrix (0.1 g) in a round-bottom flask, a known amount of PdCl2(PhCN)2 dissolved in 10 ml of toluene was added. The mixture was stirred at room temperature for 2–3 days when all Pd complex had reacted with the polymer. The yellow product was filtrated off, extracted with toluene under nitrogen in order to remove the complex that was non-chemically bound to the polymer and finally dried under vacuum. For all supported catalysts, the Pd content was fixed below the metal uptake capacity for a polymer. The dry catalysts could be stored in air for many months without change.
2.5 Catalytic tests
The typical Heck reaction was carried out in 50 ml laboratory reactor. The reactor was charged with 0.05 g of supported palladium catalyst, 0.28 ml (2.5 mmol) of iodobenzene, 0.23 ml (2.5 mmol) of methyl acrylate, 0.15 ml (1.4 mmol) of toluene, 0.35 ml (2.5 mmol) of triethylamine and 1.00 ml (10.4 mmol) of N-methylpyrrolidone (NMP). The reaction was conducted at a temperature of 80 °C for 1.5 hours. Samples were taken every 5, 15, 30, 60 and 90 minutes. The composition of the samples was analyzed by GC. The reaction products were quantitatively analyzed by gas chromatography (GC) on a Hewlett-Packard 5990 II gas chromatograph equipped with a thermal conductivity detector. The GC column was HP-50+ (cross-linked 50% Ph Me silicone) 30 m × 0.63 mm × 1.0 μm film thickness. The product was identified by matching retention times with those of authentic samples.
2.6 Catalyst recycling
In the recycling tests, when reaction was completed, the catalyst was filtered off from the reaction mixture, washed with 3 × 25 ml of toluene, vacuum dried and used for the next reaction.
2.7 Spectroscopy characterization
The FT-IR spectra were measured with a BIORAD 175 C spectrophotometer in an air atmosphere. XPS measurements were made on a VG ESCALAB 210 spectrometer with Mg Kα (hν = 1253.6 eV) excitation from an X-ray tube (reduced power 10 kV, 10 mA). The pressure in the spectrometer chamber was about 5 × 10–9 mbar. Samples were pressed to pellets under a pressure of 100 kbar for 10 min before these measurements. The C 1s, N 1s, O 1 s, Cl 2p and Pd 3d core level spectra were recorded. The analyzer pass energy was set at 20 eV. A take-off angle of 90o was used in all XPS studies. Curve fitting was performed using the ECLIPSE data system software. This software describes each of the components of a complex envelope as a Gaussian-Lorentzian sum function. The background was fitted using non-linear model function proportional to the integral of the elastically scattered electrons (Shirley backgrounds). All binding energies (BEs) were referenced to the C 1s neutral carbon peak at 284.6 eV.
2.8 Morphology studies
The morphology of samples was investigated with a scanning electron microscope JEOL 5500 LV, working in high vacuum and accelerating voltage of 10 kV. Energy-dispersive X-ray microscope (SEM–EDX), JEOL JSM 840A, Japan, was used to observe the elemental distribution. The white points in the figures denote Pd atoms.
Pore size distribution parameters were determined by application of the BET method on Sorptomatic 1900 FISONS Instrument.
2.9 Study of Pd leaching
TOF-SIMS measurements were performed using an ION-TOF GmbH instrument (TOF-SIMS IV) equipped with 25 keV pulsed Bi+ primary ion gun in the static mode (primary ion dose about 1.5 × 1011 ions/cm2). The analyzed area corresponds to a square of 500 × 500 μm in case of secondary ion mass spectra collecting and surface imaging. For each sample three spectra from different surface areas were made. To obtain the plain surface of catalysts (then better mass resolution could be achieved), powder samples were tableted before the measurements. In order to compare the quantity of palladium present on the surface of the catalysts before and after reaction, the number of counts of selected ions obtained from collected mass spectra were normalized on the basis of the value of total counts.
3 Results and discussion
3.1 Spectroscopic characteristics of the polymer supports and the immobilized Pd catalysts
Currently, there is a growing number of works on the usage of cured polymers as potential carriers for immobilizing the catalytic complexes of the transition metals groups. This is due to a number of advantages they possess, such as: resistance to most organic solvents, ease of introduction of functional groups without additional functionalization or the possibility of shaping the final product. For the purpose of our research, we decided to select a new type of polymer supports based on epoxy resins with various epoxide numbers, cured with ammonium ionic liquids. We prepared two sets of carriers, one for the Epidian 5 resin, and the other for the D.E.R™322 resin (DER), which were cured with the ionic liquids presented in Table 1.For reasons of clarity and capacity of this article, we decide to focus on the carrier of the Epidian 5 epoxy resin (EP5) cured with didecyldimethylammonium theophyllinate (EP5/[d2m2am][thp]), which serves as an example of spectroscopic characterization.
The FTIR spectrum of the EP5/[d2m2am][thp] carrier (Fig. 1a) reveals a shift of the characteristic peaks observed in spectrum of pure didecyldimethylammonium theophyllinate (not presented here), such as the shift of the absorption bands from 1683 cm−1 to 1705 cm−1 and 1630 cm−1 to 1661 cm−1, corresponding to the stretching vibrations of the CN groups of the purine ring. There was also a new absorption band at 1743 cm−1, corresponding to the carbonyl group resulting presumably from the polymerization of the epoxy resin. IR bands in the region of 1740–1750 cm−1 have been found by Spychaj studying the epoxy resin curing mechanism with imidazolium ionic liquids [18,19]. These IR absorption bands were explained by the proposed mechanism of epoxy resin anionic polymerization initiated by thermal decomposition products of 1,3-dialkylimidazolium ionic liquids. In this mechanism, assuming regeneration of imidazole derivatives, unsaturated structure of epoxy polymer is formed and finally transformed by tautomerization into the structure with the carbonyl group. Our observations indicate similar mechanism of anionic polymerization initiated by the anion derived from the ionic liquid used. The ionic liquid appears to be incorporated into the network, resulting in a cross-linked polymer stabilized with bulky counterions.

FT-IR spectra of: a) EP5/[d2m2am][thp] support; b) EP5/[d2m2am][thp]/Pd catalyst.
By comparing the spectra of the EP5/[d2m2am][thp] carrier and the EP5/[d2m2am][thp]/Pd catalyst (Fig. 1a,b), we can assume that there are two ways of coordinating the metal to the matrix. They are shown in Scheme 2. At 1743 cm−1, the absorption band, corresponding to the vibrations of the carbonyl group of epoxide chain, vanishes from the EP5/[d2m2am][thp]/Pd spectrum (Fig. 1b.), which indicates the bonding of the metal by the O atom with a free pair of electrons (Scheme 2b). We can also observe a decrease and the shift of the absorption bands from 1661 cm−1 to 1656 cm−1 and 1705 cm−1 to 1701 cm−1. This may attest to a particular disorientation of the purine ring under the influence of Pd(II), which indicates the possibility of the metal coordination to the N nitrogen atom (Scheme 2a). No change was observed for strong bands at 1581 cm−1 and 1606 cm−1, considered to be due to asymmetric and symmetric stretching vibrations of two carbonyl groups of theophylline moiety, which indicates that the palladium complex is not bonded to the epoxide support through theophylline carbonyl groups. The new vibrations band corresponding to the coordinate bond palladium-theophylline emerge in the FTIR spectra of the catalyst at 730 cm−1, probably due to the metal-N valence vibrations.

Mode of coordination of Pd(II) complex by EP5/[d2m2am][thb] support.
X-ray photoelectron spectroscopy (XPS) analysis gives a better indication of the chemistry of the surface of the heterogenized catalyst particle, whereas the other analysis methods are more relevant to the composition of the particles as a whole. XPS analyses the outer surface of particulates and enables significant information to be extracted concerning the coordination of a catalytic metal to the polymer support and the mechanism of catalytic reaction. The surface composition of catalyst particles can be determined for species present down to 0.1 atomic percent, shallow from 1.5 to 10 nm, depth profiling. Major functional groups for carbon and oxygen can be identified as well as oxidation states for other species. These characteristics are important sources of information about the interfacial chemistry governing catalytic properties. The percentage data obtained by means of the XPS technique are summarized in Table 2. In the epoxy resin carrier sample cured with the [d2m2am][thp] ionic liquid, the highest percentage has carbon, which is determined by the use of organic compounds. The CO bond is less common than the others and amounts to 6%. A low percentage of nitrogen bonds with carbon and oxygen (nearly 3%) results from the application of the ammonium ionic liquid. As a result of the immobilization of PdCl2(PhCN)2, nearly 5% of PdCl bonds and 1.35% of the divalent palladium bonds emerged. There is also a low percentage (0.37%) of the electron-deficient Pdδ+ bond, which formed due to the lateral overlapping of the d atomic orbitals. This valence state is also referred to Pd clusters [20]. They are not stable under normal conditions, but their prolonged life is related to the protection afforded by the polymer matrix. The lack of distinct changes in composition of catalyst surface after subsequent catalytic cycles indicates permanent binding of the ionic liquids particles.
The surface atomic composition of EP5/[d2m2am][thp] support and EP5/[d2m2am][thp]/Pd catalyst obtained from XPS measurements.
EP5/[d2m2am][thp] | EP5/[d2m2am][thp]/Pd | ||||
Element/band | At, %a | Suggested state | Element/band | At, % | Suggested state |
C1s | 4.16 | CC | C1s | 4.11 | CC |
C1s | 21.03 | CC | C1s | 20.86 | CC |
C1s | 27.51 | CN | C1s | 27.1 | CN |
C1s | 24.5 | COH | C1s | 22.82 | COH |
C1s | 6.05 | CO | C1s | 5.91 | CO |
Cl2p3/2 | 4.95 | PdCl | |||
N1s | 1.62 | N1s | 1.58 | ||
1.11 | NC | N1s | 1.03 | NC | |
Pd3d5/2 | 0.37 | Pdδ+ | |||
Pd3d5/2 | 1.35 | Pd (II) | |||
O1s | 1.98 | O1s | 1.95 | ||
O1s | 5.58 | CO | O1s | 5.86 | CO |
O1s | 6.23 | CO | O1s | 6.10 | CO |
O1s | 4.98 | OH | O1s | 4.80 | OH |
a The percentage of the element in the support/catalyst.
The XPS data summarized in Table 3 show that three possible coordination sites participate in binding the Pd to the epoxide support. This is indicated by the increase of the binding energy BE for purine nitrogen atoms and OCO 1s electrons after the Pd attachment. These data are consistent with the results of FTIR measurements. The XPS spectrum of the freshly prepared EP5/[d2m2am][thp]/Pd catalyst shows that palladium appears dominantly in the form of Pd(II). Minor fractions of Pdδ+ nanoclusters are also observed. It indicates that this polymeric support chains might stabilize such a catalytically active monovalent metastable species with a binding energy larger than that of the Pd metal. The recovered EP5/[d2m2am][thp]/Pd catalyst after a Heck reaction contained also some minor fraction of Pd(0). Since the epoxide matrix cured with [d2m2am][thp] ionic liquid possesses coordination sites of different coordination ability, it seems very likely that the zero-valent palladium, Pd(0), comes from the reduction of Pd(II) coordinated to the carbonyl oxygen, the weaker of the three coordination sites, whereas new species formed after each reaction run, Pd clusters, come from the reduction of Pd(II) coordinated to the purine nitrogen atoms. The binding energy of Cl 2p3/2 in the XPS spectra, in the region of 201.7–201.9 eV, indicates the presence of a –PdCl2 structure.
Binding energy values from XPS measurements.
Sample | Binding energy (eV) | ||||||
N 1s | O 1s | Pd 3d5/2 | Cl 2p3/2 | ||||
N | Pdδ+ | Pd(0) | Pd(II) | ||||
EP5/[d2m2am][thp] | 403.4 | 401.3 | 532.0 | – | – | – | – |
EP5/[d2m2am][thp]/Pd before use | 406.3 | 403.7 | 536.1 | 339.7 | – | 341.5 | 201.9 |
EP5/[d2m2am][thp]/Pd after 3 runs | 406.2 | 403.5 | 536.3 | 339.5 | 335.9 | 341.3 | 201.7 |
3.2 Morphology analysis
The characterization of palladium distribution on the surface of the catalysts and the distribution of the size of the nanoparticles were performed by means of a scanning electron microscope (SEM) and energy-dispersive X-ray spectroscopy (EDX) (Figs. 2–5). The average size of the palladium nanoparticles for each sample was determined by counting the size of about 100 particles from several SEM pictures. The DER/[d2m2am][thp]/Pd X-ray microprobe (Figs. 2 and 3) indicates a uniform distribution of the palladium particles with an average size of 0.1 nm. A less regular distribution is observed for the DER/[d2m2am][thb]/Pd catalyst with an average particle size of 0.6 nm (Figs. 4 and 5). These differences may be explained by referring to the morphology of the polymeric carrier and the steric restrictions imposed by the different microporosities of these supports. The support microporous structure can influence the activity of catalyst. The steric demands of a catalytic centre bound to a support can be very different from those of its homogeneous analogue. The porous structure of the support affects the accessibility of the interior of the swollen resin by increasing diffusional restrictions for reaction species. The results determined by the nitrogen BET adsorption method indicate that DER and EP5-based supports have different pore size distribution which probably arise from differences in crosslink densities. These materials show different ratio of micropores and very low volume of meso- and macropores. The EP5 resins had pore size distribution with the majority of the micropores of radii below 2 nm. The DER resins exhibit the lower value of pore specific volume, the lower surface area and porosity than EP5-based supports.

SEM micrograph and EDX Pd-mapping of the surface of the DER/[d2m2am][thp]/Pd catalyst before use.

SEM micrograph of the surface of the DER/[d2m2am][thp]/Pd catalyst before use.
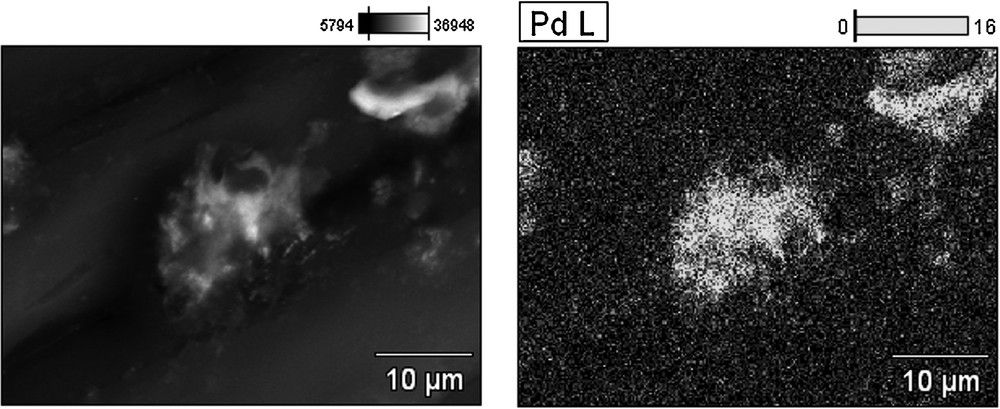
SEM micrograph and EDX Pd-mapping of the surface of the DER/[d2m2am][thb]/Pd catalyst before use.
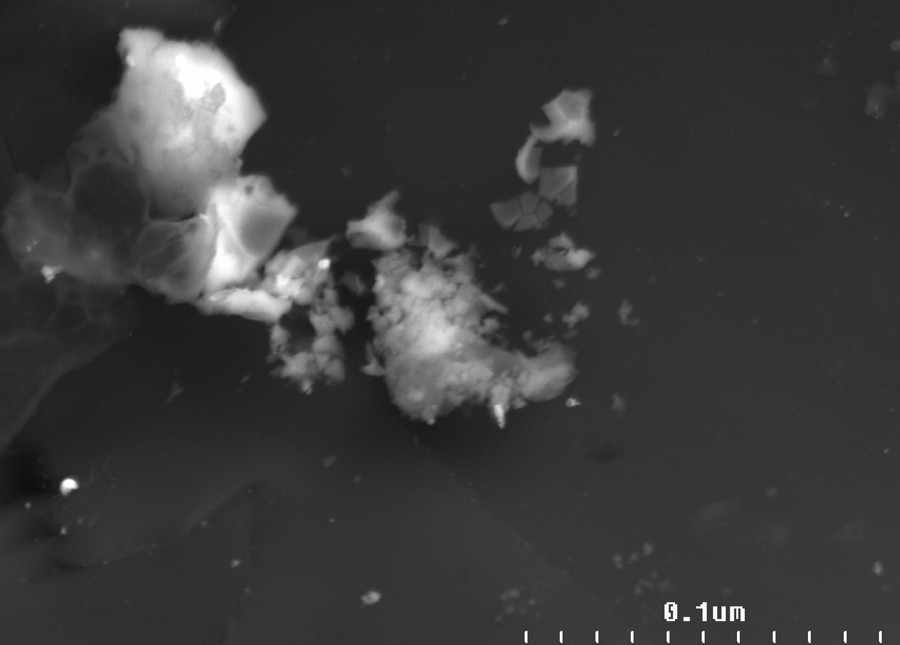
SEM micrograph of the surface of the DER/[d2m2am][thb]/Pd catalyst before use.
3.3 Catalytic tests
The activity and stability of the palladium catalysts were researched during their multiple usage in the model Heck reaction (Scheme 3). The reaction entailed coupling iodobenzene with methyl acrylate, occurring in the solution of N-methylpyrrolidone (NMP) in the presence of triethylamine. The only observed product was methyl trans-cinnamate. Results in Table 4 show that for the samples of the EP5/[d2m2am][thb]/Pd and EP5/[d2m2am][thp]/Pd catalysts, where in both cases the organic anion is present in the used ionic liquid, a significant increase of activity can be seen in the second cycle of the reaction. This is probably caused by the increasing volume of the sample (swelling), hence allowing for an easier access to the active spots in the open pores of the catalyst. In the case of the catalysts based on EP5/[d2m2am][BF4] and EP5/[mthb][BF4] carriers which have an inorganic anion in their structure, a high activity in all reaction runs is observed. We ascribe the lower catalytic activity of the catalysts supported on the DER resins to fewer Pd sites being accessible to the reactants when these are attached to the polymer of lower microporosity. The better availability of the catalytic sites situated in a EP5 resin having a greater volume of micropores and higher surface area increases the activity of supported Pd systems.

The Heck reaction of iodobenzene with methyl acrylate.
The Heck reaction of iodobenzene with methyl acrylate over epoxide-supported palladium catalysts.
Catalyst | Run | Conversiona [%] |
EP5/[d2m2am][BF4]/Pd | 1 | 88 |
2 | 91 | |
3 | 98 | |
EP5/[d2m2am][thb]/Pd | 1 | 95 |
2 | 98 | |
3 | 83 | |
EP5/[d2m2am][thp]/Pd | 1 | 56 |
2 | 34 | |
3 | 29 | |
EP5/[mthb][BF4]/Pd | 1 | 89 |
2 | 92 | |
3 | 91 | |
DER/[d2m2am][thb]/Pd | 1 | 92 |
2 | 79 | |
3 | 51 | |
DER/[d2m2am][thp]/Pd | 1 | 56 |
2 | 38 | |
3 | 27 | |
DER/[mthb][BF4]/Pd | 1 | 12 |
2 | 86 | |
3 | 92 |
a Conversion of iodobenzene after 1.5 h.
The activities of most tested catalyst decrease with the subsequent usage probably due to the leaching of the palladium. We applied time-of-flight secondary ion mass spectrometry (TOF-SIMS) to investigate the deactivation process and to observe a deterioration of the Pd distribution on the surface of catalysts after a third reaction run in comparison with the fresh sample. This technique permits the evaluation of the chemical composition of the catalyst surface and the distribution of metal or other catalyst components on the surface. However, the quantitative analysis by TOF-SIMS method is relatively difficult. TOF-SIMS may be very useful in the studies of catalyst deactivation processes. In such a case changes in the composition of the upper layer of the investigated surface can be determined. Owing to TOF-SIMS, a considerable drop in the amount of surface accessible Pd was observed for EP5/[d2m2am][BF4]/Pd and EP5/[d2m2am][thb]/Pd catalysts after the Heck reaction in comparison with the fresh samples (Table 5). The drop of Pd content was at safe level and slightly affects the catalysts activity within acceptable limits, and can be explained by the often reported enhancement of the activity for a catalyst containing mixed valence states of Pd(II) and Pd(0) [21]. Some metal leaching is often observed when supported catalysts are employed, usually higher in the first two runs and lower in the next ones. It mostly comes from the detachment of the weakly bound metal species via, for example, physical forces. These are still present after washing the catalyst during the preparation procedure. Observed decrease of activity during prolonged use can be also connected with poor chemical resistance of epoxy supports to polar solvents like NMP. However, this solvent was used for the model Heck reaction, which we chose only to preliminary characterization of catalytic properties of these catalysts. The performed catalysts showed good activity and stability comparing to other catalysts. For example, Cypryk et al. reported that the activity of the polysiloxane-supported Pd catalysts in the Mizoroki–Heck arylations of methyl acrylate, butyl acrylate, and acrylonitrile by iodobenzene and of styrene by bromobenzene was comparable to that of homogeneous PdCl2(PhCN)2 and maintained through five to ten reaction cycles [22]. Sudalai reported the use of Pd-exchanged montmorillonite clays in the Heck reaction. Investigated catalysts were reused three times without significant loss of activity [23].
Normalized intensity of 106Pd+ selected from the mass spectra of palladium catalysts.
Catalyst | Run | Intensity of 106Pd+ |
EP5/[d2m2am][BF4]/Pd | 0 | 8.2 × 10−3 |
3 | 4.4 × 10−3 | |
EP5/[d2m2am][thb]/Pd | 0 | 3.3 × 10−3 |
3 | 2.3 × 10−3 |
The ionic liquid used at the curing stage has a decisive influence on the catalytic activity of the catalysts. This was also confirmed by the results of TOF-SIMS measurements of the EP5/[d2m2am][BF4]/Pd and EP5/[d2m2am][thb]/Pd samples (Table 5). The disparity of the intensities of the palladium ions for the EP5/[d2m2am][BF4]/Pd and EP5/[d2m2am][thb]/Pd catalysts just after the immobilization shows that more palladium complex is deposited on the carrier with an inorganic anion in the structure of the ionic liquid used as hardener.
To determine the nature of the active species, the effect of catalyst poisoning has also been investigated. To rule out the possibility of catalysis by microparticles (colloids or nanoclusters) of zero-valent palladium formed from leached and reduced Pd(II) precursor, a mercury poisoning experiment was carried out by adding mercury to the reaction mixture before the catalytic test. The mercury poisoning of metal(0) particles, by amalgamating the metal or adsorbing on the metal surface is a widely used test for the heterogeneity/homogeneity of catalysis [24]. An epoxide-supported palladium catalyst vigorously stirred with a large excess of metallic mercury for 24 h prior reaction shows almost the same catalytic activity for the Heck reaction as before poisoning. It is known that trace amounts of leached metal particles or soluble palladium complex can be very catalytically active [25]. As additional evidence for the identity of the true catalyst, hot filtration technique has also been employed, and no catalytic activity was observed, confirming the absence of active species (soluble Pd(0)) in the hot filtrate.
4 Conclusions
It was shown that epoxy resins cured with ionic liquids are very useful and versatile polymeric supports for the preparation of heterogenized palladium catalysts. The mechanism of curing the resin and the manner of metal coordination to the carrier were determined. The different coordination sites in the polymer promote the formation of active catalytic species as finely dispersed metal particles with mixed valence states. The type of ionic liquid used to cure epoxy resin can greatly affect the catalytic properties of the epoxide-supported palladium catalyst. The studied catalysts showed different activity in the Heck reaction, which was related to the different structure of the ionic liquid used. Based on the recycling efficiency tests, it was found that prepared polymer-supported palladium catalysts have good catalytic stability. The usage of ionic liquids with a different structure of ions as curing agents enables us to obtain carriers which do not require additional functionalization to control the ligand-binding affinity to metal complexes.
Acknowledgement
This work was supported by the National Science Centre (Poland) (grant No 0255/B/H03/2011/40) and by the Faculty of Chemistry, Lodz University of Technology, Young Scientist Fund (grant 28.05.2012).