1 Introduction
Designs of reaction systems for the oxidation of organic compounds provide paths to synthesize a wide range of functionalized molecules. Selective oxidation of hydrocarbons to give oxygenic compounds is a fundamental reaction in organic chemistry both for elemental research and industrial manufacturing [1]. Investigation related to the new solid oxidation catalysts is one of the most significant current topics, connected with both industrial and fundamental researches, because the traditional techniques involve the use of large amounts of highly toxic oxidation state of Co, Os, Mn, and Co reagents. Lower oxidation state metals such as Co(II), Mn(II) and Cu(II) in AcOH may be utilized with O2 as the eco-friendly oxidant. However, the conditions in many cases are unpleasant, the reagent mixture is corrosive, and the chemistry is seldom selective [2]. In fact, as the traditional metal compounds used in this process are in catalytic amounts, the reaction conditions are harsh; the product selectivity is low and often corrosive promoters like bromide anions are used along with the traditional catalyst. However, the separation of the catalyst from the reaction mixture is very difficult, the catalyst cannot be reused, and also a lot of tarry waste is produced. Environmentally acceptable catalytic oxidations that carried out under mild conditions in the liquid phase with high selectivity are obviously desirable. Transition metal complexes can be used as excellent catalysts for oxidation reactions with a suitable organic ligand [3,4]. The oxidation reactions catalyzed by metal complexes are often prevented due to oxidative degradation of the complex and/or the formation of μ-oxo dimmers [5]. Several methods can be employed to diminish the above-mentioned problems, such as separation of the catalyst from the reaction medium and increase of the effectiveness of the catalysts by improving active sites’ life. Always, transition metal catalysts have been immobilized on solid supports, such as organic polymers, inorganic solids (SiO2, clay, zeolite…), colloids, molecular sieves, ion-exchange resins and polymer membrance [6–15]. The target of heterogenization of homogeneous catalysts provides active heterogeneous catalysts, which possess the same catalytic site as in their homogeneous application offered by homogeneous catalysts and become thermally more stable with the ease of separation and recycling of heterogeneous catalysts [16–20,12]. The special advantages of immobilized catalysts have prompted several groups to introduce various types of solid supports [6–20]. Among the various inorganic supports, silica is the most common. A review article by Jacobs et al. provides information about of such immobilized complexes [21]. The most studies has focused on the modified silica gel, as the supports in high performance liquid chromatography (HPLC), inorganic compound separations, ion-exchange, preconcentration of inorganic compounds and pesticides preconcentration, as well as being chemical sensors and finding use in catalysis [22–27]. The present paper now reports a novel heterogeneous catalyst for the liquid phase partial oxidation of alkyl aromatic and alkene substrates based on a chemically modified nano-sized SiO2/Al2O3 mixed oxides which significantly enhanced catalytic activity and selectivity. The synthesis of the catalyst is fully described together with the results obtained in the catalytic oxidation of ethylbenzene, cyclohexene and benzyl alcohol (used as a model substrate) under solventless conditions and TBHP as the consumable source of oxygen.
2 Experimental
2.1 Materials and instruments
All reagents were purchased from the Merck and Fluka chemical companies. Reagents were used without extra purification, but solvents were purified with standard methods. Inductively coupled plasma (ICP) measurements for Co content evaluation were performed using a Perkin-Elmer ICP/6500. Infrared data were collected on KBr pallets using a JASCO FT/IR (680 plus) spectrometer and the position of an infrared band is given in reciprocal centimeters (cm−1). Diffuse reflectance spectra were registered on a JASCO-550 UV-Vis spectrophotometer that was equipped with a diffuse reflectance attachment in which BaSO4 was as the reference. Type and quantity of the resulting products from oxidation were determined by a HP 6890/5973 GC/MS instrument and analyzed by a Shimadzu GC-16A gas chromatograph (GL-16A gas chromatograph with a 5 m × 3 mm OV-17 column, 60–220 °C (10 °C/min), Inj. 230 °C, Det. 240 °C). For elemental analysis a CHN-Rapid Heraeus elemental analyzer (Wellesley MA) was used. Before carrying out the nitrogen (99.999%) adsorption experiments, the sample was outgassed at 393 K for 14 h, then the experiment has been carried out at 76 K using a volumetric apparatus (Quantachrome NOVA automated gas sorption analyzer). The specific surface areas were calculated using the BET (Stephen Brunauer, Paul Hugh Emmett, and Edward Teller) method. The images of scanning electron micrograph (SEM) and transmission electron microscopy (TEM) were taken using a Philips 501 microscope and a Tecnai F30TEM operating at 300 kV, respectively. In addition, energy dispersive X-ray analysis was conducted on each sample. Size distribution in order to count nanoparticles in reverse microemulsion was measured by a Zetasizer Nano-ZS-90 apparatus (ZEN 3600, MALVERN instruments).
2.2 Preparation of Organometallic-SiO2/Al2O3 nano-sized
Nano-sized SiO2/Al2O3 was used as the support prepared by the sol–gel method [26]. The solid SiO2/Al2O3which produced, was being used as the support in the synthesis of the nano-catalyst. At first, 3.5 g of nano-sized SiO2/Al2O3 was activated at 500 °C for 5 h under air and then refluxed with 4.3 mL of trimethoxysilylpropylamine (3-APTMS) in dry toluene (50 mL) for 24 h. The solid achieved during this process was filtered and washed off with dry methanol at 100 °C under vacuum for 5 h. Then bipyridylketone (BPK) was added to a suspended solution of SiO2/Al2O3-APTMS in dry methanol. To synthesize the SiO2/Al2O3-APTMS-BPK-Co (Scheme 1), 2.0 g of SiO2/Al2O3-APTMS-BPK was suspended in 50 ml of ethanol at a round bottom flask followed by adding of 3.0 mmol Co(OAc)2.4H2O. The mixture was refluxed during 24 h under magnetical stirring.
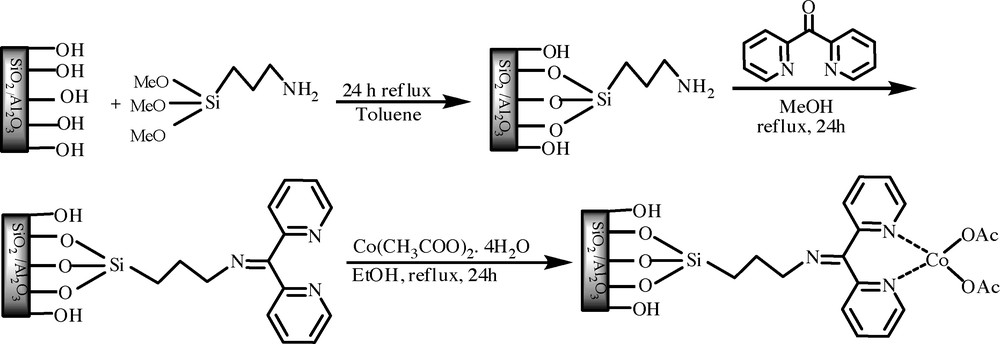
Schematic representation of preparation process for Si/Al-APTMS-BPK-C.
2.3 General procedure for oxidation of ethylbenzene, cyclohexene and benzyl alcohol
In this procedure, the heterogeneous catalyst (5.0 mg), the substrate (9.0 mmol) and an oxidant (9.0 mmol, 80% TBHP) were added in a three-necked round bottom flask equipped with a refluxed condenser. The mixture was stirred at the desired temperature. After filtering and washing with solvent, the filtrate was monitored by GC. The products were identified by GC–MS techniques. The conversion and selectivity were calculated with GC area normalization. Finally, comparative experiments were performed under different conditions.
3 Results and discussion
3.1 Characterization
Using BPK and Co(OAc)2.4H2O through the covalently immobilization, caused heterogeneous Co catalyst being synthesized onto the SiO2/Al2O3 mixed oxides, as illustrated in Scheme 1. The formation of Co catalyst onto the SiO2/Al2O3 was verified using CHN, FT-IR, UV–Vis, and SEM. The surface area, pore size and volume of the modified support were significantly reduced compared to the pristine SiO2/Al2O3 (this decrease indicates the decline in interaction between adsorbate, N2 molecules, and the nano-sized SiO2/Al2O3 surface after modification with organic chains). The loading of cobalt in the heterogeneous cobalt catalyst was characterized by elemental analyses. The final metal content was around 0.193 mmolg−1, indicating that 58.7% of the immobilized ligands were complexed with cobalt ions (Table 1).
Chemical composition and physicochemical properties of the immobilized Co-nano-catalyst on the SiO2/Al2O3 mixed oxide. Co(OAc)2.4H2O.
Sample | Elemental analysis (wt%)b | Organic functional group (mmol/g mixed odide)c | Immobilized Co-Schiff base-complex (mmol/g mixed oxide)d | %Coordinated Schiff base groups to Co ions | Structural parameterse | ||||
C | N | Co | Surface area (m2/g) | Pore volume (cm3/g) | Pore diameter (A°) | ||||
SiO2/Al2O3 nanosizeda | – | – | – | – | – | – | 498 | 0.045 | 36 |
Si/Al-APTMS | 8.13 | 3.75 | – | 2.67 | – | – | 378 | 0.031 | 25 |
Si/Al-APTMS-BPK | 10.9 | 4.92 | – | 3.51 | – | – | 320 | 0.026 | 20 |
Si/Al-APTMS-BPK-Co | 7.45 | 1.38 | 1.14 | 0.98 | 0.193 | 58.7 | 281 | 0.021 | 18 |
a Molar ratio of Si/Al was 60:40, determined from EDX analysis.
b Nitrogen was estimated from the elemental analyses. Co content determined from EDX analysis.
c Determined from the N-contents.
d Determined from the Co-content, assume that cobalt ions coordinated with Schiff base ligands.
e The pore size calculated using the BJH method.
3.1.1 The FT-IR spectra
In Fig. 1, the FT-IR spectra of SiO2/Al2O3-APTMS, SiO2/Al2O3-APTMS-BPK, and SiO2/Al2O3-APTMS-BPK-Co are shown. The strong absorption bands related to Si–O–Si stretching vibrations were observed in the spectrum of SiO2/Al2O3 at 1010–1290 and 798 cm−1. The FT-IR spectrum of SiO2/Al2O3-APTMS shows several signals originating from amino propyl groups, which are related to CH stretching modes of the propyl group, appeared in the area of 1450–1560 cm−1 and 2935–2860 cm−1. The results show that the SiO2/Al2O3 was successfully modified by amine spacer groups. However, the NH deformation peak at 1540–1560 cm−1 confirms the successful functionalization of the SiO2/Al2O3 mixed oxide with 3-APTMS. In Fig. 1B, the CN imine vibration signal was observed at 1630 cm−1 [21], which confirms the condensation reaction between BPK and organo-functionalised SiO2/Al2O3. The peaks in the ranges of 3066–3020 and 1437–1434 cm−1 are attributed to the CH and CC stretching vibrations of pyridine groups, respectively [21,26]. After interacting of cobalt ions with immobilized BPK over modified SiO2/Al2O3, the weak absorption peak at 566 cm−1 appeared; it is attributed to the Co-N bands.
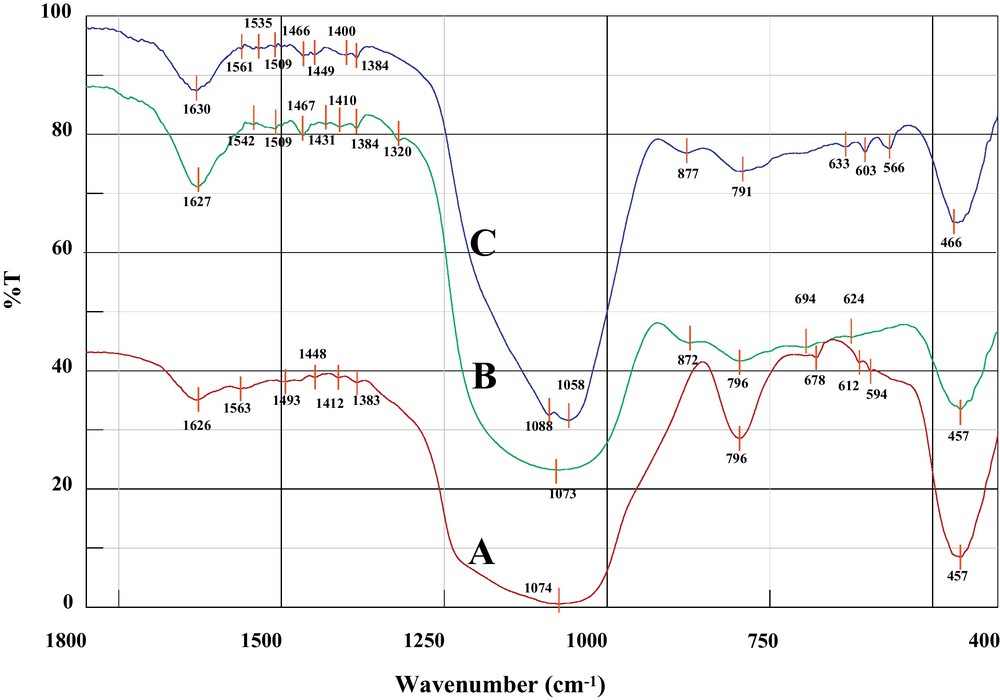
FTIR spectra of: (A) SiO2/Al2O3-APTMS; (B) SiO2/Al2O3-APTMS-BPK; (C) SiO2/Al2O3-APTMS-BPKCo.
3.1.2 The UV-vis spectra
By comparing the UV–Vis spectrum of the SiO2/Al2O3 and the Si/Al-APTMS-BPK (Fig. 2), it was observed that the spectrum of the SiO2/Al2O3 showed an absorption band near 249 nm but, for the SiO2/Al2O3-APTMS-BPK transitions π→π* and n→π* of the ligands caused strong adsorption at the 255–320 nm [28]. A characteristic feature of immobilized Co(II) species after the reaction of SiO2/Al2O3-APTMS-BPK with Co(OAc)2·4H2O was observed through a change of the color of powder from yellow to deep brown. Consequently, UV-visible spectra indicated the appearance of several new metal d–d transition bands at around 570 nm upon cobalt immobilization.
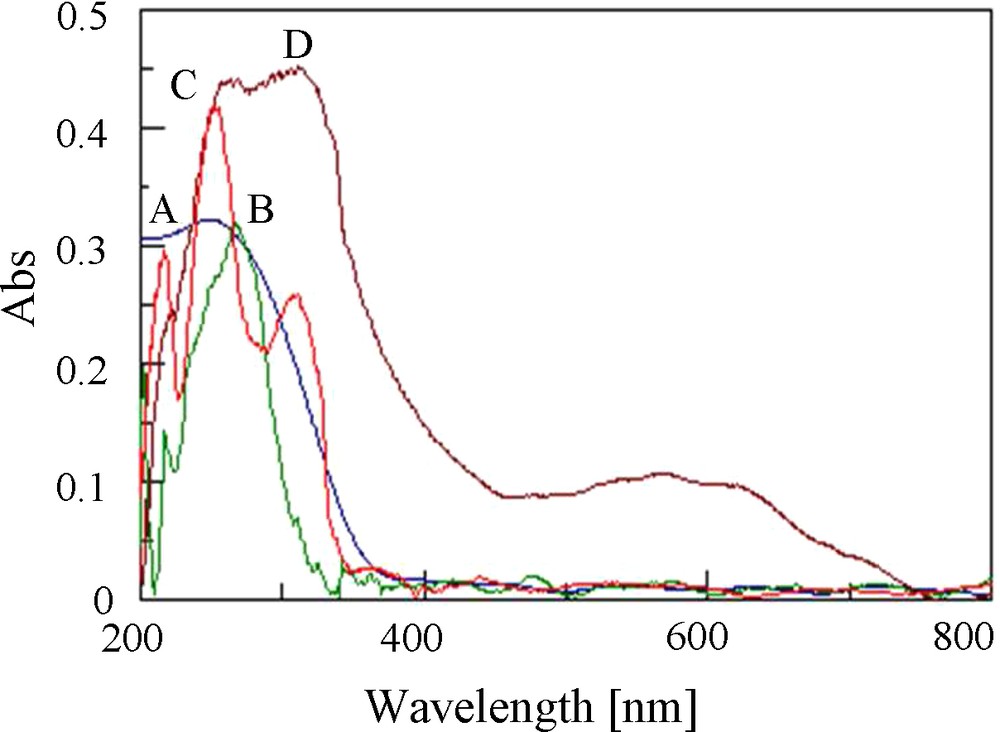
DR UV–vis spectra of: (A) SiO2/Al2O3; (B) SiO2/Al2O3-APTMS; (C) SiO2/Al2O3-APTMS-BPK; (D) SiO2/Al2O3-APTMS-BPK-Co.
3.1.3 Scanning electron microscope
Scanning electron microscope (SEM) images of the nano Co catalyst is shown in Fig. 3. It could be seen that the nanoparticles appearance and size of them were similar, demonstrating that the nanoparticles of SiO2-Al2O3 have good mechanical stability and they have not been destroyed during the whole modifications. The particle size distributions obtained for the reverse micro emulsion solution was around 28 nm.
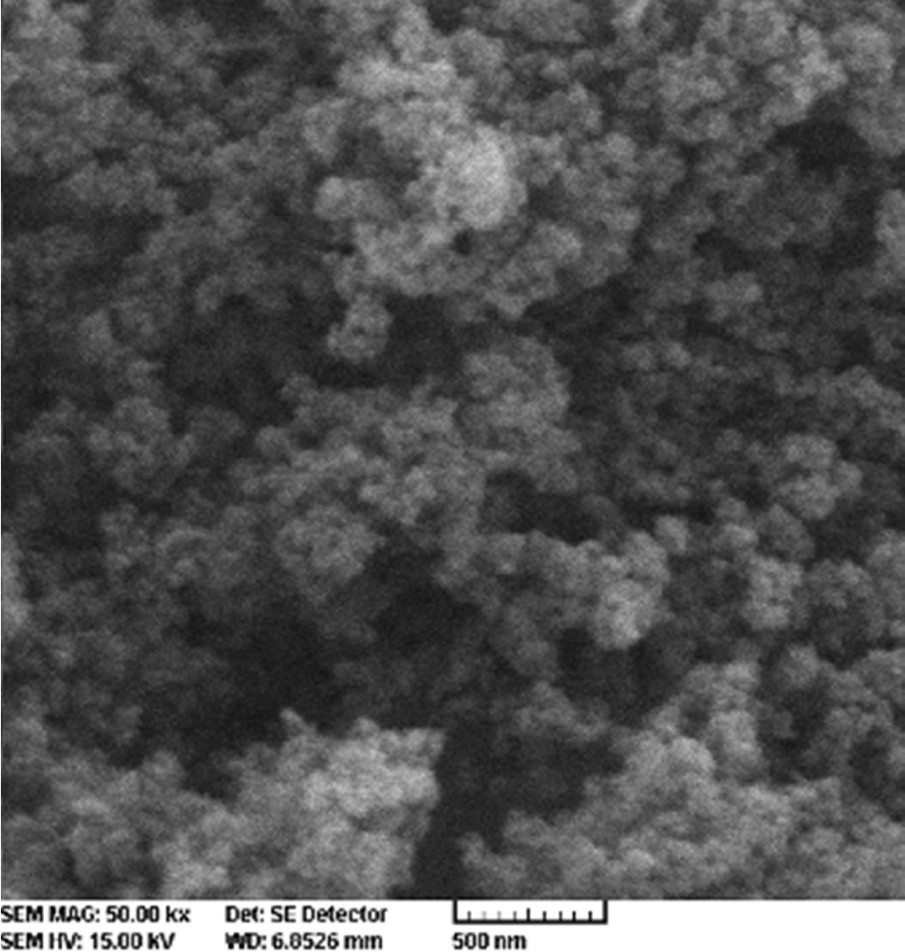
SEM of SiO2/Al2O3-APTMS-BPK-Co nano-catalyst.
3.2 Oxidation of ethylbenzene
To optimize the effectiveness of reaction conditions on the oxidation of ethylbenzene (EB) by TBHP in the presence of the nano-cobalt catalyst, various parameters, such as the amount of the substrate to oxidant, the temperature, and the nature of the solvent, on the performance of the heterogeneous catalyst were investigated. In this reaction, major products are acetophenone (1) benzaldehyde (2) and benzoic acid (3) (Scheme 2).

Oxidation of ethylbenzene catalyzed by SiO2/Al2O3-APTMS-BPK-Co in the presence of tert-butyl hydroproxide.
The GC analysis did not show any oxidation products of the aromatic ring in effluents. In order to study the effects of solvents on the oxidation of EB, various solvents such as acetonitrile, ethanol, water, benzene and dichloromethane at 100 °C were experimented (Table 2). The reaction in the presence of coordinating solvents such as ethanol had low conversion, while using water as a solvent made no reaction. It seems that the donor electrons of these solvents had more ability to occupy the vacant space around the existing metal in catalyst, so this prevents coordinating from oxidant molecules [29]. The major product in the oxidation of EB in all solvents except ethanol and dichloromethane was acetophenone. The percent of EB conversion decreased in the following order with different solvents; 41.0% (dichloromethane) > 32.0% (benzene) > 26.0% (acetonitrile) > 16.0% (ethanol) > 0.0% (water). The use of protic solvents and chlorinated solvent favor the selectivity to benzaldehyde and benzoic acid, while aprotic solvents lead to acetophenone [30]. The acetophenone selectivity on different solvents decreased in the following order; benzene (55.0%) > acetonitrile (46.0%) > dichloromethane (36.0%) > ethanol (24.0%) > water (0.0%) (Table 2). Thus, dipole moments of the solvent probably play an essential role in the oxidation of EB. More investigations showed that the reaction under solventless conditions had higher selectivity and more catalytic activity than in the presence of a solvent (as protic and aprotic ones) (Table 2). This observation more likely demonstrates that the EB and solvent molecules are competitors for the active sites of metal center in catalyst.
Effect of solvents on ethylbenzene oxidation.
Solvent | Ethylbenzene conversion (wt%) | Product selectivity (%) | ||
Acetophenone | Benzaldehyde | Benzoic acid | ||
Without | 47.2 | 79.0 | 8.83 | 12.17 |
Acetonitrile | 32.7 | 46.2 | 39.6 | 15.2 |
Ethanol | 16.8 | 24.0 | 63.7 | 13.2 |
Benzene | 26.2 | 55.8 | 27.5 | 18.7 |
Water | 0.0 | 0.0 | 0.0 | 0.0 |
Dichloromethane | 41.4 | 36.9 | 48.2 | 16.7 |
In order to evaluate the effect of substrate to oxidant molar ratio on the catalytic activity and selectivity in solventless condition, the reactions were carried out at different molar ratios of EB:TBHP (1:1, 1:3 and 1:5) at 100 °C after 24 h (Fig. 4). It was found that the different molar ratio of EB:TBHP has strong effects on catalytic activity and selectivity. In most of the experiments acetophenone was the major product and catalytic activity does rise with increasing concentration of TBHP to EB, which is due to the presence of excess tert-butyl hydroperoxide coordinated with active sites of the nano-catalyst (heterogeneous Co catalyst) (Fig. 4). In all EB oxidation reaction conditions, as the reaction time increased, the conversion and selectivity to acetophenone increased as well.
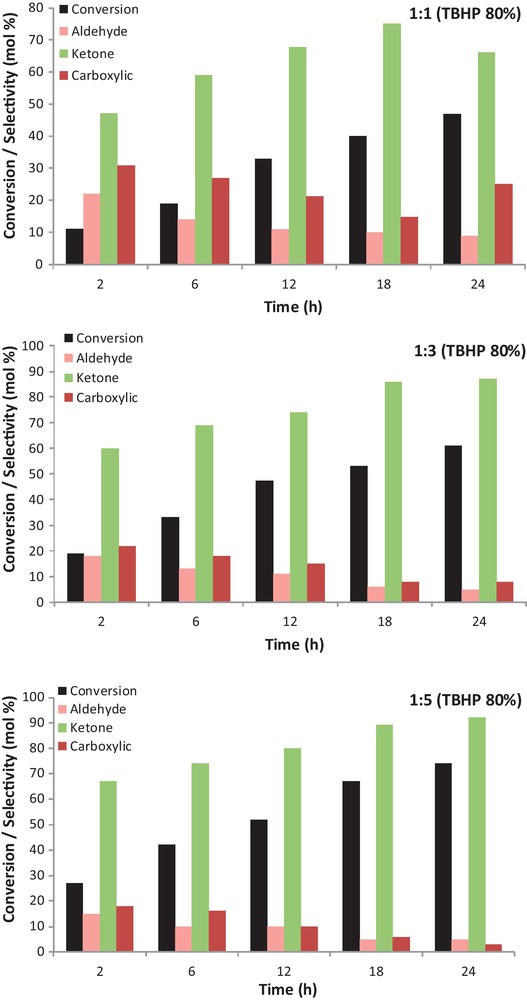
The influence of different oxidant ratios on the ethylbenzene oxidation by the SiO2/Al2O3-APTMS-BPKCo catalyst. Conditions: ethylbenzene, 2 mmol; Cat., 50 mg; T, 100 °C; solventless.
Increasing the temperature for accelerating the reaction is apparently effective. Consequently, the temperature effect (50 °C, 80 °C and 100 °C) was also monitored on the oxidation of EB at the EB:TBHP molar ratio of 1:1 after 24 h in the presence of NCC (Fig. 5). Generally, by increasing reaction temperature and reaction time, the conversions grow higher (74%) and also the reaction selectivity to acetophenone increased (92%) while the selectivity to benzaldehyde and benzoic acid decreased when the reaction was carried out at 100 °C. Thus raising temperature is effective for the oxidation reaction since the energy was not sufficient for the activation of oxygen molecules or the catalytic circulation at the low temperature.
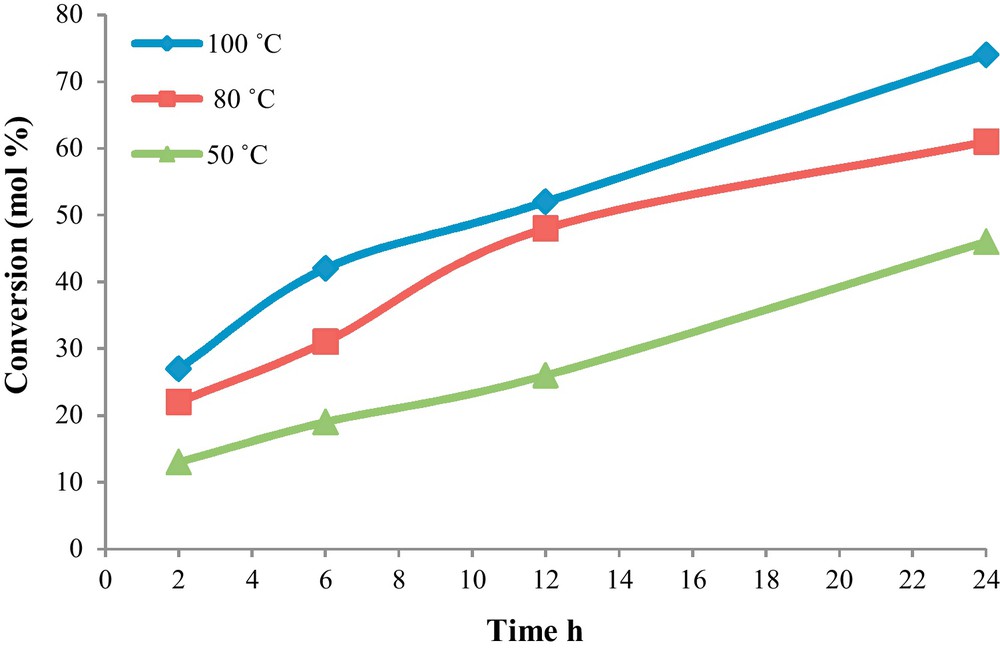
The influence of reaction temperature on the ethylbenzene oxidation and selectivity to acetophenone by the SiO2/Al2O3-APTMS-BPK-Co catalyst. Conditions: ethylbenzene, 2 mL; Cat., 50 mg; TBHP, 10 mmol; solventless.
To estimate the reusability of NCC, after the first run, the catalyst was filtrated and washed with ether and dried at 80 °C under vacuum and then used for the next run under the same conditions. For the third performance of the catalyst, 89% selectivity with 69% conversion still can be gained (Fig. 6). A few losses of activity and selectivity was revealed, satisfying that NCC has high stability during the catalytic process.
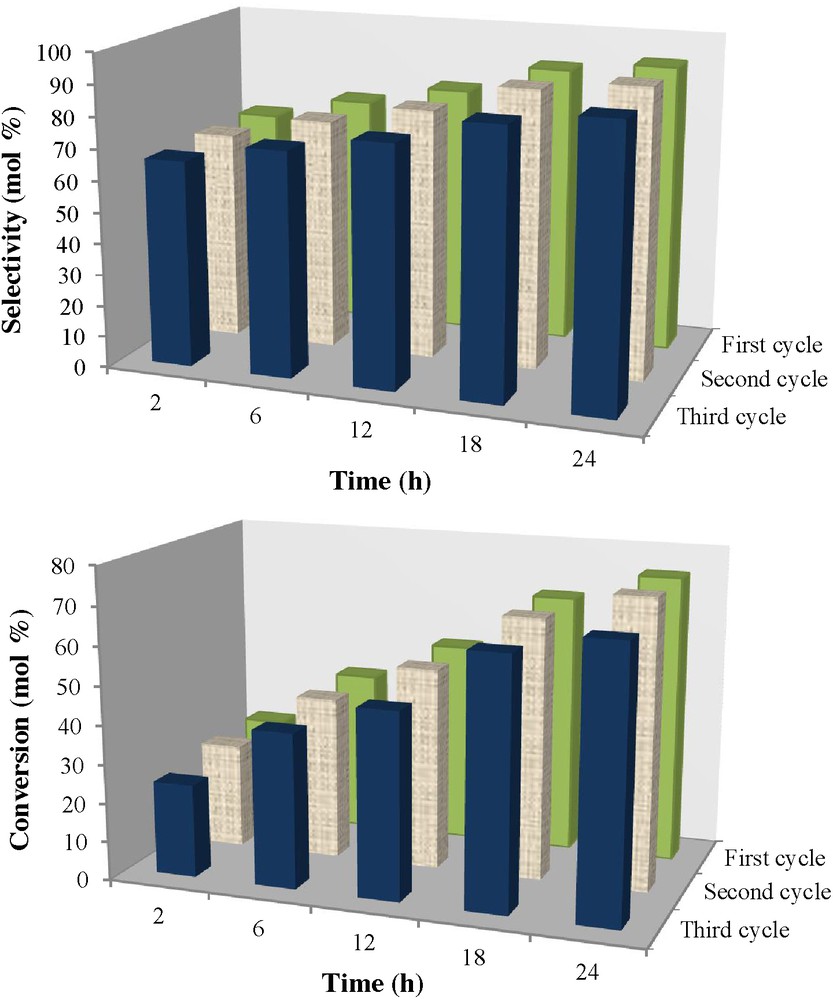
Reusability of the Co-nano-catalyst on the oxidation of ethylbenzene and selectivity to acetophenone. Conditions: ethylbenzene, 2 mmol; Cat., 50 mg; TBHP, 10 mmol; T, 100 °C; solventless.
Finally, another experiment was carried out in order to prove the important role of the catalyst in the oxidation of EB. First, extra EB was added to the filtrate after the removal of catalyst. Under these conditions, no more products were produced. Then, to prove whether metal is coordinated to the Schiff base ligand or with Si -OH and Al -OH groups on the surface of SiO2/Al2O3, some Co acetate was added to the blank SiO2/Al2O3, and the reaction took place in the same conditions; then the conversion of ethylbenzene was almost unnoticeable. In Table 3, our catalyst is being compared with the literature catalysts. The catalyst and applied method in this paper have the advantages in terms of heterogeneous nature, high reusability, high conversions and selectivity of the catalyst.
Comparison of literature catalysts and our catalyst system for oxidation of ethylbenzene.
Entry | Catalytic system | Reaction conditionsa | EB conversion (%) | Selectivity | ||
AP | BZ | Other | ||||
1 | Bhoware and Singh [31] | 0.05 g Co-MCM-41(100), TBHP, solvent-free, 80 °C, 24 h | 26 | 85 | 9.1 | 5.9 |
2 | Wang et al. [32] | 0.09 g CoTPP-P(4VP-co-st)/SiO, O2, solvent -free, 95 °C, 0.2 h | 24 | 98 | – | 2 |
3 | Qi et al. [33] | 2 mmol Co(III) complex, O2, solvent-free, 150 °C, 4 h | 70 | 90 | 0.7 | 9.1 |
4 | Veterivel and Pandurangam [34] | 0.3 g Mn-MCM-41, TBHP, solvent-free, 80 °C, 24 h | 70 | 39 | 10 | 50 |
5 | Xavirert et al. [44] | 0.05 g YCu, H2O2, benzene, 70 °C, 8 h | 60 | 100 | – | – |
6 | Radhika and Sugunam [45] | 0.1 g 6VC, H2O2, CH3CN, 60 °C, 6h | 16 | 75 | 11 | 16 |
7 | Jothiramalingam et al. [46] | 0.1 g Zr-k-OMS, TBHP, CH3CN, 65 °C, 10 h | 62 | 98 | – | – |
8 | Jana et al. [47] | 2.45 g NiAl-hydrotalcite, O2, solvent-free, 135 °C, 9 h | 47 | 99 | – | 0.7 |
9 | Waters [48] | 0.1 g CNCr-2, TBHP, CH3CN, 70 °C, 8 h | 45 | 69 | – | 30 |
10 | This work | 5 mg Si/Al-ATMPS-BPK-Co, TBHP, solvent-free, 80 °C, 24 h | 69 | 92 | 6 | 2 |
a Co TPP-P (4VP-co-st)/SiO2: Co tetraphenylporphrins on P (4VP-co-st)/SiO2, YCu-fsal: Y zeolite encapsulated Cu(II) Complexe of 3-formylsalicylic acid, 6VC: Vanadia/Ceria catalysts (6 wt% of V2O5), Zr-K-OMS: Zireonium doped K-OMS-2 type manganese oxide material, CNCr-2: Cu1−xNixCr2O4 (x = 0.5).
3.3 Oxidation of cyclohexene
To examine the catalytic activity of nano-cobalt catalyst, oxidation of cylohexene was carried out under solventless conditions at 100 °C by applying TBHP as oxidant (Scheme 3). The products of this reaction were 2-cyclohexene-1-one (1), cyclohexene oxide (2), and 1,2-cyclohexandiol (3) which were identified by GC-MS and quantified by GC analysis. Fig. 7 shows that at the same temperature (100 °C), but with different molar ratios of CH:TBHP (1:1, 1:3 and 1:5), cyclohexene conversion and the selectivities of the products have been changed. As the molar ratios of CH:TBHP and reaction time were raised to (1:5) at 24 h, respectively, the cyclohexene conversion and the selectivity to 2-cyclohexene-1-one increased from 36 to 55 and 67 to 88%, respectively, while the selectivities to cyclohexene oxide and 2-cyclohexene-1-ol decreased (Fig. 7). In general, the allylic oxidation of cyclohexene requires 5:1 stoichiometric molar ratio of TBHP:substrate. However, under the reaction condition used in this work, all of the TBHP cannot be consumed for this reaction owing to a possible partial decomposition and/or remaining unreacted TBHP. Therefore, the reaction may consume higher amount of TBHP for a complete conversion [48]. So, it could be concluded from the results that under the mentioned reaction conditions, the allylic hydrogen is more reactive than the CC double bond. However, the abstraction of hydrogen from the allylic carbon leads to allylic radical, which requires lower activation energy than the reaction at the double bond.

Oxidation of cyclohexene catalyzed by Si/Al-APTMS-BPK-Co.
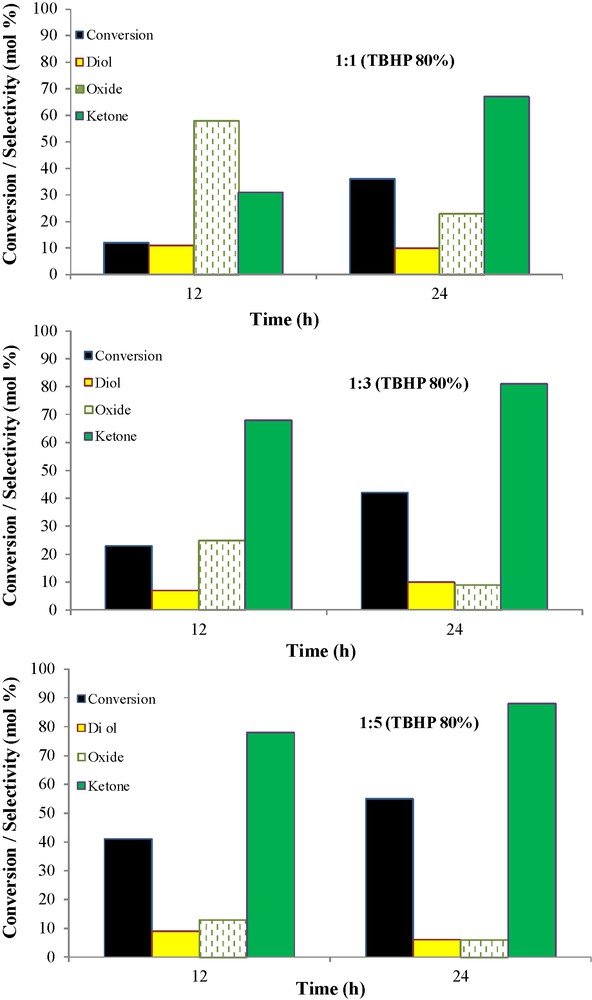
The influence of different oxidant ratio on the cyclohexene oxidation by the Si/Al-APTMS-BPK-Cocatalyst. Conditions: cyclohexene, 2 mmol; Cat., 50 mg; T, 100 °C; solventless.
In order to examine the reusability of the immobilized cobalt catalyst onto the SiO2/Al2O3, several successive experiments were carried out by application of the used catalyst. According to Fig. 8, there is no significant loss of activity and selectivity even after the fifth run. Therefore, the results clearly proved that the NCC catalyst conversion of cyclohexene (55%) with ca. 88.0% selectivity to 2-cyclohexene-1-one under solventless condition. Furthermore, in Table 4, our catalyst is being compared with the literate catalysts.
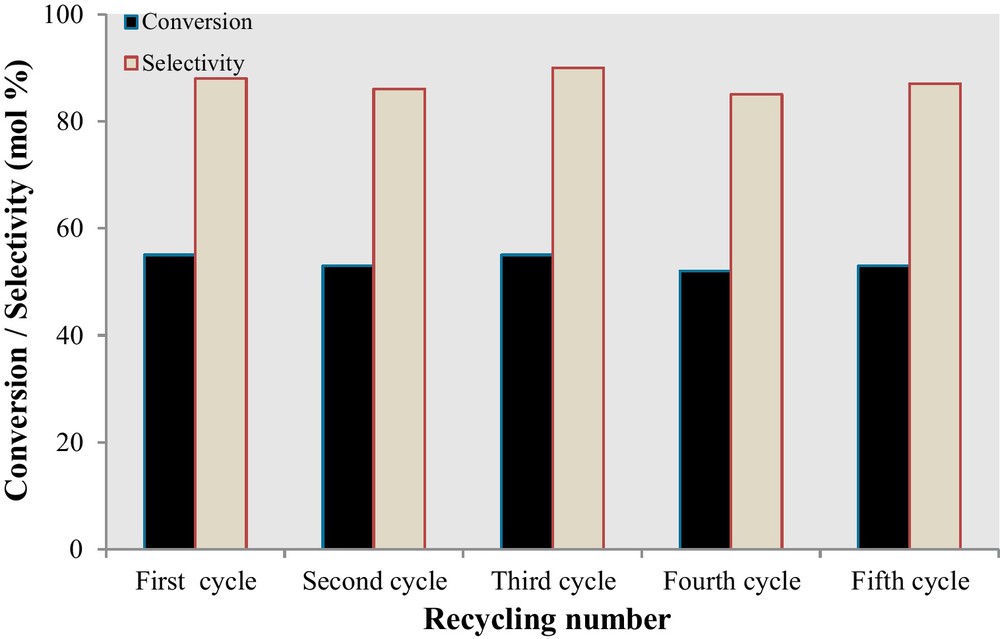
Reusability of the Co-nano-catalyst in the oxidation of cyclohexene and selectivity to 2-cyclohexene-1-one. Conditions: cyclohexene, 2 mmol; Cat., 50 mg; TBHP, 10 mmol; 24 h; T, 100 °C; solventless.
Comparison of literature catalysts and our catalyst system for oxidation of cyclohexene.
Entry | Catalytic system | Reaction condition | Conversion (%) | Selectivitya | |
Keton | Other product | ||||
1 | Luque et al. [37] | 0.2 Co-salen-SBA 15, H2O2, MW300W, 90 °C, 0.33 h | > 99 | 89 | 11 |
2 | Ghiaci et al. [35] | 5 mg (Ru/Co/Ce) (THNO), TBHP, CH2Cl2 | 73.6 | 80.3 | 19.7 |
3 | Salavati and Niasari [36] | 1.02 × 10−5 mol [Co(H4C6N6S2)], TBHP, CH2Cl2, rt, 8 h | 43.6 | 55.6 | 44.4 |
4 | Baricelli et al. [38] | 0.01 mol [Ir (CH3CN)4 NO2] (PF6)2, O2, CH3 CN 120 °C, 12 h | 43.2 | 74 | 18 |
5 | Sehlotho and Nykong [39] | (1.7 mg/ml) CoPc, TBHP, DMF/dichloromethane, rt, 8 h | 92 | 60.8 | 39.2 |
6 | Li et al. [40] | Trans–RuCl2 [k3–1R, 2R–P(NH)–(NH) P]Pph3/CNTS, O2, solvent-free, 105 °C, 1 h | 80.7 | 49.0 | 18.2 |
7 | Dapurkar et al. [41] | 20 mg CrMCM–41, O2, 70 °C, 24 h | 41.5 | 74.2 | 25.8 |
8 | Malumbazo and Mapolie [42] | (0.01 mol) t-BuCo/MCM-41, H2O2, O2, CH3CN 60 °C, 5 h | 50 | 100 | – |
9 | Malumbazo and Mapolie [42] | (0.01 mol) tBu Co/Davisil 710, H2O2, O2, CH3CN 60 °C, 5 h | 45 | 56 | 44 |
10 | Sujandi Han et al. [43] | 100 mg Co (III) SBA–15, H2O2, CH3CN 40 °C, 12 h | 34.8 | 10.2 | 89.8 |
11 | This work | 5 mg SiAl-ATMPS-BPK-Co, TBHP, solvent-free, 80 °C, 24 h | 55 | 88 | 12 |
a Ketone: 2-cyclohexene-1-one.
3.4 Oxidation of benzyl alcohol
The liquid-phase oxidation of benzyl alcohol was performed by TBHP at 25, 40, 60, 80 and 100 °C on the immobilized NMC under different conditions without the use of any solvent (Scheme 4). Table 5 confirms the highness of catalytic activity and excellent selectivity to benzaldehyde (100%) in all experimental condition. GC analysis showed that benzaldehyde was the sole product (confirmed by performing three replicate experiments). With increasing the temperature from 25 to 100 °C, the benzyl alcohol conversion increases. However, by reusing the catalyst, the percentage of benzyl alcohol conversion and benzaldehyde selectivity are constant after three times. The nature of the recovered catalyst after reusing three times has been followed by FTIR spectroscopy, and no significant change was observed. This indicates that the heterogeneous nano-cobalt catalyst with high catalytic performance and stability for the selective oxidation of benzyl alcohol was produced by covalent anchoring of cobalt acetate on the nano-sized SiO2/Al2O3.
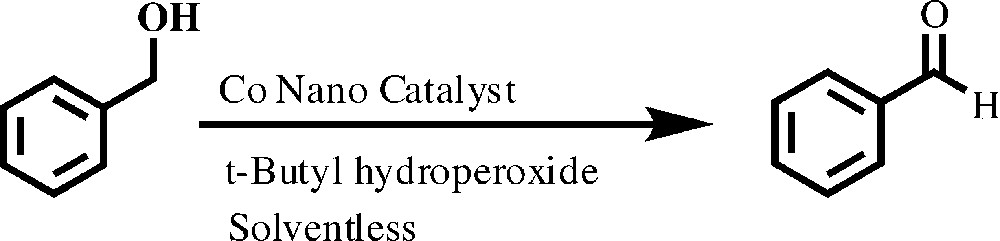
Oxidation of benzyl alcohol to benzyl aldehyde.
Oxidation of benzyl alcohol with heterogeneous cobalt nano-catalyst.
Reaction temperature (T °C) | Conversion (mol %) | Selectivitye (mol %) |
25 | 49.1 | Benzaldehyde |
40 | 65.8 | Benzaldehyde |
60 | 78.8 | Benzaldehyde |
80 | 91.0 | Benzaldehyde |
100 | 97.9 | Benzaldehyde |
60a | 87.3 | Benzaldehyde |
60b | 99.6 | Benzaldehyde |
60c | 78.7 | Benzaldehyde |
60d | 78.5 | Benzaldehyde |
a Benzyl alcohol: TBHP (1:2).
b Benzyl alcohol: TBHP (1:3).
c Second run.
d Third run.
e Selectivity is 100%.
4 Conclusions
According to this study, a simple and novel system including a nano-Co(II)-catalyst as high efficient heterogenous catalyst was investigated. The catalytic active system designed by a nano-heterogeneous cobalt associated with TBHP under solventless condition exhibited efficient catalytic activity in the selective oxidation of ethylbenenze, cyclohexene, and benzyl alcohol. The catalyst appears to be of particular interest for the industrial production of aromatic and alkyl ketones under mild condition.