1 Introduction
Quinoxaline and pyrazine derivatives represent an imperative class of nitrogen-containing heterocycles as they have received considerable interest from pharmaceutical to academic and industrial perspectives. For example, drug formulations with the core of quinoxaline, such as Lamprene, are currently available and they are privileged structures in combinatorial drug discovery. Besides their interesting therapeutic properties, such as antiviral, antibacterial, anti-inflammatory, and antiprotozoal and as kinase inhibitors [1,2], they have also been evaluated as anticancer, anthelmintic, antifungal, and insecticidal agents [3]. The quinoxaline nucleus is a part of several antibiotics, such as echinomycin, levomycin, and actinomycin that are known to inhibit the growth of Gram-positive bacteria and are active against various transplantable tumours [4,5]. Furthermore, they have found applications as dyes, electroluminescent materials, organic semiconductors, cavitands, chemically controllable switches, and DNA cleaving agents [6–8]. In this regards, various strategies have been introduced for the synthesis of these scaffolds, which include condensation of arene-1,2-diamines with 1,2-ketones (I2, DMSO; I2, MeCN; montmorillonite K-10; PEG-400; solid phase); CAN; MeOH–AcOH (9:1); Ac2O, reflux; carbon-doped MoO3–TiO2 (CMT); p-toluene sulfonic acid (PTSA) as Brønsted acid catalyst in aqueous sodium p-toluene sulfonate (NaPTS) solution (Brønsted acid hydrotrope-combined catalyst (BAHC); lead (II) bromide; lithium chloride), oxidative cyclization of α-hydroxy ketones with 1,2-diamines (MnO2; 1 mol% MnO2, microwaves; Pd(OAc)2; MnO2–NaBH4; PEG-400), cyclization–oxidation of phenacyl bromides with 1,2-diamines (HClO4–silica gel), and oxidative coupling of epoxides with ene-1,2-diamines [bismuth powder, Cu(OTf)2] [9–23]. However, many of these methods suffer from several drawbacks such as the use of strong oxidizing agents and expensive metal catalysts, and also the yields are far from satisfactory. Therefore, the development of a simple, convenient, and general approach would certainly be useful in generating combinatorial libraries for drug discovery.
Over the past decade, zirconium compounds have been found to play an ever-increasing role in organic synthesis, which has been recognized by a number of excellent review articles and books covering various aspects of zirconium compounds as the catalysts or reagents in synthetic organic chemistry [24,25].
Herein, we set out to explore a new green synthetic method for the synthesis of 2,3-disubstituted quinoxalines, pyrazines and pyridopyrazines via zirconium(IV) oxide chloride-catalyzed condensation of related 1,2-diamines and 1,2-dicarbonyl compounds in water.
A survey of the literature shows that there are not many reports on the synthesis of pyridopyrazine derivatives [15]. The most convenient method for the synthesis of these compounds is the reaction of 2,3- and 3,4-diaminopyridines with different diketones. The problem is that these diaminopyridines are almost unreactive toward diketones in normal conditions. Herein, our contribution is intended to extend the scope of available methods for the synthesis of pyridopyrazines, pyrazines and 2,3-disubstituted quinoxalines, and to describe the application of a new transition metal-based catalyst in their synthesis.
2 Result and discussion
In continuation of our efforts in applying metal oxides in organic synthesis [26,27], we tried ZrOCl2·8H2O-catalyzed condensation reaction of 1,2-diamines and 1,2-diketones for the preparation of the corresponding pyridopyrazines, pyrazines, and 2,3-disubstituted quinoxalines (Scheme 1).
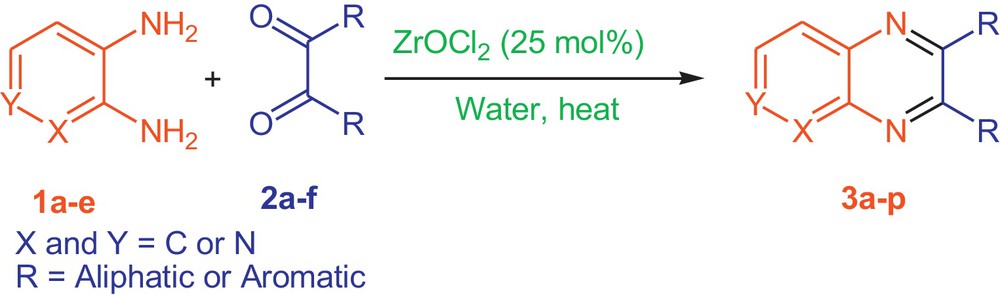
General route for the synthesis of desired heterocycles.
In this regard, we initially attempted the condensation of 2,3-diaminopyridine (1a) and benzil (2a, PhCOCOPh) as a model reaction under various reaction conditions. The optimization of the reaction conditions showed that excellent conversion of the starting material into the product was achieved in 2 h by using ZrOCl2·8H2O as a catalyst under refluxing water. In a typical procedure, the reaction of 2,3-diaminopyridine 1a (1.0 mmol, 1.0 equiv) and benzil 2a (1 mmol, 1 equiv) in the presence of ZrOCl2·8H2O (25 mol%) under refluxing water for 2 h afforded compound 3a in 92% yield (Table 1, entry 1). The proposed mechanism for the synthesis of 3a in the presence of ZrOCl2 is shown in Scheme 2.
Synthesis of desired heterocyclic derivatives catalyzed by ZrOCl2·8H2O in water.
Entry | Diamine 1 | Dicarbonyl 2 | Product 3a | Time (h) | Yield (%)b |
1 | 1a | 2 | 92 | ||
2 | 1a | 2 | 93 | ||
3 | 1a | 3 | 88 | ||
4 | 1a | 2.5 | 85 | ||
5 | 1b | 2c | 3 | 86 | |
6 | 1b | 2b | 2.5 | 88 | |
7 | 1b | 2a | 2.5 | 91 | |
8 | 1b | 2.5 | 87 | ||
9 | 1b | 2d | 2.5 | 86 | |
10 | 1c | 2a | 1 | 95 | |
11 | 1c | 2d | 1 | 91 | |
12 | 1d | 1 | 90 | ||
13 | 1e | 2a | 1 | 97 | |
14 | 1e | 2c | 2 | 92 | |
15 | 1e | 2b | 1 | 95 | |
16 | 1e | 2f | 0.5 | 98 |
a All reactions were run under the following conditions: 1,2-diamine 1 (1 mmol, 1 equiv), diketone 2 (1.0 mmol, 1 equiv) and catalyst (25 mol%) in refluxing water (2 mL) were heated for appropriate times.
b Isolated yield.
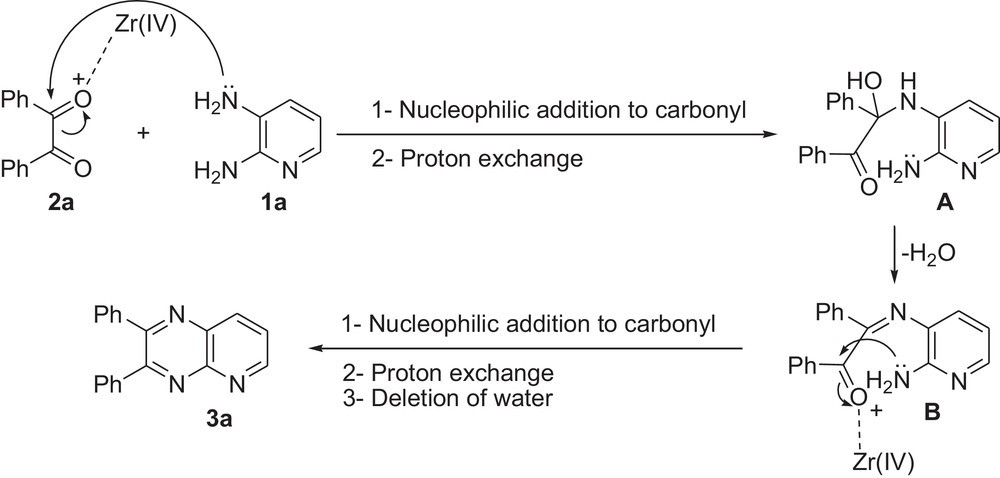
Proposed mechanism for the formation of 3a from starting materials in the presence of ZrOCl2.
In order to show the generality and scope of this new protocol, a variety of 1,2-diamines and 1,2-diketones were examined. The results are summarized in Table 1 (entries 1–16). Most of the reactions proceeded very cleanly and no undesirable side products were observed. The results showed that besides electron-rich diamines, such as ethylene dimine and 1,2-phenylene diamine, the electron-deficient ones, such as 2,3-diaminopyridine and 3,4-diaminopyridine can provide the desired products in good to excellent yields (Table 1, entries 1–15). Furthermore, our protocol proved to be a good route for the synthesis of multifunctional and highly conjugated pyrazines (Table 1, entries 4, 8, 9, 11 and 12).
Finally, we investigated the recycling of reaction media in a subsequent reaction, for example, in the reaction of o-phenylenediamine (1e) with benzil (2a), and ZrOCl2 was reused five times without any appreciable loss of activity.
3 Conclusions
In summary, we successfully developed a simple, efficient and eco-friendly method for the synthesis of pyridopyrazines, pyrazines and quinoxalines from 1,2-diamines and various 1,2-diketones using the cheap and readily available ZrOCl2·8H2O catalyst. Not only the electron-rich diamines (ethylene dimine and 1,2-phenylene diamine), but also the electron-deficient ones (2,3-diaminopyridine and 3,4-diaminopyridine), provided the desired products in good to excellent yields in water as a green solvent.
4 Experimental method
4.1 General
Commercial grade of 1,2-phenylenediamine, diaminopyridines, 1,2-dicarbonyl compounds and zirconium(IV) oxide chloride were purchased from Sigma–Aldrich, Merck and Acros organics companies. The solvents were of analytical grade and used as received. Silica gel (Merck, grade 9385, 230–400 mesh, 60 A°) for column chromatography was used as received. All other reagents were purchased from Merck and used as received unless otherwise noted. The course of the synthesis and purity of the products were followed by TLC on silica gel plates (Merck, silica gel 60 F254, ready to use), using dichloromethane:methanol (9:1) or n-hexane:ethyl acetate (1:3) as eluents. The eluent for column chromatography was the same as for TLC.
The melting points were recorded using a Buchi B540 melting point apparatus and are uncorrected. 1H and 13C NMR spectra were recorded at room temperature on a Bruker AC 300, 400 or 500 MHz spectrometers using CDCl3 or DMSO-d6 as the NMR solvents. 1H NMR spectra are referenced to tetramethylsilane (0.00 ppm) and 13C NMR spectra are referenced from the solvent central peak (for example, 77.23 ppm for CDCl3). Chemical shifts are given in ppm. IR spectra were taken as KBr pellets using a Jasco 4200 FT-IR spectrophotometer. IR is reported as characteristic bands (cm−1) at their maximum intensity.
4.2 General procedure for the synthesis of compounds 3a–3p
For the synthesis of entitled heterocycles, a round bottom flask equipped with a stir bar was charged with 1,2-phenylenediamine (1.0 mmol), 1,2-diketones (1.0 mmol), water (2 mL) and zirconium(IV) oxide chloride (25 mol%). The resulting mixture was heated in an oil bath at 100 °C for the appropriate time, and the course of the reaction was monitored using TLC on silica gel. Finally, the reaction mixture was cooled and the crude mixture was purified by column chromatography or crystallization to give the desired product. The authenticity of the products was established by comparing their melting points with data of the literature and by analyzing the spectroscopic data of 1H and 13C NMR and IR [9–16,26,27].
4.3 Spectral data for compounds 3a–3p
4.3.1 2,3-Diphenylpyrido[2,3-b]pyrazine (3a)
Yield 92%; yellow solid, mp: 141–143 °C. IR (KBr) υ (cm−1): 3056, 1544, 1430, 1384, 1332, 1068, 1019, 780, 697. 1H NMR (500 MHz, DMSO, d6) δ (ppm): 7.31–7.49 (m, 10H), 7.86 (dd, J = 8.2, 4.1 Hz, 1H), 8.56 (dd, J = 8.2, 1.2 Hz, 1H) 9.14 (m, 1H). 13C NMR (125 MHz, CDCl3) δ (ppm): 156.5, 155.3, 154.9, 150.0, 139.1, 138.7, 136.5, 130.6, 130.6, 130.0, 129.9, 128.9, 126.8.
4.3.2 2,3-Bis(4-fluorophenyl)pyrido[2,3-b]pyrazine (3b)
Yield 93%; yellow solid, mp: 140–142 °C. IR (KBr) υ (cm−1): 2925, 1728, 1597, 1548, 1508, 1446, 1386, 1331, 1226, 832. 1H NMR (500 MHz, CDCl3) δ (ppm): 7.02–7.09 (m, 4H), 7.53–7.63 (m, 4H), 7.72 (q, J = 8.3 Hz, 1H), 8.49 (dd, J = 8.3, 1.7 Hz, 1H), 9.17 (dd, J = 4.1, 1.7 Hz, 1H) 13C NMR (75 MHz, CDCl3) δ (ppm): 165.2, 165.1, 161.9, 161.8, 154.9, 154.3, 153.3, 149.7, 138.0, 136.1, 134.4, 134.3, 134.0, 133.9, 132.3, 132.2, 131.8, 131.7, 125.4, 115.8, 115.6, 115.5, 115.3. Anal. Calcd for C19H11F2N3 (319.3): C, 71.47; H, 3.47; N, 13.16. Found: C, 71.64; H, 3.59; N, 13.38.
4.3.3 2,3-Bis(4-methoxyphenyl)pyrido[2,3-b]pyrazine (3c)
Yield 88%; yellow solid, mp: 137–139 °C. IR (KBr) υ (cm−1): 2933, 2839, 1605, 1513, 1447, 1384, 1251, 1175, 1023, 833. 1H NMR (300 MHz, CDCl3) δ (ppm): 3.84 (d, 6H), 6.85–6.91 (m, 4H), 7.53–7.68 (m, 5H), 8.45 (dd, J = 8.4, 1.8 Hz, 1H), 9.11 (dd, J = 4.2, 1.8 Hz, 1H). 13C NMR (75 MHz, CDCl3) δ (ppm): 160.7, 155.7, 154.2, 153.5, 149.8, 137.8, 135.8, 131.8, 131.2, 131.1, 130.7, 124.7, 113.9, 113.6, 55.3, 55.3.
4.3.4 Acenaphtho[1,2-b]pyrido[2,3-e]pyrazine (3d)
Yield 85%; yellow solid, mp: 225–227 °C. IR (KBr) υ (cm−1): 3051, 1612, 1561, 1433, 1373, 1212, 1098, 1034, 826, 771. 1H NMR (300 MHz, CDCl3) δ (ppm): 7.69–7.90 (m, 3H), 8.12 (dd, J = 8.2, 2.7 Hz, 2H), 8.40–8.57 (m, 3H), 9.12 (d, J = 4.1 Hz, 1H). 13C NMR (75 MHz, CDCl3) δ (ppm): 157.1, 154.9, 152.4, 150.6, 138.3, 137.2, 136.4, 131.2, 130.9, 130.2, 130.0, 129.9, 128.9, 128.7, 124.3, 123.3, 122.3. Anal. Calcd for C17H9N3 (255.2): C, 79.99; H, 3.55; N, 16.46. Found: C, 79.75; H, 3.80; N, 16.52.
4.3.5 2,3-Bis(4-methoxyphenyl)pyrido[3,4-b]pyrazine (3e)
Yield 86%; yellow solid partial to green, mp: 145–147 °C. IR (KBr) υ (cm−1): 3025, 2923, 1604, 1510, 1460, 1383, 1248, 1173, 1028, 835. 1H NMR (500 MHz, CDCl3) δ (ppm): 3.84 (S, 6H), 6.89 (d, J = 8.7 Hz, 2H), 7.52 (d, J = 8.0 Hz, 2H), 7.93 (d, J = 5.7 Hz, 1H), 8.77 (d, J = 5.7 Hz, 1H), 9.53 (s, 1H). 13C NMR (75 MHz, CDCl3) δ (ppm): 160.9, 160.6, 157.4, 154.9, 154.1, 146.9, 143.4, 136.2, 131.4, 131.2, 130.9, 130.8, 121.2, 113.9, 55.3.
4.3.6 2,3-Bis(4-fluorophenyl)pyrido[3,4-b]pyrazine (3f)
Yield 88%; orange solid, mp: 132–134 °C. IR (KBr) υ (cm−1): 3049, 1597, 1509, 1381, 1325, 1230, 835. 1H NMR (500 MHz, CDCl3) δ (ppm): 7.07 (m, 4H), 7.51–7.55 (m, 4H), 7.96 (d, J = 5.8 Hz, 1H), 8.83 (d, J = 5.8 Hz, 1H), 9.58 (s, 1H). 13C NMR (75 MHz, CDCl3) δ (ppm): 165.3, 165.2, 162.0, 161.8, 156.6, 154.4, 154.0, 147.5, 143.5, 136.2, 134.1, 132.0, 131.8, 131.8, 131.7, 121.2, 115.9, 115.6. Anal. Calcd for C19H11F2N3 (319.3): C, 71.47; H, 3.47; N, 13.16. Found: C, 71.64; H, 3.59; N, 13.33.
4.3.7 2,3-Diphenylpyrido[3,4-b]pyrazine (3g)
Yield 91%; white solid partial to green, mp: 170–172 °C. IR (KBr) υ (cm−1): 3047, 2963, 1590, 1542, 1383, 1326, 1024, 808, 696. 1H NMR (500 MHz, CDCl3) δ (ppm): 7.34–7.55 (m, 10H), 7.99 (d, J = 5.7 Hz, 1H), 8.83 (d, J = 5.7 Hz, 1H), 9.60 (s, 1H). 13C NMR (75 MHz, CDCl3) δ (ppm): 157.9, 155.3, 154.4, 147.3, 143.5, 138.2, 136.3, 129.8, 129.8, 129.7, 129.4, 128.4, 121.3.
4.3.8 Dibenzo[f,h]pyrido[3,4-b]quinoxaline (3h)
Yield 87%; yellow solid, mp: 216–218 °C. IR (KBr) υ (cm−1): 3025, 2921, 1600, 1499, 1387, 1343, 1216, 1041, 828, 754, 719. 1H NMR (300 MHz, CDCl3) δ (ppm): 7.69–7.84 (m, 4H), 8.07 (d, J = 5.7 Hz, 1H), 8.48 (d, J = 7.8 Hz, 2H), 8.86 (d, J = 6.0 Hz, 1H), 9.30 (d, J = 7.8 Hz, 2H), 9.73 (s, 1H). 13C NMR (75 MHz, CDCl3) δ (ppm): 155.1, 146.2, 143.9, 136.9, 135.9, 132.8, 131.5, 131.0, 130.5, 129.5, 129.4, 128.2, 128.1, 127.0, 126.5, 123.9, 123.0, 122.9, 121.3.
4.3.9 Acenaphtho[1,2-b]pyrido[3,4-e]pyrazine (3i)
Yield 86%; orange solid, mp: 245–247 °C. IR (KBr) υ (cm−1): 3031, 2922, 1611, 1569, 1424, 1296, 1103, 961, 830, 771. 1H NMR (300 MHz, CDCl3) δ (ppm): 7.82 (m, 2H), 7.97 (d, J = 5.7 Hz, 1H), 8.11 (m, 2H), 8.37 (m, 2H), 8.79 (d, J = 8.7 Hz, 1H), 9.54 (s, 1H). 13C NMR (75 MHz, CDCl3) δ (ppm): 157.5, 155.2, 154.0, 147.0, 144.1, 137.1, 136.3, 130.8, 130.6, 130.0, 129.9, 128.8, 128.7, 122.9, 122.4, 122.0.
4.3.10 5,6-Diphenyl-2,3-dihydropyrazine (3j)
Yield 95%; white solid, mp: 154–156 °C. IR (KBr) υ (cm−1): 3028, 2943, 2831, 1610, 1551, 1439, 1261, 986, 766, 698. 1H NMR (500 MHz, CDCl3) δ (ppm): 3.69 (s, 4H), 7.23 (d, J = 7.8 Hz, 4H), 7.30 (m, 2H), 7.39 (d, J = 8.0 Hz, 4H). 13C NMR (75 MHz, CDCl3) δ (ppm): 45.8, 127.9, 128.1, 129.6, 137.7, 160.3.
4.3.11 8,9-Dihydroacenaphtho[1,2-b]pyrazine (3k)
Yield 91%; yellow solid, mp: 165–167 °C. IR (KBr) υ (cm−1): 2929, 2838, 1671, 1627, 1483, 1429, 1329, 1111, 961, 824, 771. 1H NMR (300 MHz, CDCl3) δ (ppm): 3.94 (s, 4H) 7.71 (m, 2H), 7.94–8.01 (m, 4H). 13C NMR (75 MHz, CDCl3) δ (ppm): 158.6, 131.7, 130.6, 128.5, 128.3, 118.7, 118.6, 44.9.
4.3.12 5,6-Dimethylpyrazine-2,3-dicarbonitrile (3l)
Yield 90%; white solid, mp: 162–164 °C. IR (KBr) υ (cm−1): 3006, 2239, 1770, 1717, 1536, 1389, 1197, 1123, 986, 793, 695. 1H NMR (500 MHz, CDCl3) δ (ppm): 2.70 (s, 6H). 13C NMR (125 MHz, CDCl3) δ (ppm): 158.4, 130.7, 113.6, 23.3.
4.3.13 2,3-Diphenylquinoxaline (3m)
Yield 97%; white solid, mp: 121–123 °C. IR (KBr) υ (cm−1): 3048, 2923, 1544, 1441, 1336, 765, 691. 1H NMR (500 MHz, CDCl3) δ (ppm): 7.32–7.38 (m, 6H), 7.52 (d, J = 7.8 Hz, 4H), 7.77 (q, J = 6.4 Hz, 2H), 8.18 (q, J = 9.5 Hz, 2H). 13C NMR (75 MHz, CDCl3) δ (ppm): 153.5, 141.2, 139.0, 129.9, 129.8, 129.2, 128.8, 128.2.
4.3.14 2,3-Bis(4-methoxyphenyl)quinoxaline (3n)
Yield 92%; white solid, mp: 145–147 °C. IR (KBr) υ (cm−1): 2932, 2836, 1605, 1510, 1462, 1344, 1291, 1244, 1173, 1026, 832. 1H NMR (300 MHz, CDCl3) δ (ppm): 3.83 (s, 6H), 6.88 (d, J = 8.7 Hz, 4H), 7.50 (d, J = 8.7 Hz, 4H), 7.72 (m, 2H), 8.13 (m, 2H). 13C NMR (75 MHz, CDCl3) δ (ppm): 160.1, 153.0, 141.0, 131.5, 131.2, 129.5, 129.0, 113.7, 55.3.
4.3.15 2,3-Bis(4-fluorophenyl)quinoxaline (3o)
Yield 95%; white solid, mp: 133–135 °C. IR (KBr) υ (cm−1): 3063, 1600, 1510, 1476, 1395, 1342, 1227, 1158, 1051, 842, 763. 1H NMR (300 MHz, CDCl3) δ (ppm): 7.03–7.09 (m, 4H), 7.49–7.54 (m, 4H), 7.80 (q, J = 9.6 Hz, 1H), 8.16 (q, J = 9.0 Hz, 1H). 13C NMR (75 MHz, CDCl3) δ (ppm): 164.8, 161.5, 152.1, 141.2, 135.0, 134.9, 131.8, 131.7, 130.2, 129.1, 115.6, 115.4. Anal. Calcd for C20H12F2N2 (318.3): C, 75.46; H, 3.80; N, 8.80. Found: C, 75.58; H, 3.71; N, 8.91.
4.3.16 2,3-Dimethylquinoxaline (3p)
Yield 98%; white solid, mp: 102–104 °C. IR (KBr) υ (cm−1): 2998, 1564, 1484, 1433, 1393, 1321, 1206, 1161, 982, 760. 1H NMR (500 MHz, CDCl3) δ (ppm): 2.69 (s, 6H), 7.63 (d, J = 6.3 Hz, 2H), 7.94 (d, J = 6.1 Hz, 2H). 13C NMR (125 MHz, CDCl3) δ (ppm): 153.3, 141.0, 128.7, 128.2, 23.1.
Acknowledgments
We are grateful to the PNU for funding this work.