1 Introduction
The formylation of amines is a very useful reaction in the synthesis of pharmaceutically valuable compounds [1,2]. N-Formyl compounds have been extensively employed in different reactions, for example as an important amine-protecting group in peptide synthesis, as a precursor for the synthesis of formamidines or isocyanides and as a Lewis-base catalyst for allylation and synthesis of acid chlorides from carboxylic acids or hydrosilylation of carbonyl compounds [3–5].
A number of synthetic methods have been reported in the literature for the N-formylation of amines and various reagents are available for this reaction, such as acetic formic anhydride [6], chloral [7], activated formic acid using DCC [8] or EDCI [9], activated formic esters [10], ammonium formate [11], 2,2,2-trifluoroethyl formate [12], ZnO [13], and PEG-400 [14].
Earlier reported methods for the N-formylation of amines suffer from drawbacks, such as the use of expensive and toxic reagents and catalysts, the environmental pollution caused by the utilization of organic solvents, low yields, high temperatures, long reaction times, harsh reaction conditions, difficulty in availability or preparing the starting materials or catalysts, necessity to use catalysts, tedious work-up, waste control and formation of side products [6–14]. Therefore, the need for “greener” methods or chemical products that reduce or eliminate the use and generation of hazardous compounds is essential.
From the standpoint of “green chemistry” and for reasons of economy and pollution, solvent-free methods are of great interest to make the classical procedures more clean, safe and easy to perform [15].
Application of the ultrasound irradiation has received an increased attention in recent years and is able to activate several organic reactions [16a–c], but it has not been used as an alternative energy source for the N-formylation of amines. In an irradiated liquid, the collapse of bubbles caused by cavitation produces intense local heating and high pressures with very short lifetimes. These transient, localized hotspots can cause the reaction to take place rapidly. The hotspot has an equivalent temperature of roughly 5000 °C (9000 °F), a pressure of about 2000 atmospheres, a lifetime considerably less than a microsecond and heating and cooling rates above 10 billion °C/s [16b–d]. So, a large number of organic syntheses can be carried out in higher yields, shorter reaction time and milder conditions under ultrasound irradiation than with conventional methods.
In continuation of our nitrogen-containing compounds syntheses [17], we hereby report the N-formylation of amines at room temperature under solvent- and catalyst-free conditions using ultrasound irradiation (Scheme 1).

N-formylation of amines under ultrasound irradiation.
2 Result and discussion
Formic acid is neither explosive, flammable, expensive, moisture sensitive, nor very volatile. Also, it is a very useful and efficient reagent and can be easily stored [18]. For these reasons, it has received much attention among formylating agents in the N-formylation of amines. Aqueous formic acid (85%) has been reported as a formylating agent under conventional heating; however, this method needs a Dean–Stark trap under reflux conditions in toluene and involves long reaction times [19].
The reaction conditions for formylation of amines were optimized using aniline with different amounts of HCOOH (Table 1). When the reaction was carried out at room temperature or thermal conditions (60 °C) in the absence of ultrasonic irradiation, the formamide was obtained in low yield and after long reaction times (Table 1, entries 1–7), while in the presence of ultrasonic irradiation the yield increased from 94 to 97% and the reaction time is reduced to 2 min (Table 1, entries 7–12). Also, some reactions were carried out at higher temperatures, and it was found that there were no remarkable changes in the reaction time and yield (Table 1, entries 13 and 14).
N-formylation of 1.0 mmol of aniline under different reaction conditions without solvent and catalyst.
Entry | HCOOH (mmol) | Temperature (°C) | Time | Yield (%)a |
1 | 1 | rt | 1 hb | 0 |
2 | 2 | rt | 1 hb | 0 |
3 | 3 | rt | 5 hb | 0 |
4 | Excess (5 mmol) | rt | 5 hb | 0 |
5 | 1 | 60 | 3 hb | 20 |
6 | 2 | 60 | 3 hb | 30 |
7 | 3 | 60 | 2 hb | 45 |
8 | 1 | rt | 5 hc | 94 |
9 | 1.5 | rt | 2 hc | 95 |
10 | 2 | rt | 1 hc | 97 |
11 | 2.5 | rt | 2 minc | 97 |
12 | Excess (5 mmol) | rt | 2 minc | 96 |
13 | 2.5 | 40 | 2 minc | 95 |
14 | 2.5 | 50 | 2 minc | 96 |
a Yields are after work-up.
b In the absence of ultrasonic irradiations.
c In the presence of ultrasonic irradiations.
The first principle of green chemistry states that it is better to prevent waste production than to treat waste or clean it up after it has been created [15b]. So, in the reaction of aniline with formic acid (mole ratio 1:1), after completion of the reaction, there is no formic acid residue and no side product in the reaction mixture. Also, no organic solvents were used for the extraction of the product in the work-up step and the method did not require any further purification. By increasing the mole ratio of formic acid from 1 to 2.5, the reaction time was clearly reduced; however it had no significant effect on the yield of the product (Table 1, entries 9, 10 and 11). The best result (97%) with a very short reaction time (2 min) was obtained with 2.5 mmol of formic acid (Table 1, entry 11). The use of an excess of formic acid (5 mmol) had no significant effect on the reaction time and yield (Table 1, entry 12).
It is important to mention that after completion of the reaction (aniline: formic acid, 1:2.5), the recovery of an excess of formic acid from the reaction mixture is important. There are several methods for recovery of formic acid, such as extractive and azeotropic distillation [20], but due to the high cost of the latter, extraction is a preferred method.
The conversion of formic acid into clean fuel has been considered to be the most promising of the recycling methods. Among energy sources, hydrogen has received much attention as an alternative and clean energy resource. However, the lack of convenient methods for storage and delivery of the hydrogen gas strongly restricts its application. One of the most promising methods for the preparation of hydrogen involves the steam reforming of methane and the water–gas shift reaction at high temperatures (> 200 °C) [21]. Formic acid can be used as a suitable hydrogen storage to minimize the external hydrogen requirements [22]. A number of the most important methods have been reported in recent years for the decomposition of formic acid to H2 (as a clean fuel) and CO2 (as a hydrogen carrier) through metal-catalyzed processes under very mild conditions [22]. In a recent publication in Green Chemistry, Himeda investigated the decomposition of formic acid using iridium catalyst in H2O [23]. Therefore, to prevent the generation of waste or pollution and in compliance with the first principle of green chemistry, the excess of formic acid can be recovered and decomposed to CO2 and H2 according to the literature.
The reactivities of several aromatic, heterocyclic and aliphatic amines were tested in the N-formylation reaction in solvent- and catalyst-free conditions under ultrasound irradiation at room temperature; the results were indicated in Table 2.
Green N-formylation of different amines by ultrasound irradiation.
Entry | Substrate | Product | Time (min) | Yield (%)a |
1 | 4 | 97 | ||
2 | 40 | 96 | ||
3 | 210 | 95 | ||
4 | 5 | 93 | ||
5 | 10 | 95 | ||
6 | 18 | 96 | ||
7 | 110 | 97 | ||
8 | 240 | 95 | ||
9 | 8 | 94 | ||
10 | 2 | 95 | ||
11 | 14 | 94 | ||
12 | 2 | 95 | ||
13 | 135 | 93 | ||
14 | 18 | 92 | ||
15 | 2 | 93 | ||
16 | 80 | 92 | ||
17 | 20 | 94 | ||
18 | 40 | 92 | ||
19 | 200 | 92 | ||
20 | 10 | 94 | ||
21 | 18 | 87 | ||
22 | No reaction | – | – | |
23 | No reaction | – | – |
a Yields refers to pure isolated product.
In all cases, the experimental results show that the reaction times are reduced and the yields increased under the sonication condition. Interestingly, this method is applicable for the N-formylation of steric hindered amines, such as dibenzylamine as well (Table 2, entry 18). Also, the N-formylation of para-nitroaniline was carried out in 95% yield by this method (Table 2, entry 3), while Choi and et al. were not able to do it, probably because the amino group in para-position has a very low basicity and hence it is not easily acylated [19].
The N-formylation of secondary amines needs longer reaction times than that of primary ones; thus, when a mixture of primary and secondary amines were exposed to formic acid (the ratio of amine to formic acid, 1:1), only the primary amine was formylated, which shows the excellent chemoselectivity of the reaction (Scheme 2).
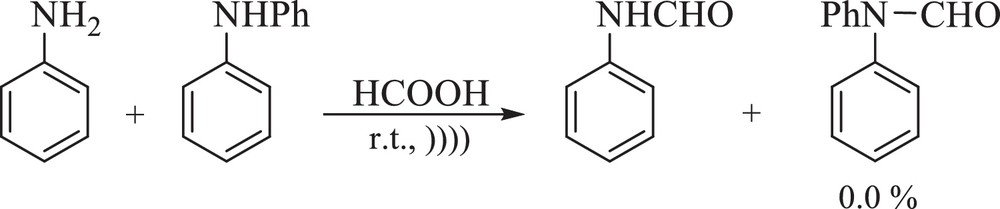
Chemoselective N-formylation of amines.
With those molecules containing both the hydroxyl and amino groups, only the N-formylated products were formed. So, O-formylation did not take place and the applied method shows a good chemoselectivity (Table 2, entries 4, 5, 22 and 23).
In a similar method, the amount of aniline was increased from 1.0 to 100.0 mmol and we found that the reaction proceeds successfully and that the N-phenylformamide product was obtained in high yield. So, the methodology is particularly suitable for conducting the reaction in a relatively larger scale without any difficulty, since it does not require a concomitant addition of bases, acids or catalysts.
Compared with the other literature works on the N-formylation of amines, the notable features of our method are:
- • the reaction system is clean and simple;
- • organic solvents are not needed;
- • catalysts are not needed;
- • the excess of formic acid can be easily recovered according to the literature;
- • the yields of the products are very high;
- • high reaction temperatures/pressures and prolonged reaction times are avoided;
- • no side product was observed under the reaction conditions; thus, this method did not require any further purification.
To the best of our knowledge, this new procedure provides the first example of an efficient ultrasound-promoted approach for the synthesis of N-formamides.
No reaction was observed when a mixture of aniline and formic acid was heated even after 5 h in the absence of ultrasound irradiation. In the presence of ultrasound irradiation, the solid amines were gradually dissolved in formic acid and then disappeared after about 10 s, while the classical conditions need longer reaction times. Ultrasounds provide an unusual mechanism due to the immense temperatures and pressures as well as the extraordinary heating rate generated by cavitation bubble collapse. The possible mechanism, which indicates the influence of ultrasound irradiation, was shown in Scheme 3 and has probably a concerted mechanism [24]. The breakdown of C–OH and N–H bonds in formic acid and amines facilitates the elimination of H2O and the final formamides will be produced. The energy needed by these cleavages and the formation of formamides is provided by the short-lived and localized hotspots that were generated during the cavitational collapse.

Mechanism of N-formylation of amines under ultrasonic irradiation.
The second principle of green chemistry states that the synthetic systems should be focused to improve the atom economy or atom efficiency by the employment of equimolar amounts of starting materials [15b]. Atom economy (AE) is a useful concept for estimating the environmental impacts of starting materials in a reaction to reach a desired product [25].
Scheme 4 and Table 3 show numbers of synthetic methods reported in the literature (equations (1–19)) for the N-formylation of amines as well as the present methodology (equation 20) in order to better understand the advantages of the proposed method.
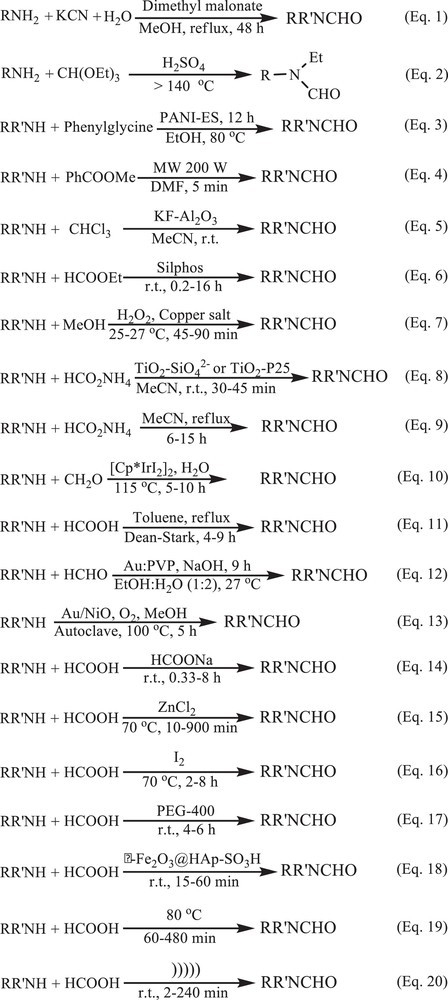
N-formylation of amines under different reaction conditions.
Atomic economy comparison of present methodology with other methods for the N-formylation of amines.
Entry | Equation | Product | Time | Yield (%)a | AE (%) |
1 | 3 | 12 h | 32 | 49.59 | |
6 | 75 min | 92 | 72.58 | ||
7 | 45 min | 75 | 76.10 | ||
8 | 30 minb, 45 minc | 99.2 | 77.56 | ||
9 | 11 h | 96 | 77.56 | ||
11 | 9 h | 99 | 87.05 | ||
12 | 8 h | 53 | 53.79 | ||
13 | 5 h | 72 | 59.62 | ||
14 | 1 h | 95 | 87.05 | ||
15 | 10 min | 96 | 87.05 | ||
17 | 6 h | 96 | 87.05 | ||
18 | 20 min | 97 | 87.05 | ||
19 | 25 min | 90 | 87.05 | ||
20 | 4 min | 97 | 87.05 | ||
2 | 3 | 24 h | NR | 75.09 | |
8 | 420 minb, 480 minc | 92b, 82.3c | 82.58 | ||
11 | 3 h | NR | 90.22 | ||
14 | 3.5 h | 92 | 90.22 | ||
15 | 720 min | 90 | 90.22 | ||
16 | 8 h | 86 | 90.22 | ||
17 | 6 h | 86 | 90.22 | ||
18 | 35 min | 94 | 90.22 | ||
19 | 480 min | 85 | 90.22 | ||
20 | 210 min | 95 | 90.22 | ||
3 | 1 | 48 h | 70 | 71.03 | |
3 | 12 h | 21 | 52.33 | ||
6 | 5 min | 95 | 74.58 | ||
7 | 75 min | 80 | 78.04 | ||
9 | 6 h | 88 | 79.41 | ||
14 | 2.5 h | 85 | 88.24 | ||
15 | 90 min | 90 | 88.24 | ||
16 | 2 h | 91 | 88.24 | ||
17 | 6 h | 42 | 88.24 | ||
18 | 40 min | 92 | 88.24 | ||
19 | 60 min | 90 | 88.24 | ||
20 | 20 min | 94 | 88.24 | ||
4 | 1 | 48 h | 55 | 67.63 | |
9 | 10 h | 95 | 76.67 | ||
13 | 5 h | 66 | 58.39 | ||
14 | 3 h | 86 | 86.48 | ||
15 | 360 min | 60 | 86.48 | ||
16 | 4 h | 71 | 86.48 | ||
18 | 30 min | 96 | 86.48 | ||
20 | 18 min | 87 | 86.48 |
a Yields are after work-up.
b Catalyzed by TiO2–SO42–.
c Catalyzed by TiO2–P25.
The following item are observed in most of the N-formylation procedures in the literature:
- • the use of flash chromatography or recrystallization for the purification of the products;
- • the use of organic solvents in the purification step;
- • the use of different reagents;
- • the use of harsh reaction conditions (relatively high temperature, hazards…);
- • the use of homogeneous/heterogeneous catalysts.
All the above items are money and time consuming; so the method we propose can be considered as a green protocol in which the reaction will take place in solvent- and catalyst-free conditions at room temperature.
The following comparison of different N-formylation methods can be deduced from the literature.
Disadvantages of equation (1) [26]: the use of a relatively volatile organic solvent (MeOH), in situ formation of HCN (highly toxic), long reaction times (48 h), relatively low yields, harsh reaction conditions (reflux), the use of dimethyl malonate (toxic and hazardous substance) and KCN as a formylating agent that cannot be easily recovered and recycled. The by-products of this reaction are formamidine, KOH, and NH3. Also, this method is not applicable for the N-formylation of hindered amines, such as dibenzylamine and obtaining the corresponding formamide failed even after 96 h under reflux.
Disadvantages of equation (2) [27]: the use of sulfuric acid (hazardous, toxic, extremely corrosive and homogeneous reagent which decomposes during work-up and cannot easily be recovered and recycled), harsh reaction conditions (> 140 °C), and tedious work-up (vacuum distillation). Also, this method is only limited to the formylation of aromatic amines. The by-product of this reaction is ethyl N-phenylformimidate.
Disadvantages of equation (3) [28]: the use of a catalyst and of a relatively volatile organic solvent (EtOH), long reaction times (12 h), relatively high temperature (80 °C), harsh reaction conditions (molecular oxygen) and low yields of the products, the use of amino acids as formylating agents for the in situ generation of CO which cannot be recovered, difficulties in preparation of the nanoporous polyaniline emeraldine salt and availability of reagents. The by-product of this reaction is N-benzylidenebenzyl- amine. No formylated products were obtained when para-nitroaniline and secondary amines were used.
Disadvantages of equation (4) [29]: the use of DMF (a high boiling point organic solvent which is soluble in both organic solvents and water, thus, the removing at the end of reaction is difficult), methyl benzoate (a very hazardous substance which cannot be easily recovered and recycled) and harsh reaction conditions (microwave 200 W, 200 °C). This method is not applicable for the N-formylation of hindered amines, such as dibenzylamine and obtaining the corresponding formamide failed even after 4 h at 200 °C. The by-product of this reaction is HN(CH3)2 and flash column chromatography is required for the purification of the products.
Disadvantages of equation (5) [30]: the use of a relatively volatile organic solvent (MeCN) and catalyst, the use of a toxic and volatile reagent (CHCl3) which is a hazardous substance, difficulties in the preparation of the catalyst and availability of reagents.
Disadvantages of equation (6) [31]: the use of large amounts of Silphos (a heterogeneous phosphine reagent) and ethyl formate as a formylating agent that cannot be easily recovered and recycled, difficulty in the reagent preparation, long reaction times (0.2–16 h) and by-product formation. No chemoselectivity was observed with this method.
Disadvantages of equation (7) [32]: the use of different basic copper hydroxyl salts as catalyst, difficulties in the preparation of the catalyst and availability of reagents, application of relatively volatile organic solvent (MeOH) and the use of H2O2 (corrosive and hazardous reagent). Flash column chromatography is required for the purification of products.
Disadvantages of equation (8) [33]: the use of relatively volatile organic solvent (MeCN) and catalyst, difficulties in the preparation of the catalyst and availability of reagents. No N-formylation occurs with diphenylamine in both catalytic conditions.
Disadvantages of equation (9) [11]: the use of relatively volatile organic solvent (MeCN), harsh reaction conditions (reflux) and long reaction times (6–15 h).
Disadvantages of equation (10) [34]: the use of an excess of para-formaldehyde as a formylating agent which cannot be easily recovered, difficulties in the preparation of the catalyst and availability of reagents, harsh reaction conditions (115 °C), long reaction times (5–10 h), and the use of a solvent. Flash chromatography is required for the purification of the products.
Disadvantages of equation (11) [19]: the use of toluene (low to moderate levels of toluene can cause tiredness, confusion, weakness, drunken-type actions, memory loss, nausea, loss of appetite, and hearing and color vision loss), harsh reaction conditions (reflux and use of Dean–Stark trap) and long reaction times (4–9 h). Column chromatography is required for the purification of the products. No N-formylation reaction was observed with para-nitroaniline.
Disadvantages of equation (12) [35]: the use of a relatively volatile organic solvent (EtOH), long reaction times (9 h), the use of base, basic conditions, and difficulties in the preparation of the catalyst as well as availability of reagents. No N-formylation reaction was observed with para-nitroaniline and N-methyl-ortho-toluidine. The by-product of this reaction is CO2 and only 2% of the target product was obtained from diphenylamine.
Disadvantages of equation (13) [36]: the use of a relatively volatile organic solvent (MeOH), high temperature (100 °C), harsh reaction conditions (the use of autoclave for the N-formylation and MeOH oxidation), long reaction times (5 h), difficulties in the preparation of the catalyst and availability of reagents, difficulties in the aerobic oxidation of MeOH and hard work-up (use of DMF and MeOH). The by-product of this reaction is imine and the method is limited only to the N-formylation of four amines.
Disadvantages of equation (14) [37]: the use of sodium formate as an additional substance and catalyst, long reaction times (0.33–8 h) and formation of impurities. Column chromatography is required for the purification of the products.
Disadvantages of equation (15) [38]: the use of ZnCl2 as a Lewis acid which is homogeneous in the reaction conditions and cannot easily be recovered and reused, relatively high temperature (70 °C) and formation of impurities. Column chromatography is required for the purification of the products.
Disadvantages of equation (16) [39]: the use of iodine (hazardous, toxic, corrosive and homogeneous reagent which cannot easily be recovered and reused), long reaction times (2–8 h), in situ formation of HI (harmful, toxic and corrosive), relatively high temperature (70 °C) and formation of impurities. Column chromatography is required for the purification of the products.
Disadvantages of equation (17) [40]: the use of polyethylene glycol, which is slightly hazardous in case of skin contact (irritant, permeator), eye contact (irritant) and ingestion and inhalation, long reaction times (4–6 h) and formation of impurities. Column chromatography is required for the purification of the products. This method is limited only to aromatic primary amines.
Disadvantages of equation (18) [41]: the use of expensive catalyst, difficulties in the preparation of the catalyst and availability of reagents and evolution of HCl (toxic, corrosive and homogeneous reagent) during the preparation of the catalyst.
Disadvantages of equation (19) [42]: the use of a relatively high temperature (80 °C) and formation of impurities. Column chromatography is required for the purification of the products.
Advantages of equation (20): the use of ultrasound irradiation as an alternative energy source represents an interesting, fast and clean synthetic route. The reactions will take place at room temperature with high atom economy, high yields and easy operations without the application of toxic organic solvents, bases, acids and expensive reagents or catalysts. Also, none of the above-mentioned disadvantages are observed. This green procedure does not require any purification techniques since no by-product was formed. The only by-product of this reaction is H2O, which is a green solvent. It is important to mention that the ultrasonic energy was successfully used to replace the conventional energy source (thermal conditions, 80 °C) [43] for the large-scale N-formylation of amines in short reaction times and higher purity at room temperature. As a general result, there was a significant increase of formamides yield for all derivatives in the ultrasonic-assisted reactor without formation of impurities (Table 2).
3 Conclusions
In conclusion, we have developed an economically and environmentally benign, fast, efficient and safe procedure for the N-formylation of amines under solvent- and catalyst-free conditions using ultrasound irradiation at room temperature. This method has the advantages of excellent yields and reaction rates, simple methodology, mild and green reaction conditions, cleaner reaction profiles, excellent chemoselectivity and easy work-up, in which the separation techniques are not necessary to get the pure compounds. The methodology is applicable to both the small and large scale N-formylation reactions and can also be used for the introduction of other amine protecting groups.
4 Experimental
4.1 Apparatus and analysis
All the reagents were purchased from Merck Chemicals and Aldrich and were used without further purification. The products were characterized by comparison of their physical and spectral data with authentic samples. The NMR spectra were recorded in DMSO and CDCl3. 1H NMR spectra were recorded on a Bruker Avance DRX 90, 300 and 500 MHz instruments. The chemical shifts (δ) are reported in ppm relative to the TMS as an internal standard and J values are given in Hz. 13C NMR spectra were recorded at 24.5 and 75 Hz. FT-IR (KBr) spectra were recorded on a PerkinElmer 781 spectrophotometer. Melting points were taken in open capillary tubes with a BUCHI 510 melting point apparatus and were uncorrected. The elemental analysis was performed using the Heraeus CHN-O-Rapid analyzer. TLC was performed on silica gel polygram SIL G/UV 254 plates. Ultrasonication was performed in a PARSONIC 2600s ultrasound cleaner with a frequency of 28 kHz and an output power of 50 W (Builtin heating, 20–70 °C thermostatically adjustable). The reaction flask was located at the maximum energy area in the ultrasound cleaner and the surface of the reactants was placed slightly lower than the level of the water. The reaction temperature was controlled by addition or removal of water from the ultrasonic bath. The temperature of the water bath was controlled at about 25–30 °C.
4.2 Caution
Although formic acid is often used as a source for a formyl group and hydride ion in organic synthesis, it is nonetheless a toxic material and appropriate safety precautions should be taken.
4.3 General experimental procedure for the synthesis of N-phenyl formamide (the mole ratio of amine to HCOOH, 1:1)
A mixture of formic acid (1 mM) and aniline (1 mM) was irradiated by ultrasound for 5 h at room temperature (Table 1). During stirring, a white crystalline solid was separated out. After completion (as monitored by TLC), the solid was separated and characterized by NMR, FT-IR, elemental analysis (CHN), and melting point measurement (Table 2, entry 1).
4.4 Typical procedure for the N-formylation of amines (the mole ratio of amine to HCOOH, 1:2.5)
A mixture of formic acid (2.5 mM) and amine (1 mM) was irradiated by ultrasound for an appropriate time at room temperature (Table 2). After completion (as monitored by TLC), H2O was added and the organic layer was extracted with EtOAc to afford the pure product (Table 2).
The physical data (mp, IR, NMR) of known compounds were found to be identical to those reported in the literature [19,26–42]. The formation of formamides was confirmed by IR spectra, which showed two characteristic peaks, one between 3300 and 3400 cm−1 (secondary NH) and the other between 1640 and 1680 cm−1 (N-formyl, CO). The 1H NMR spectra of the N-formamides showed one amide NH proton signal and another the signal of the aldehyde proton.
The elemental analysis (CHN), IR, 1H NMR and 13C NMR data of the unknown formamides (Table 2, entries 8 and 14) and 1H NMR data of the known compounds (Table 2, entries 1–7, 9–13 and 15–21) are given below.
4.5 Spectral data of some compounds
4.5.1 N-Phenyl formamide
Mp 46–48 °C (lit. [32] 46.3–47.8 °C); 1H NMR (90 MHz, CDCl3): δH 7.61–7.12 (m, 5H), 8.29 (s, 1H, cis), 8.66 (d, 1H, trans), 8.99 (brs, 1H, cis), 9.38 (brs, 1H, trans) (Table 2, entry 1).
4.5.2 N-(2-Chlorophenyl) formamide
Mp 76–80 °C (lit. [Aldrich] 76–80 °C); 1H NMR (90 MHz, acetone-d6): δH 6.95–8.36 (m, 5H), 8.93 (s, 1H) (Table 2, entry 2).
4.5.3 N-(4-Nitrophenyl) formamide
Mp 196–197 °C (lit. [41] 194–195 °C); 1H NMR (90 MHz, acetone-d6): δH 7.91 (d, J = 8.7 Hz, 2H), 8.25 (d, J = 9.0 Hz, 2H), 8.49 (s, 1H), 9.77 (s, 1H) (Table 2, entry 3).
4.5.4 N-(4-Chlorophenyl) formamide
Mp 100–102 °C (lit. [32] 99.8–101.1 °C); 1H NMR (300 MHz, CDCl3): δH 7.02–7.67 (m, 4H), 8.34 (s, 1H), 8.65 (s, 1H) (Table 2, entry 6).
4.5.5 N-(4-Bromophenyl) formamide
Mp 116–118 °C (lit. [Aldrich] 117–121 °C); 1H NMR (90 MHz, CDCl3): δH 6.31 (s, 1H), 6.52–7.46 (m, 4H), 8.61 (s, 1H) (Table 2, entry 7).
4.5.6 N-(2,5-dichlorophenyl) formamide
Mp 148–151 °C; FTIR (Nujol, cm−1): 3240, 2888, 1707, 1673, 1588, 1527, 1447, 1416, 1278, 1262, 1150, 1124, 1093, 1049, 906, 872, 829, 799, 736; 1H NMR (300 MHz, CDCl3): δH 9.70 (d, 1H), 8.52 (s, 1H), 7.75 (s, 1H), 7.32 (d, 1H, J = 8.3), 7.07 (d, 1H, J = 8.4); Anal. calcd for C7H5NOCl2: C, 44.24; H, 2.62; N, 7.33. Found: C, 43.93; H, 2.53; N, 7.41 (Table 2, entry 8).
4.5.7 N-(2-Methylphenyl) formamide
Mp 60–62 °C [37]; 1H NMR (90 MHz, acetone-d6): δH 8.41 (s, 1H), 7.95 (d, 1H), 6.98–7.29 (m, 4H), 2.29 (s, 3H) (Table 2, entry 9).
4.5.8 N-(4-Methylphenyl) formamide
Mp 52–54 °C (lit. [43] 50–54 °C); 1H NMR (300 MHz, CDCl3): δH 2.29 (S, 3H), 6.98–8.32 (m, 4H), 8.62 (s, 1H), 8.89 (s, 1H) (Table 2, entry 10).
4.5.9 N-(2,4-Dimethylphenyl) formamide
Mp 114–118 °C (lit. [Aldrich] 114–118 °C); FTIR (KBr, cm−1): 3167, 3073, 2923, 2877, 1686, 1656, 1608, 1510, 1469, 1375, 1314, 1256, 1217, 1121, 1036, 932, 900, 868, 828, 794, 721, 603; 1H NMR (300 MHz, DMSO-d6): δH 2.28 (s, 3H), 2.29 (s, 3H), 7.66 (d, 1H, J = 8.7), 8.43 (d, 1H, J = 15.5) (Table 2, entry 11).
4.5.10 N-(4-Acetylphenyl) formamide
Mp 104–107 °C [37,40]; 1H NMR (90 MHz, acetone-d6): δH 2.42 (s, 3H), 7.65 (d, J = 8.8 Hz, 2H), 7.86 (d, J = 9.0 Hz, 2H), 8.32 (s, 1H), 9.42 (s, 1H) (Table 2, entry 13).
4.5.11 N-(3-fluorophenyl) formamide
Mp 59–61 °C; FTIR (KBr, cm−1): 3279, 3221, 3163, 3092, 3055, 3010, 2881, 1693, 1675, 1608, 1560, 1486, 1452, 1400, 1339, 1319, 1283, 1260, 1180, 1151, 1125, 1069, 1000, 975, 911, 892, 818, 797, 736, 694, 655; 1H NMR (90 MHz, CDCl3): δH 7.42–8.33 (m, 4H), 8.89 (d, 1H, J = 10.6), 10.49 (s, 1H) (Table 2, entry 14).
4.5.12 4-Phenylpiperazine-1-carbaldehyde
Mp 82–84 °C; FTIR (KBr, cm−1): 2916, 2814, 1654, 1632, 1599, 1499, 1447, 1405, 1385, 1366, 1350, 1334, 1283, 1254, 1239, 1197, 1149, 1092, 1057, 1033, 1007, 916, 766, 693; 1H NMR (300 MHz, CDCl3): δH 10.35 (s, 1H), 8.55 (s, 1H), 6.92–7.33 (m, 5H, Ar–H), 3.72–3.16 (m, 8H); 13C NMR (500 MHz, CDCl3): δC 161.2, 151.4, 129.7, 121.4, 117.6, 50.9, 49.8, 46.0, 40.4 (Table 2, entry 16).
4.5.13 N-Benzyl formamide
Mp 60–62 °C (lit. [38] 60–62 °C); 1H NMR (90 MHz, CDCl3): δH 6.86–8.52 (m, 4H), 9.29 (s, 1H) (Table 2, entry 17).
4.5.14 N,N-Dibenzyl formamide
Mp 51–52 °C (lit. [43] 52 °C); 1H NMR (90 MHz, acetone-d6): δH 4.06 (s, 4H), 7.23 (s, 10 H), 8.13 (s, 1H) (Table 2, entry 18).
4.5.15 N,N-Diphenyl formamide
Mp 69–71 °C (lit. [41] 67–71 °C); 1H NMR (500 MHz, CDCl3): δH 7.20–7.45 (m, 10H), 8.6 9 (s, 1H) (Table 2, entry 19).
4.5.16 N-(1-Naphthyl) formamide
Mp 141–143 °C (lit. [44] 139 °C); 1H NMR (300 MHz, CDCl3): δH 7.27–8.06 (m, 7H), 8.65 (d, 1H), 8.83 (s, 1H) (Table 2, entry 20).
Acknowledgements
We are thankful to the University of Bu-Ali Sina for the support of this work.