1 Introduction
Since the discovery of the Heck reaction by Heck and Misoroki in the early 1970s, it has been recognized as one of the most important synthetic pathways to arylated olefins, important components for agrochemicals, pharmaceuticals, and cosmetics production [1–3]. In the Heck coupling reaction, usually aryl halides react with alkenes in the presence of palladium and of a suitable base in a single step under mild conditions [1]. Palladium catalysts used in the Heck reaction usually contain phosphorus ligands; because of palladium they have several setbacks associated with their air-sensitivity and rapid decomposition [3]. Moreover, phosphine ligands are expensive and difficult to prepare and this, coupled with the high cost of palladium metal, limits their industrial appeal. To circumvent these problems, significant research efforts are currently directed towards the design of cheaper metal sources than palladium and more stable catalysts [4,5].
In fact, the choice of a suitable supporting material is an important factor that may affect the performance of the Heck reaction [6]. Moreover, compared with supported palladium catalysts, supported transition metal (Cu, Co, Ni) catalysts for the Heck reaction are much less expensive [5]. Recently, several publications have appeared on the development of an immobilized Ni ion catalyst in Heck and Suzuki cross-coupling reactions [5,7,8].
Ionic liquid (IL) technology offers a new and environmentally benign approach toward modern synthetic chemistry [9–12]. ILs have interesting advantages, such as extremely low vapor pressure, excellent thermal stability, reusability, and talent to dissolve many organic and inorganic substrates [10–12]; nowadays, supported ILs containing different metal particles or ions have been used as catalysts in different reactions, such as the Heck reaction [13,14], hydrogenation [15–17]. Sasaki et al. prepared a Ni ion-containing IL salt and a Ni ion-containing immobilized IL on silica for the Suzuki reaction [7]. Han et al. synthesized a Ni2+-containing IL immobilized on silica to catalyze styrene oxidation with H2O2 for producing benzaldehyde [18].
Though ILs possessed such promising advantages, their widespread practical application was still hampered by several drawbacks:
- • high viscosity, which resulted in only a minor part of ionic liquids taking part in the catalyzed reaction;
- • homogeneous reaction, which was difficult for product separation and catalyst recovery;
- • consequently, high cost for the use of relatively large amounts of ILs [19,20].
Therefore, in order to solve these problems mentioned earlier, an immobilized IL catalyst combining the advantageous characteristics of ionic liquids, inorganic acids and solid acids had been proposed [21,22].
During the past decades, advances in nanoscience and nanotechnology have pushed forward the synthesis of functional magnetic nanoparticles (MNPs), which is one of the most active research areas in the field of advanced materials. MNPs that have unique magnetic properties and other functionalities have enabled a wide spectrum of applications [23]. Iron oxide magnetic nanoparticles (Fe3O4–MNPs) are approximately 20–30 nm in size and contain a single magnetic domain with a single magnetic moment and exhibit superparamagnetism [24]. Additionally, recent studies show that MNPs are excellent supports for catalysts [25,26]. The supported catalysts proved to be effective and easily separated from the reaction media by applying an external magnetic field.
In this paper, we synthesized a Ni2+-containing immobilized IL on magnetite Fe3O4 nanoparticles and carried out studies of its catalytic activity for the Heck coupling of aryl halides and olefins (Scheme 1). The heterogeneous catalyst could be recovered easily and reused many times without significant loss of its catalytic activity.

Heck reaction catalyzed by IL–Ni(II)–MNPs.
2 Results and discussion
2.1 Characterization of the prepared IL–Ni(II)–MNPs
Scheme 2 shows the sequence of events in the functionalization of MNPs with IL–Ni2+. In the first step, magnetite nanoparticles of 18–20 nm were prepared by the coprecipitation of iron(II) and iron(III) ions in an alkaline solution at 85 °C using the method described by Massart [27]. Then, 1-methyl-3-(3-trimethoxysilylpropyl) imidazolium chloride (IL) was prepared from the reaction of N-methyl imidazole with (3-chloropropyl) trimethoxysilane at 80 °C [28]. Then, IL was added to an acetonitrile solution of NiCl2 in a 250 mL round-bottom flask and refluxed for 24 h; IL–Ni(II) was then obtained. In a second step, the external surface of MNPs was coated with IL–Ni(II) to obtain IL–Ni(II)–MNPs (Scheme 2).

Preparation steps for fabricating IL–Ni(II)–functionalized magnetic Fe3O4 nanoparticles.
Fig. 1 shows the Fourier transform infrared (FT-IR) spectra of both the unfunctionalized and functionalized MNPs. The Fe–O stretching vibration near 580 cm−1, the O–H stretching vibration near 3432 cm−1, and the O–H deformed vibration near 1625 cm−1 were observed in both Fig. 2(a) and (b). The significant features observed in Fig. 1(b) are the appearance of the peaks at 1007 cm−1 (Si–O stretching), at 1612 cm−1 (CN stretching) and at 2800 cm−1 (–CH2 stretching). A peak at about 3390 cm−1 can be observed in Fig. 1(b), which indicates the formation of an hydrogen bond (C–H····Cl interaction) that has been observed in analogous compounds containing the 1-methyl-3-methylimidazolium cation [MmIM]+ and NiCl42− ([MmIM]2[NiCl4]) on the MNPs [18]. These results provided evidence that IL–Ni+2 was successfully attached to the surface of the Fe3O4 nanoparticles.

Compared FT-IR spectra of (a) magnetic nanoparticles (MNPs), (b) IL–Ni(II)–MNPs.
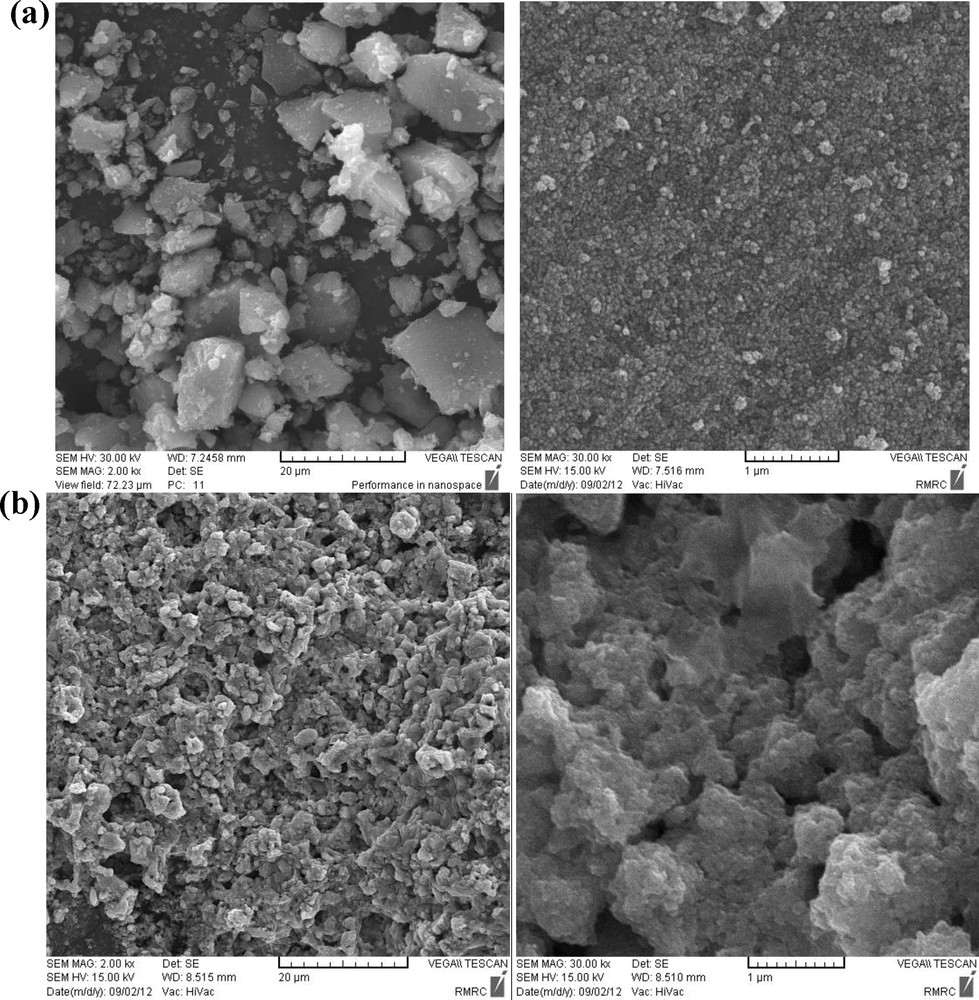
SEM image of (a) magnetic nanoparticles (MNPs), (b) IL–Ni(II)–MNPs.
The SEM image shows that magnetite (Fe3O4) nanoparticles have a mean diameter of about 18 nm and a nearly spherical shape (Fig. 2(a)). Fig. 2(b) shows that IL–Ni(II)–MNPs particles are larger, with a smoother surface, while silica is uniformly coated on them to form a silica shell.
Fig. 3 presents the X-ray diffraction patterns of the prepared MNPs and IL–Ni(II)–MNPs. The position and relative intensities of all the peaks confirm well with standard XRD pattern of Fe3O4 (JCPDS card No. 79-0417), indicating the retention of the crystalline cubic spinel structure of Fe3O4–MNPs. The XRD patterns of the particles show six characteristic peaks of a cubic iron oxide phrase (2θ = 30.35, 35.95, 43.45, 53.70, 57.25, 62.88, 71.37, 74.46). These are related to their corresponding indices (2 2 0), (3 1 1), (4 0 0), (3 3 1), (4 2 2), (3 3 3), (4 4 0) and (5 3 1), respectively [26]. It is implied that the resultant nanoparticles are pure Fe3O4 with a spinel structure. The crystal size of Fe3O4·MNPs nanoparticles can be determined from the XRD pattern by using the Debye–Scherrer equation (Fig. 3(a)):
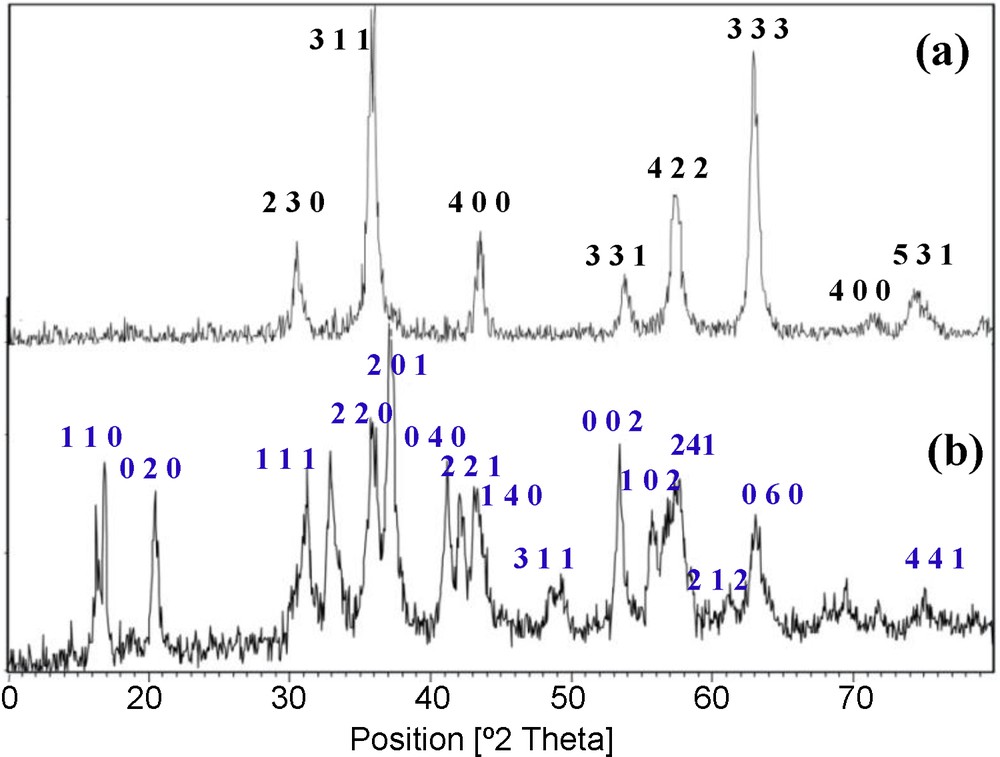
XRD patterns of (a) magnetic nanoparticles (MNPs), (b) IL–Ni(II)–MNPs.
Here, the (3 1 1) peak of the highest intensity was picked out to evaluate the particle diameter of the nanoparticles. Fe3O4–MNPs were calculated to be 18 nm. Fig. 3(b) depicts the XRD pattern of the prepared IL–Ni(II)–MNPs, with the diffraction peaks of both NiCl2 (JCPDS card No. 01-1143) and Fe3O4 (JCPDS card No. 79-0417).
To confirm the composition of the functionalized MNPs, we considered the EDS spectrum of IL–Ni(II)–MNPs displayed in Fig. 4. The EDS results indicated the presence of Ni, Cl, Si, and Fe in the composites, which indicates that the iron oxide nanoparticles have been coated by NiCl2. According to the above analysis, it could be concluded that IL–Ni(II)–MNPs have been successfully synthesized.
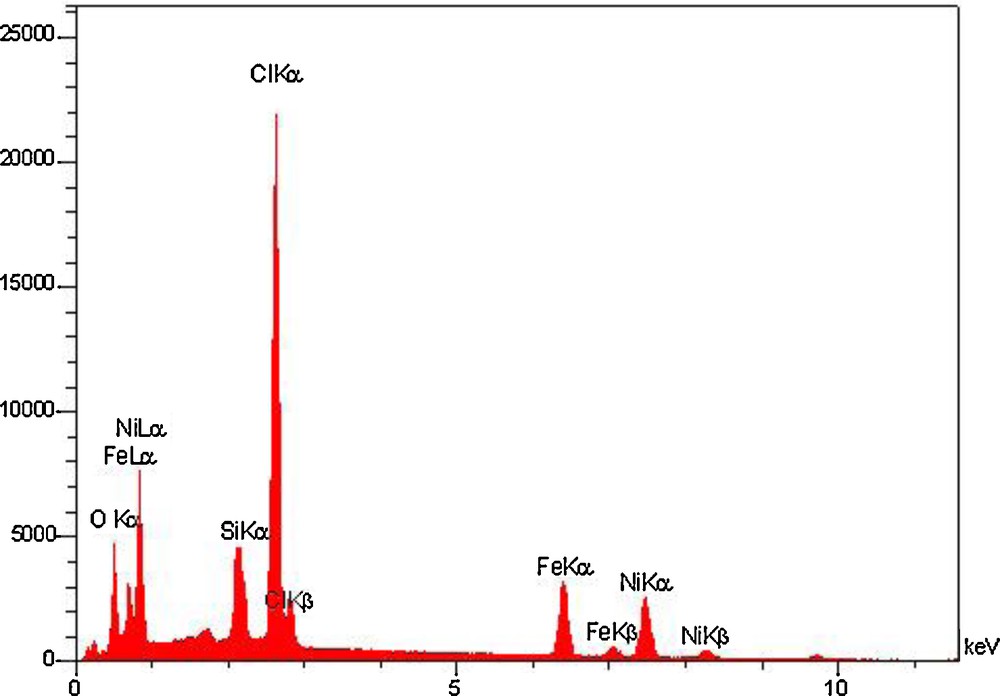
EDS spectrum of IL–Ni(II)–MNPs.
The magnetization curves of Fe3O4 nanoparticles and IL–Ni(II)–MNPs are shown in Fig. 5. The room temperature specific magnetization (M) versus applied magnetic field (H) curves of the samples indicate a saturation magnetization value (Ms) of 40.5 emu/g, lower than that of bare MNPs (60.1 emu/g) due to the coated shell. In Fig. 5, we can also see that both magnetization curves follow a Langevin behavior over the applied magnetic field and that coercivity (HC) could be ignored, which can be considered as superparamagnetism [26].
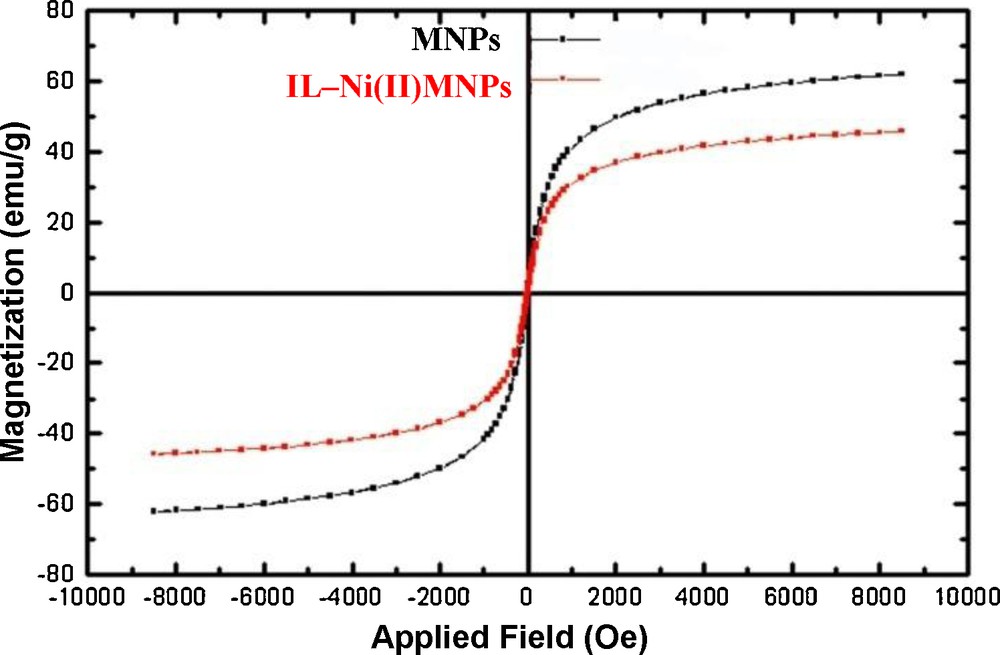
Magnetization curves for the prepared magnetic nanoparticles (MNPs) and IL–Ni(II)–MNPs at room temperature.
2.2 Evaluation of the catalytic activity of IL–Ni(II)–MNPs in the Heck reaction
The Heck reaction is one of the most important C–C bond formation reactions in organic synthesis. A variety of parameters, such as solvent, catalyst, base and temperature can influence the reactivity of the Heck coupling [29]. The benefits of N,N-dimethylformamide (DMF) as a solvent or solvating ligands are well known [29,30]. In this study, the IL–Ni(II)–MNPs were investigated as the catalysts in the model Heck coupling reaction of iodobenzene, and ethyl acrylate. This was performed in order to carry out the Heck vinylation reaction in a simple, efficient and inexpensive way. So, initially, the reactions were performed in DMF as a solvent with different bases at 90 °C in the presence of IL–Ni(II)–MNPs as the catalyst. A variety of bases, including Na2CO3, NaOAc, K2CO3 and Et3N, were employed to improve the yield for the synthesis of ethyl cinnamate. The results are presented in Table 1. An excellent yield was found with base Et3N (Table 1, entry 4) at 90 °C for 4 h. When the reaction was carried out in the presence of the catalyst (0.012 g), it led to the desired product in 80% yield in 4 h at 90 °C (Table 1, Entry 6). The catalytic activity of IL–Ni(II)–MNPs was evident when no product was obtained in the absence of the catalyst (Table 1, Entry 8).
Optimizing the Heck reaction conditions in the presence different bases and amounts of catalysta.
Entry | Catalyst (g) | Base | Time (h) | Yield (%)b |
1 | 0.010 | Na2CO3 | 4 | 30 |
2 | 0.010 | NaOAc | 4 | 39 |
3 | 0.010 | K2CO3 | 4 | 45 |
4 | 0.010 | Et3N | 4 | 70 |
5 | 0.08 | Et3N | 4 | 60 |
6 | 0.012 | Et3N | 4 | 80 |
7 | 0.014 | Et3N | 4 | 80 |
8 | – | Et3N | 24 | – |
a Iodobenzene (0.01 mol) ethyl acrylate (0.01 mol) and base (0.01 mol) in N,N-dimethylformamide (DMF) as a solvent at 90 °C.
b Determined by GC using mesitylene as the internal standard.
In the following study, we examined the effect of temperature on the reaction. We found out that, at lower temperatures, even if the reaction time was prolonged, only low yields were obtained, and that the higher the temperature, the better is the yield. The efficiency of the reaction is mainly affected by the amount of catalyst and the temperature. As indicated in Table 2, the best results have been obtained at 100 °C. This reaction can be considered as very mild for the Heck reactions, even for nickel catalysts.
Optimizing the Heck reaction conditions at different temperaturesa.
Entry | Temperature (°C) | Time (h) | Yield (%)b |
1 | 50 | 10 | 11 |
2 | 70 | 8 | 58 |
3 | 80 | 6 | 71 |
4 | 90 | 4 | 80 |
5 | 100 | 4 | 97 |
6 | 110 | 4 | 97 |
7 | 120 | 4 | 97 |
8 | 120 | 24 | – |
a Iodobenzene (0.01 mol) ethyl acrylate (0.01 mol), Et3N (0.01 mol) and 0.012 gr IL–Ni(II)–MNPs in N,N-dimethylformamide (DMF) as the solvent.
b Determined by GC using mesitylene as the internal standard.
Based on the above observations, we conducted the same reactions using various aryl halide and olefins in the presence of 0.012 g of IL–Ni(II)–MNPs under optimal conditions. As expected, satisfactory results were observed, and the results are summarized in Table 3. No catalytic activity was observed within 4 h with bromobenzene, but after 8 h, a substrate conversion of 28% was reported. However, some catalytic activity was observed in the coupling reactions of chlorobenzene with methyl acrylate, even after 10 h (conversion of 10%). This trend is consistent with the increasing strength of carbon–halide bonds from aryl iodide to aryl chloride [29] (Table 3, Entries 1–3).
Heck coupling reaction of aryl halide and olefins catalyzed by IL–Ni(II)–MNPsa.
Entry | Aryl halide | Olefins | Product | Time (h) | Yield (%)b |
1 | 4 | 97 | |||
2 | 4 | 98 | |||
3 | 4 | 88 | |||
4 | 8 | 28 | |||
5 | 10 | 10 |
a Aryl halide (0.01 mol), olefin (0.01 mol) and Et3N (0.01 mol) in N,N-dimethylformamide (DMF) solvent at 100 °C.
b Determined by GC using mesitylene as the internal standard.
On the other hand, aryl iodides are better reaction partners than bromides, which gave only poor conversions (less than 30%), even after extended reaction times (>24 h). Bases, such as Et3N are well suited, whereas inorganic bases, such as K2CO3 or NaOAc result in poor yields (Table 1, Entries 1–3).
The reusability is one of the important properties of this catalyst. After completion of the reaction, the catalyst was washed with DMF and acetone, dried and used directly with fresh substrates under identical conditions, without further purification. It was shown that the catalyst could be reused for the next cycle without any appreciable loss of its activity. Similarly, reusability studies for the sequential reaction were also carried out, and the catalyst was found to be reusable for five cycles (Fig. 6). As observed in Fig. 7, the XRD pattern of the recovered nanocatalyst was indexed according to the magnetite phase (JCPDS card No. 01-1143 for Fe3O4 and JCPDS card No. 75. 1609 for NiCl2), and so, there is no considerable change in its magnetic phase. Thus, the magnetite nanocatalyst is stable in the Heck reaction.
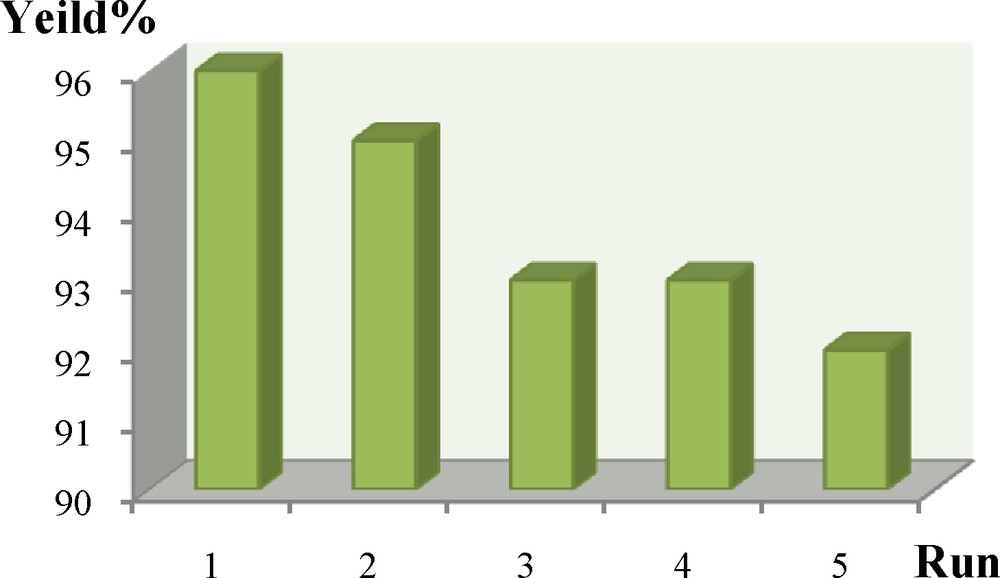
Recyclability of IL–Ni(II)–MNPs in the reaction of iodobenzene (0.01 mol) ethyl acrylate (0.01 mol), Et3N (0.01 mol), with N,N-dimethylformamide (DMF) as the solvent (GC yield).
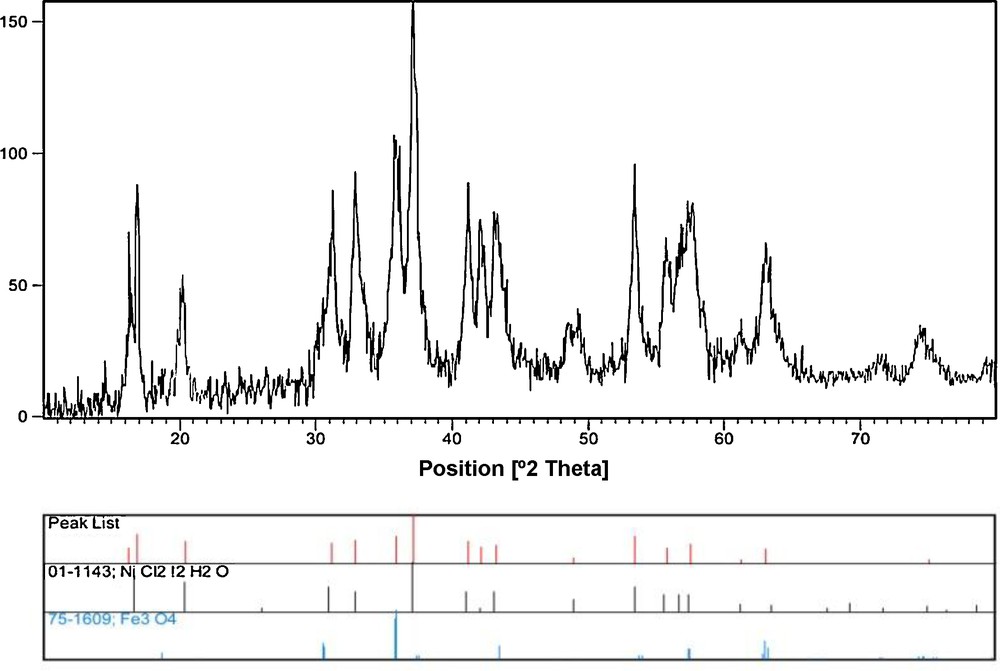
XRD patterns of the recovered IL–Ni(II)–MNPs after four recoveries.
In this simple and reliable protocol, we have reported the synthesis of a new catalyst for the Heck reaction. Additionally, the supported catalyst proved to be effective and easily separated from the reaction media by applying an external magnetic field. This heterogeneous catalyst could be recovered easily and reused many times without significant loss of its catalytic activity.
3 Conclusions
Ni2+-containing ILs immobilized on the MNPs have been synthesized and were used as a heterogeneous catalyst for the Heck reaction. IL–Ni(II)–MNPs displayed good catalytic activities under mild conditions towards iodobenzene and bromobenzene with olefins. This method offers several advantages, including high yield, short reaction time, simple work-up procedure, easiness of separation, and recyclability of the magnetic catalyst, as well as its ability to tolerate a wide variety of substitutions in the reagents.
4 Experimental method
High-purity chemical reagents were purchased from Merck and Sigma. All the materials were of commercial reagent grade. 1H NMR and 13C NMR spectra were recorded with a Bruker DRX-400 spectrometer at 400 and 100 MHz, respectively. FT-IR spectra were obtained with potassium bromide pellets in the 400–4000 cm−1 range with a PerkinElmer 550 spectrometer. The element analyses (C, H, N) were obtained with a Carlo Erba Model EA 1108 analyzer, and were carried out on a PerkinElmer 240c analyzer. Nanostructures were characterized using a Holland Philips Xpert X-ray powder diffraction (XRD) diffractometer (Cu Kα, radiation, λ = 0.154056 nm), at a scanning speed of 2°/min from 10° to 100° (2θ). Scanning electron microscope (SEM) images were recorded with a FEI Quanta 200 SEM operated at 20 kV accelerating voltage. The samples for SEM examination were prepared by spreading a small drop containing nanoparticles onto a silicon wafer and were dried almost completely in air at room temperature for 2 h, and then were transferred onto SEM conductive tapes. The transferred sample was coated with a thin layer of gold before measurement. Gas chromatography–mass spectroscopy (GC–MS) (Shimadzu + 17A, Ver 3) (column: DB-5ms, control mode: split, injection: 260 °C) was used to determine the yield of the Heck reactions.
4.1 Preparation of the magnetic Fe3O4 nanoparticles (MNPs)
Fe3O4–MNPs were prepared using the simple chemical coprecipitation method described in the literature [26]. Typically, 20 mmol of FeCl3·6H2O and 10 mmol of FeCl2·4H2O were dissolved in 75 mL of distilled water in a three-necked round-bottom flask (250 mL) under Ar atmosphere for 1 h. Thereafter, under rapid mechanical stirring, 10 mL of NaOH (10 M) were added into the solution within 30 min with vigorous mechanical stirring and ultrasound treatment under continuous Ar atmosphere bubbling. After being rapidly stirred for 1 h, the resultant black dispersion was heated to 85 °C for 1 h. The black precipitate formed was isolated by magnetic decantation, exhaustively washed with double-distilled water until neutrality, and further washed twice with ethanol and dried at 60 °C under vacuum.
4.2 Synthesis of 1- methyl -3-(3-trimethoxysilylpropyl)-1H-imidazol-3-ium chloride (IL)
1-Methylimidazole (13.6 mL, 0.17 mol) and (3-chloropropyl) trimethoxysilane (31 mL, 0.17 mol) were refluxed at 80 °C for 3 days in the absence of any catalyst and solvent under Ar atmosphere. The unreacted materials were washed with diethyl ether (3 × 8 mL). The diethyl ether was removed under reduced pressure at room temperature, and then was heated under high vacuum, to yield a yellowish viscous liquid. The isolated yield was 98% [28].
FT-IR (KBr, cm−1): 1656, 1612, 1584. 1H NMR (400 MHz, CDCl3): δH (ppm): 10.22 (broad, 1H, Are-H), 7.59 (1H, dd, J = 7.89 and 2.86 Hz, Are-H), 7.26 (1H, dd, J = 7.89 and 2.79 Hz, Are-H), 4.06 (2H, t, J = 7.25 Hz, –NCH2), 3.86 (3H, s, –NCH3), 3.30 (9H, s, OCH3), 1.74 (2H, tt, J = 7.14 Hz, –CH2), 0.37 (2H, t, J = 7.09 Hz, SiCH2). 13C NMR (100 MHz, CDCl3, TMS): δC = 138.13, 123.34, 121.58, 58.53, 51.66, 36.51, 24.32, 18.2, 7.03. Anal. calcd.: C, 48.45; H, 8.39; N, 8.69. Found: C, 48.35; H, 8.32; N, 8.79.
4.3 Synthesis of the Ni2+-containing IL
The Ni2+-containing IL was synthesized according to the method reported by other authors [18]. In the experiment, anhydrous NiCl2 (0.65 g, 0.05 mol), IL (3.23 g, 0.10 mol) and anhydrous acetonitrile (100 mL) were added into a 250-mL round-bottom flask; the mixture was refluxed at 363 K for 24 h under Ar atmosphere until a transparent blue solution was obtained. After cooling down, acetonitrile was evaporated under vacuum. A blue viscous liquid was obtained after the solution had been washed by toluene, which was dried under vacuum for 24 h to afford IL–Ni(II).
4.4 Modification of magnetic nanoparticles with IL–Ni2+ (IL–Ni(II)–MNPs)
Freshly prepared magnetite nanoparticles (2 g) were suspended in ethanol (95%, 250 mL), and sonicated for 60 min. The resulting suspension was mechanically stirred, followed by the addition of a solution of ethanol (95%, 100 mL) containing IL–Ni(II) (6 g) and concentrated ammonia (28%, 1 mL). Stirring under Ar was continued for 36 h. The modified magnetite nanoparticles were magnetically separated and washed three times with ethanol (95%, 50 mL) and dried under vacuum for 24 h.
4.5 Heck arylations catalyzed by IL–Ni2+–MNPs
In a typical Heck arylation reaction, iodobenzene (0.01 mol), ethyl acrylate (0.01 mol) triethylamine (0.01 mol) and DMF (20 mL) were added to the IL–Ni(II)–MNPs (0.01 g was dissolved in 5 mL of DMF) in a 50-mL flask equipped with a heating arrangement and a stirrer and the temperature was set at 100 °C. Samples were drawn at regular intervals and analyzed by GC to determine the percentage conversions. After the reaction was completed, the catalyst was separated by an external magnet.
Acknowledgements
We gratefully acknowledge the financial support from the Research Council of the University of Kashan through Grant NO (159198/VI).