1 Introduction
Replacement of conventional toxic and pollutant Brønsted and Lewis acid catalysts with environmentally benign and reusable solid heterogeneous catalysts is an active area of current research. Solid acid catalysts have advantages such as ease of product separation, recycling of the catalyst and environmental acceptability as compared to liquid acid catalysts [1]. Carbon-based solid acid catalysts have many advantages. They are insoluble in common organic solvents, cause low corrosion, and are environmentally benign. Also the products could be easily separated from the reaction mixture and the catalyst is recoverable without decreasing its activity [2]. Because of their strong protonic acid sites, they generally exhibit higher catalytic activity than conventional catalysts [3,4]. In recent years, much effort has been directed towards the development of new organic transformations under environmentally friendly conditions. In this context, organic reactions under solvent-free grinding conditions have proven to be useful to chemists for lab-scale as well as for large-scale conversions [5,6].
Among natural biopolymers, xanthan is the most abundant bacterial exopolysaccharide, being produced through fermentation [7]. It has been widely studied during the past several decades because it is a biodegradable material and a renewable resource. Its unique properties make it an attractive alternative to conventional organic or inorganic supports in catalytic applications [8]. It is very stable under a wide range of temperatures and pH values [9]. Recently, xanthan sulfuric acid (XSA) has emerged as a promising biopolymeric solid-support acid catalyst for acid catalysed reactions, such as the synthesis of 3,4-dihydropyrimidin-2(1H)-ones [10], thiadiazolo benzimidazoles [11], 4,4′-(arylmethylene)bis(1H-pyrazol-5-ols) [12], 14-aryl-14H-dibenzo[a,i]xanthene-8,13-diones [13], N-substituted pyrroles [14], α-amino phosphonates [15] and coumarins [16].
Indoles are convenient starting materials for the synthesis of a series of compounds with promising practical uses. Bis(indolyl)methanes and bis(indolyl)ethanes are important derivatives of indole. Bis(indolyl)methanes are the most active cruciferous substances for promoting beneficial oestrogen metabolism in women and men [17]. They are also effective in the prevention of cancer due to their ability to modulate certain cancer causing oestrogen metabolites [18]. Moreover, these compounds may normalize abnormal cell growth associated with cervical dysplasia. Several methods have been reported in the literature for the synthesis of bis(indolyl)methanes using heterogeneous catalysts including zeolite [19], ion exchange resins [20], PPh3HClO4 [21], silica-supported sodium hydrogen sulphate and amberlyst-15 [22], H3PO4SiO2 [23], metal hydrogen sulphates [24], P2O5SiO2 [25], KHSO4SiO2 [26], SiO2POCl2 [27], LiHSO4SiO2 [28], SiO2ClCH2COOH [29], Ce(OTf)4 [30], Zeokarb-225 [31], Nano-TiO2 [32], PEGSO3H [33], silica sulfuric acid [34] and cellulose sulfuric acid [35]. Many of the methods used have disadvantages such as long reaction periods, use of hazardous solvents, use of expensive reagents or preformed reagents, poor yields of products, and the fact that they are not environmentally friendly. For these reasons, there is a great effort to replace the conventional catalysts by eco-friendly and green-process catalysts.
It is, therefore, of interest to examine the behaviour of XSA as a catalyst for the synthesis of bis(indolyl)methanes. To the best of our knowledge, there is no report on the synthesis of bis(indolyl)methanes using XSA as a catalyst. Herein, we describe the use of XSA as a highly efficient, biodegradable and recyclable solid acid catalyst for the synthesis of bis(indolyl)methanes by the reaction of indole with different heterocyclic/aromatic aldehydes under solvent-free conditions in excellent yields. The compounds were identified on the basis of spectral data. The structure and morphology of the catalyst was established for the first time with the help of powder X-ray diffraction (XRD), scanning electron microscopy (SEM), energy dispersive X-ray analysis (EDX), differential scanning calorimetry (DSC), and thermo-gravimetric analysis (TGA).
2 Results and discussion
2.1 Catalyst characterization
2.1.1 FT-IR spectrum of xanthan sulfuric acid (XSA)
The FT-IR spectrum of the catalyst showed absorption bands at 1263 and 1374 cm−1 for the symmetric and asymmetric stretching vibrations of the SO2 group, respectively. Another stretching absorption band at 599 to 659 cm−1 was attributed to the SO functional group. The spectrum also showed a strong broad band for OH stretching absorption in the range from 3261 to 3467 cm−1 [36].
2.1.2 Powder X-ray diffraction (XRD) analysis of free xanthan and XSA
The structure of the prepared catalyst was identified by powder XRD. X-ray patterns of the free xanthan and catalyst xanthan–OSO3H were recorded in the 2θ = 20–79° range. The XRD of free xanthan showed no considerable peaks of crystallinity (Fig. 1a) [37]. However, the XRD of XSA (Fig. 1b) showed small amount of crystallinity with the characteristic peaks of SO3 groups.

Powder XRD pattern of (a) free xanthan and (b) fresh catalyst.
2.1.3 SEM-EDX analysis of the catalyst
To study the surface morphology of the catalyst, SEM micrographs of the catalyst were employed. The SEM images of the catalyst (Fig. 2) clearly show the strand-like nature of the sample.

The SEM images of freshly synthesized catalyst (XSA) at different magnifications.
Further, EDX analysis (Fig. 3) of the catalyst showed the presence of S, O and C elements, suggesting formation of the expected catalytic system.

EDX analysis of the catalyst (XSA).
2.1.4 DSC-TGA of the catalyst (XSA)
DSC analysis (Fig. 4) of the catalyst (XSA) was performed in the temperature range between 20 and 300 °C at a constant heating rate of 10 °C/min under nitrogen atmosphere. The DSC curve shows an irreversible endothermic transition in the region between 100 and 136 °C, which may be due to the loss of a water molecule from the polymer matrix. This analysis also shows that the catalyst is stable up to 270 °C, after which it shows some exothermic transition.

DSC of the catalyst (XSA).
TG analysis (Fig. 5) of the catalyst (XSA) was performed in the temperature range between 20 and 500 °C at a constant heating rate of 20 °C/min under nitrogen atmosphere. The TG curve shows the weight loss at 61.5 °C, which is due to the loss of water molecules trapped in the support matrix. The weight loss of 42% at a temperature of 283.9 °C may be due to the decomposition of xanthan. Further, weight loss of 23.7% at a temperature of 373.7 °C may be due to the decomposition of the SO3 group attached to the support [38].

TGA of catalyst (XSA).
2.2 Synthesis of bis(indolyl)methanes
In the present study, a series of bis(indolyl)methanes were prepared by the condensation of indole (1) and different aldehydes (2a-h) in the presence of XSA (Scheme 1). The reactions proceeded smoothly to afford bis(indolyl)methanes (3a–h) in good to excellent yields. The results are summarized in Table 1.

Synthesis of bis(indolyl)methanes using XSA under grinding condition.
XSA catalyzed synthesis of bis(indolyl)methanes.
Entry | Aldehyde | Product | Timea (min) | Yieldb (%) |
1 | 25 | 92 | ||
2 | c | 20 | 86 | |
3 | 30 | 88 | ||
4 | 25 | 90 | ||
5 | 35 | 86 | ||
6 | 30 | 84 | ||
7 | 32 | 86 | ||
8 | 35 | 90 |
a Reaction progress monitored by TLC.
b Isolated yield.
c New compounds.
Since 3-position of indole is the preferred site for electrophilic reactions, substitution occurred exclusively at this position, and N-substituted products were not detected in the reaction mixture.
The structural assignment of all the compounds (3a–h) was done by collection of elemental and spectroscopic data (IR, NMR and MS). The IR spectrum of the newly synthesized compound (3a) exhibited strong absorption bands at 3467 and 3400 cm−1 for two NH groups of the indole moiety. The proton nuclear magnetic resonance spectroscopy exhibited sharp singlets at δ = 1.98 and 5.87 for three methyl and one methine protons (Ha), respectively. Fifteen aromatic protons (five protons of a phenyl group of pyrazole moiety and ten protons of indole unit) were discernible as multiplet at δ 6.80–7.57. Two NH protons of the indole moiety were present as singlet at δ = 10.47. The 13C NMR spectrum showed signals at δ = 13.4 and 29.6 for methyl and methine carbon, respectively. Other carbon signals appeared at their appropriate positions and will be discussed in the experimental section. Further, evidence of the formation of 3a was obtained by mass spectrometry, which showed a molecular ion peak at m/z = 436.
A plausible mechanism for the formation of 3a in the presence of XSA is displayed in Scheme 2.
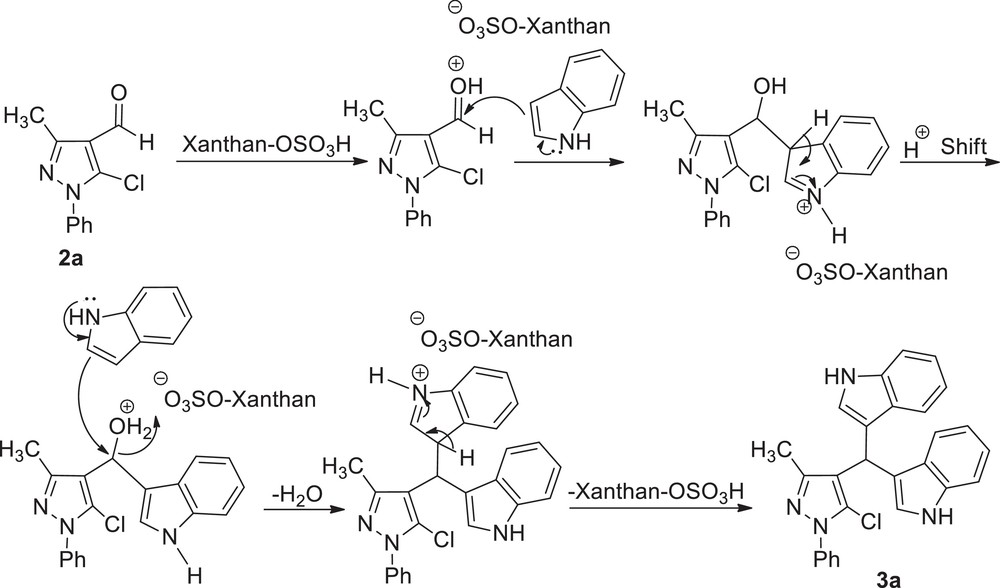
Proposed mechanism for the formation of 3a.
2.3 Catalytic reaction
To study the appropriate reaction conditions for the synthesis of bis(indolyl)methanes, the condensation of aldehyde (2a) and indole (1) was initially examined in the presence of chlorosulfonic acid. It was observed that chlorosulfonic acid promoted the reaction with impure products (28%) with generation of harmful wastes, which pose environmental problems. When the reaction was carried out in the presence of xanthan gum, a highly sticky orange reaction mixture was obtained. Though the reaction mixture indicated the formation of a product, no pure product could be isolated. When XSA was used as a solid acid catalyst, the product was obtained in excellent yields (84–92%) in a shorter time period (20–35 min). The reaction of 5-chloro-3-methyl-1-phenylpyrazole-4-carboxaldehyde (1 mmol) with indole (2 mmol) in the presence of XSA was used as a model reaction.
2.3.1 Effect of different catalysts
In order to emphasize the efficiency of XSA in comparison with other catalysts, the model reaction was carried out with various catalysts such as l-proline, Zn (l-proline)2, zinc acetate, sulfamic acid, sulfuric acid, benzenesulfonic acid, NiCl2, and AlCl3 (Table 2). It was observed that l-proline and Zn (l-proline)2 could not catalyse the reaction (Table 2, entries 1, 2). When the reaction was performed with sulfamic acid, it was completed after a long time period with impure products (Table 2, entry 3). In a comparative study, the model reaction was also carried out in sulfuric acid and benzenesulfonic acid. The result showed that the reaction took a longer time for completion (more than 4 h) and that the products were obtained in trace amounts (Table 2, entries 4, 5). Using zinc acetate, the reaction was completed in a relatively shorter time period, but with a moderate yield of the product (Table 2, entry 6). With NiCl2 and AlCl3, only trace amounts of the products were obtained (Table 2, entries 7, 8). When XSA was used as a catalyst, the reaction was completed in a shorter reaction time, with an excellent yield of the product (Table 2, entry 9).
The screening of different catalysts on the model reaction under solvent-free condition.
Entrya | Catalyst | Timeb | Yieldc (%) |
1 | l-proline | – | – |
2 | Zn (l-proline)2 | – | – |
3 | Sulfamic acid | 2 h | 60 |
4 | Sulfuric acid | 5 h | Trace |
5 | Benzenesulfonic acid | 4.5 h | Trace |
6 | Zinc acetate | 40 min | 72 |
7 | NiCl2 | 1 h | Trace |
8 | AlCl3 | 50 min | Trace |
9 | XSA | 25 min | 92 |
a Reaction of 5-chloro-3-methyl-1-phenylpyrazole-4-carboxaldehyde (1 mmol) with indole (2 mmol) in the presence of 200 mg of catalyst.
b Reaction progress monitored by TLC.
c Isolated yield.
2.3.2 Effect of solvents
In order to study the solvent's effect, the model reaction was carried out in different protic and aprotic solvents such as CH3COOH, MeOH, EtOH, (CH3)2CHOH, CH2Cl2, and CH3CN. When the reaction was performed in EtOH, MeOH, and (CH3)2CHOH, lower yields of the product were obtained after a longer time period (Table 3, entries 4, 5, 6). Using CH3COOH, the reaction was completed in a relatively shorter time period, with impure products (Table 3, entry 3). In CH2Cl2 and CH3CN, again only trace amounts of the product were obtained (Table 3, entries 7, 8). When the reaction was carried out under grinding conditions, both the yield and the reaction time were significantly improved (Table 3, entry 1). In comparison with the solvent-free grinding condition, the model reaction was also performed under solvent-free heating. It was observed that the reaction was completed in a relatively shorter time period, but the product was obtained in lower yields (Table 3, entry 2).
Comparative study for the synthesis of bis(indolyl)methanes using solution conditions versus the solvent-free method.
Entrya | Solvent | Temperature | Timeb | Yieldc (%) |
1 | Grinding | RT | 25 min | 92 |
2 | No solventd | 70 °C | 35 min | 48 |
3 | CH3COOH | RT | 3 h | Mixture |
4 | EtOH | RT | 7 h | 72 |
5 | MeOH | RT | 8 h | 64 |
6 | (CH3)2CHOH | RT | 23 h | 54 |
7 | CH2Cl2 | RT | 43 h | 36 |
8 | CH3CN | RT | 46 h | 28 |
2.3.3 Loading of the catalyst
The model reaction was carried out using different amounts (60, 100, 140, 180, 200 and 220 mg) of XSA for the optimization of the catalyst. It was found that 200 mg of catalyst is sufficient for the fruitful completion of the reaction. It was found that when the reaction was carried out without the use of a catalyst, it required longer reaction time for completion with the formation of by-products (Table 4, entry 7). As the amount of the catalyst increased, a reduction in time period and an enhancement in the product yield was observed. A maximum yield of the product (92%) and a shorter time period (25 min) for the completion of reaction were observed when the amount of the catalyst was 200 mg. Further, an increase in the amount of the catalyst reduced the time period, but the yield of the product was lowered (Table 4, entry 6).
2.4 Recycling study of catalyst
Using the model reaction, indole, aldehyde and XSA were ground together in a mortar with a pestle at room temperature for a specified time period. On completion of reaction, methanol was added and the reaction mixture filtered. The recovered catalyst was washed with methanol thoroughly (4 × 10 mL), dried in an oven at 80 °C for 2 h and used for the subsequent cycles. The same procedure was applied for all recycling studies. The results (Fig. 6) revealed that the catalyst exhibited a good catalytic activity up to four consecutive cycles.

Recyclability of the catalytic system.
The recovered catalyst was identified by powder XRD, SEM, and EDX analysis. It was observed that the peaks remained the same (Fig. 7) and also that no change in the morphology of the catalyst (Fig. 8) was observed as compared to the fresh catalyst, suggesting that the catalyst was active up to four cycles. EDX analysis (Fig. 9) also showed that no leaching due to hydrolysis of the supported xanthan hydrogen sulphate occurred up to four runs. Furthermore, acid–base titration was done to confirm the number of acidic groups and it was found to be 0.58 mequiv/g for the recovered catalyst (after four runs) against 0.62 mequiv/g for the fresh catalyst [36].

Powder XRD pattern of a recovered catalyst after four runs.
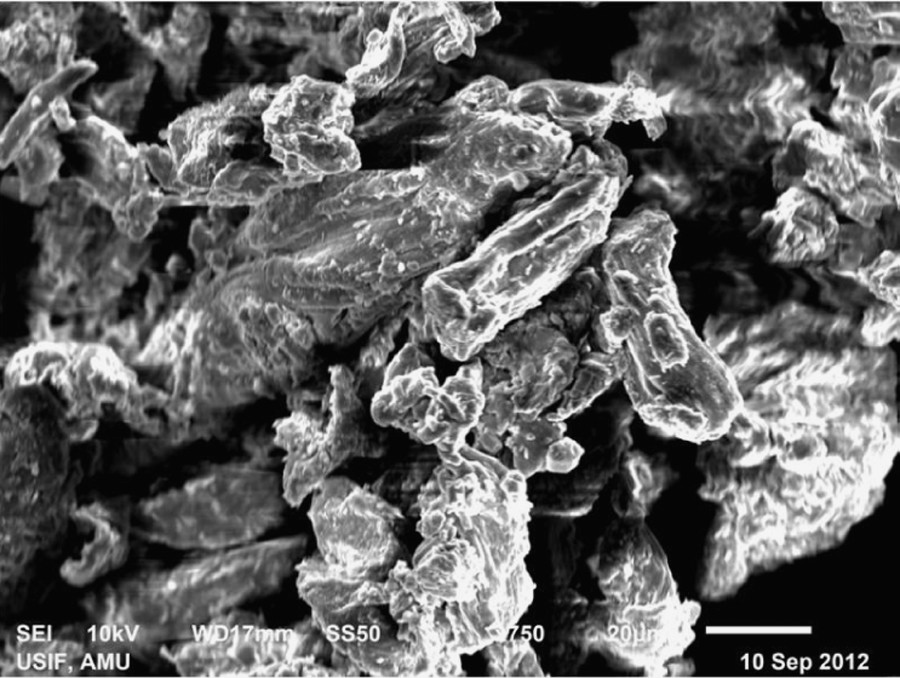
SEM image of the recovered catalyst after four runs.
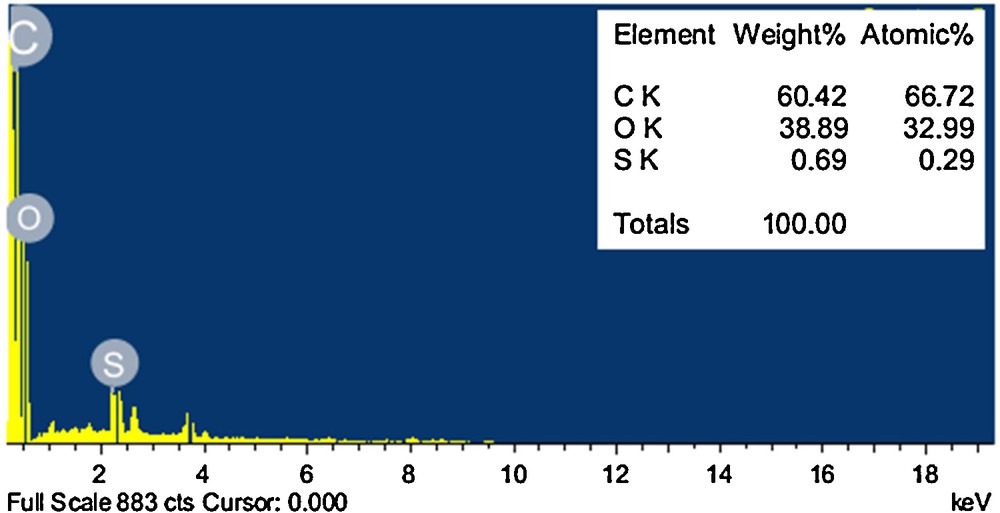
EDX analysis of the recovered catalyst after four runs.
3 Experimental
3.1 General
Melting points of all synthesized compounds were taken in a Riechert Thermover instrument and are uncorrected. The IR spectra (KBr) were recorded on PerkinElmer RXI spectrometer. 1H NMR and 13C NMR spectra were recorded with a Bruker DRX-300 and Bruker Avance II 400 spectrometer using tetramethylsilane (TMS) as an internal standard and DMSO-d6/CDCl3 as a solvent. ESI–MS were recorded with a Quattro II (ESI) spectrometer. Elemental analyses (C, H and N) were conducted using the Elemental vario EL III elemental analyser and their results were found to be in agreement with the calculated values. 5-Chloro-3-methyl-1-phenylpyrazole-4-carboxaldehyde, 5-azido-3-methyl-1-phenylpyrazole-4-carboxaldehyde and 3-formylchromone were synthesized by the reported procedures [40–42]. Xanthan was purchased from Otto Chemie Pvt Ltd. Other chemicals were of commercial grade and used without further purification. The homogeneity of the compounds was checked by thin layer chromatography (TLC) on glass plates coated with silica gel G254 (E. Merck) a using chloroform–methanol (3:1) mixture as the mobile phase and visualized using iodine vapours. X-ray diffractograms (XRD) of the catalyst were recorded in the 2θ range between 20 and 80° with a scan rate of 4° min−1 on a Rigaku Minifax X-ray diffractometer with Ni-filtered Cu Kα radiation at a wavelength of 1.54060 Å. The SEM-EDX characterization of the catalyst was performed on a JEOL JSM-6510 scanning electron microscope equipped with an energy dispersive X-ray spectrometer operating at 20 kV. DSC data were obtained with DSC-60 and TGA with DTG-60H (simultaneous DTA-TG apparatus) Shimadzu instruments.
3.2 Preparation of the catalyst
The catalyst (XSA) was synthesized by the reported procedure [36]. To a magnetically stirred mixture of xanthan (5.0 g) in CHCl3 (15 mL), chlorosulfonic acid (1.00 g) was added dropwise at 0 °C during 2 h. HCl gas was removed from the reaction vessel immediately. After completion of the addition, the mixture was stirred for 3 h. Then, the mixture was filtered and washed with methanol (25 mL) and dried at room temperature to obtain XSA as a white powder (5.30 g).
3.3 General procedure for the synthesis of bis(indolyl)methanes
A mixture of indole (2 mmol), aldehyde (1 mmol) and XSA (200 mg) were ground together in a mortar with a pestle at room temperature for a specified period. On completion of reaction (as monitored by TLC), methanol (20 mL) was added and the reaction mixture filtered. The catalyst was washed with methanol several times. The solvent was evaporated under reduced pressure to obtain the product in almost pure form, which was further purified by crystallization from suitable solvents.
3.4 Spectroscopic data
3.4.1 4-[Bis(indol-3-yl)methyl]-5-chloro-3-methyl-1-phenylpyrazole (3a)
Mp: 210–212 °C. IR (KBr) νmax/cm−1: 3467 and 3400 (NH), 1596 and 1541(CC). 1H NMR (DMSO-d6, 300 MHz): δ 1.98 (s, 3H, CH3), 5.87 (s, 1H, Ha), 6.80–7.57 (m, 13Ar–H + 2Hb), 10.47 (s, 2H, NH). 13C NMR (300 MHz): δ 148.58, 138.35, 136.75, 128.78, 127.48, 126.64, 124.37, 123.64, 121.00, 118.92, 118.34, 115.40, 111.41, 29.66, 13.45. ESI–MS: M+436 (m/z). Anal. calcd for C27H21N4Cl: C, 74.38; H, 4.82; N, 12.84. Found: C, 73.37; H, 4.85, N, 12.82.
3.4.2 4-[Bis(indol-3-yl)methyl]-5-azido-3-methyl-1-phenylpyrazole (3b)
Mp: 220–222 °C. IR (KBr) νmax/cm−1: 3421 and 3374 (NH), 1542 and 1521 (CC). 1H NMR (DMSO-d6, 300 MHz): δ1.98 (s, 3H, CH3), 5.87 (s, 1H, Ha), 6.80–7.57 (13 Ar–H + 2Hb), 10.47 (s, 2H, NH). 13C NMR (300 MHz): δ146.58, 134.75, 133.95, 126.36, 125.24, 124.28, 123.80, 122.32, 120.86, 118.35, 117.66, 114.82, 110.35, 30.72, 14.26. ESI–MS: M+443 (m/z) Anal. calcd. For C27H21N7: C, 73.20; H, 4.74; N, 22.12. Found: C, 73.24; H, 4.77; N, 22.09.
3.4.3 3-[Bis(indol-3-yl)methyl]chromone (3c)
Mp: 236–240 °C. IR (KBr) νmax/cm−1: 3389 and 3226 (NH), 1635 (CO), 1571 (CC). 1H NMR (DMSO-d6, 300 MHz): δ 6.08 (s, 1H, Ha), 6.88–8.13 (m, 9Ar–H + 2Hb + Hc+Hd + He), 10.69 (s, 2H, NH). 13C NMR (300 MHz): δ176.25, 156.08, 154.53, 136.94, 133.34, 126.75, 125.52, 123.77, 123.69, 121.13, 118.96, 118.11, 115.94, 111.47, 29.39. ESI–MS: M+390 (m/z). Anal. calcd. for C26H18N2O: C, 80.07; H, 4.62; N, 7.18. Found: C, 80.11; H, 4.65; N, 7.21.
3.4.4 3-[Bis(indol-3-yl)methyl]-6-methylchromone (3d)
Mp: 244–246 °C. IR (KBr) νmax/cm−1: 3289 and 3146 (NH), 1646 (CO), 1619 (CC). 1H NMR (DMSO-d6, 300 MHz): δ 2.35 (s, 3H, CH3), 6.42(s, 1H, Ha), 6.89–8.74 (m, 9Ar–H + 2Hb+Hc + Hd+He), 10.27 (s, 1H, NH), 11.90 (s, 1H, NH). 13C NMR (300 MHz): δ 175.64, 162.23, 156.34, 151.26, 135.71, 133.82, 128.62, 127.52, 124.46, 122.85, 121.24, 117.88, 116.74, 115.56, 113.60, 20.82. ESI–MS: M+404 (m/z). Anal. calcd. for C27H20N2O2: C, 80.27; H, 4.95; N, 6.93. Found: C, 80.29; H, 4.92; N, 6.89.
3.4.5 3-[Bis(indol-3-yl)methyl]-6-chlorochromone (3e)
Mp: 240–242 °C. IR (KBr) νmax/cm−1: 3304 (NH), m 1640 (CO), 1604 (CC). 1H NMR (DMSO-d6, 300 MHz): δ 6.47 (s, 1H, Ha), 7.03–8.23 (m, 9Ar-H + 2Hb + Hc + Hd + He), 12.04 (s, 2H, NH). 13C NMR (300 MHz): δ 178.46, 165.32, 158.21, 150.60, 134.17, 128.26, 126.81, 124.24, 123.87, 121.66, 118.23, 117.65, 115.82, 112.28, 25.62. ESI–MS: M+ 424 (m/z). Anal. calcd. for C26H17N2O2Cl: C, 73.59; H, 4.01; N, 6.60. Found: C, 73.62; H, 4.03; N, 6.63.
3.4.6 3, 3′-(Thiophen-2-ylmethylene)bis(indole) (3f)
Mp: 148–150 °C. IR (KBr) νmax/cm−1: 3404 and 3384 (NH), 1545 (CC). 1H NMR (DMSO-d6, 300 MHz): δ 6.09 (s, 1H, Ha), 6.87–7.37 (m, 11Ar–H + 2Hb), 10.49 (s, 2H, NH). 13C NMR (300 MHz): δ 149.35, 136.61, 126.37, 126.13, 124.55, 123.26, 120.95, 118.30, 118.25, 111.37, 35.14. ESI–MS: M+ 328 (m/z). Anal. calcd. for C21H16N2S: C, 76.83; H, 4.88; N, 8.53. Found: C, 76.80; H, 4.83; N, 8.61.
3.4.7 Tris(1H-indol-3-yl)methane (3 g)
Mp: 230–232 °C. IR (KBr) νmax/cm−1: 3396 and 3054 (NH), 1553 (CC). 1H NMR (DMSO-d6, 300 MHz): δ6.03 (s, 1H, Ha), 6.81–8.04 (m, 12Ar-H + 3Hb), 10.46 (s, 3H, NH). 13C NMR (300 MHz): δ136.75, 126.91, 123.36, 120.70, 118.49, 111.23, 31.16. ESI–MS: M+361 (m/z). Anal. calcd. for C25H19N3: C, 83.17; H, 5.26; N, 11.63. Found: C, 83.14; H, 5.21; N, 11.61.
3.4.8 3,3′-Bis(indol-3-yl)phenylmethanes (3 h)
Mp: 124–126 °C. IR (KBr) νmax/cm−1: 3404 and 3194 (NH), 1603 (CC). 1H NMR (DMSO-d6, 300 MHz): δ5.88 (s, 1H, Ha), 6.65–7.39 (m, 13Ar–H + 2Hb), 7.89 (s, 2H, NH). 13C NMR (300 MHz): δ 144.68, 136.75, 128.41, 127.89, 126.74, 125.72, 123.70, 120.92, 119.21, 118.31, 118.21, 111.30, 39.89. ESI–MS: M+322 (m/z). Anal. calcd. for C23H18N2: C, 85.79; H, 5.59; N, 8.69. Found: C, 85.82; H, 5.53; N, 8.64.
4 Conclusion
In summary, we have developed a simple, novel and efficient protocol for the synthesis of bis(indolyl)methanes using XSA as biodegradable, reusable, heterogeneous catalyst under solvent-free conditions at room temperature. The advantages of the present methodology are excellent yields of products, inexpensive catalyst, energy-sustainable protocol, shorter time period, simple operational procedure and purification of products merely by recrystallization.
Acknowledgements
UGC, New Delhi, is gratefully acknowledged for awarding research fellowship to ST and Special Assistance Programme Scheme (Departmental Research Support Phase 1). The authors are thankful to the Centre of Nanotechnology, Department of Applied Physics and University Sophisticated Instrument Facility (USIF), AMU, Aligarh for providing powder X-Ray diffractometer and SEM-EDX facilities. The authors would also like to thank SAIF, Punjab University, Chandigarh for providing the spectral data.