1 Introduction
Metal nanoparticles (NPs) are important due to their remarkable properties and potential application in a variety of areas, such as catalysis, sensors, electronics, and optics [1]. The last decade has witnessed tremendous growth in the field of nanoscience and nanotechnology. The easy accessibility to nanoparticles has prompted investigations on their application in catalysis. Several reports showed a remarkable level of their performance as catalysts in terms of selectivity, reactivity, and improved yields of product [2]. Metal nanoparticles, such as nickel, palladium, gold, platinum, etc. have properties that may well markedly differ from those of their bulk metals [3]. Recently, it has been proved that Ni(0) nanoparticles as catalysts offer great opportunities for a wide range of applications in organic synthesis [4], such as Suzuki–Miyaura cross-coupling [5], hydrogenation [6], oxidative addition [7], Stille coupling [8], Knoevenagel condensation [9], Hantzsch condensation [10], etc. However, more attention has been paid to nickel catalysts because nickel is comparatively cheap and more environmentally friendly. Green chemical reactions have become a prominent issue in recent decades. Reactions in aqueous media are considerably more safe, non-toxic, environmentally friendly, and inexpensive. Organic reaction in water has attracted a great deal of interest in both academic and industrial research because of environmental concerns [11].
Pyran derivatives are of considerable interest in industry as well as in academia owing to their promising biological and medicinal activity, such as analgesic, anti-tumour, anti-cancer, anti-inflammatory properties and also serve as potential inhibitors of human Chk1 kinase (Fig. 1) [12]. Furthermore, they are also found in applications as pharmaceutical ingredient and biological agrochemicals [13].

An inhibitor of human Chk 1 Kinase.
Considerable effort has been made towards the synthesis of pyran annulated heterocyclic derivatives due to their wide applications. Recently, a few methods have been reported by using various catalysts [14–19]. Although most of these processes offer distinct advantages, at the same time they suffer from certain drawbacks, such as longer reaction time, unsatisfactory yields, high costs, harsh reaction conditions, use of stoichiometric amounts as well as of environmentally toxic catalysts, and also lack of recyclability [20].
Ultrasound irradiation has been established as an efficient technique in synthetic organic chemistry. The ultrasonic irradiation with its advantages, i.e. convenient operation, mild reaction conditions, short reaction time and high efficiency, has become particularly popular in recent times [21]. In order to enlarge the application of ultrasound irradiation to the synthesis of heterocyclic compounds, we wish to report a general, efficient and eco-friendly procedure for the synthesis of pyran derivatives.
2 Experimental
2.1 Materials and methods
The melting points were determined in open capillaries and uncorrected. IR spectra were recorded with a PerkinElmer Spectrum BX FT-IR apparatus (υmax in cm−1) on KBr disks. 1H NMR and 13C NMR (400 MHz and 100 MHz, respectively) spectra were recorded on a Bruker Avance II-400 spectrometer in CDCl3 and DMSO- d6 (chemical shifts in δ with TMS as the internal standard). Mass spectra were recorded on Waters ZQ-2695. Transmission electron microscope (TEM) spectra were recorded with a JEOL JSM 100CX device. Scanning electron microscope (SEM) measurements were recorded with a JEOL JSM-6360. XRD spectra were recorded with a Bruker D8 XRD instrument SWAX. CHN were recorded using a PerkinElmer 2400, Series II, CHN-OS analyser. Silica gel G (E-mark, India) was used for TLC. Hexane refers to the fraction boiling between 60 °C and 80 °C. The ultrasonication reaction was carried out in JAC 1500 (made in Korea).
2.2 X-ray crystallography
The X-ray diffraction data were collected at 296 K using the Mo Kα radiation (λ = 0.71073 Å) using a Bruker Nonius SMART APEX II CCD diffractometer equipped with a graphite monochromator. SMART software was used for data collection and also for indexing the reflections and determining the unit cell parameters; the collected data were integrated using SAINT software. The structures were solved by direct methods and refined by full-matrix least-squares calculations using SHELXTL software. All non-H atoms were refined using the anisotropic approximation (CCDC 836545).
2.3 General procedure for the synthesis of pyran derivatives
A mixture of carbonyl compounds 1 (1 mmol), malononitrile 2 (1 mmol) and activated C–H compounds 3–5 (1 mmol) was poured into a round-bottom flask; nickel nanoparticles (10 mol%), water (2 mL) or water–ethanol mixture (1:1) (2 mL) [in the case of 4-hydroxy coumarin 5] were added to it and irradiated in ultrasound for a duration as mentioned in Table 1. After completion (TLC), the reaction mixture was cooled, filtered and the solvent was removed under reduced pressure. The residue was extracted with ethyl acetate (3 × 10 mL) and the combined organic extract was washed with water (3 × 10 mL), brine (10 mL), and dried over anhydrous Na2SO4. After removing the solvent, the crude product was purified by column chromatography over silica gel (60–120 mesh) using hexane–ethyl acetate as an eluent to afford the pure products.
Synthesis of pyran derivatives under ultrasonic irradiation in water.
Entry | Ar (1) | Product | Molecular formula of product | Time (min) | Yield (%)a |
1 | Benzaldehyde | 6a | C20H16N4O | 7 | 90 |
2 | 4-Methyl benzaldehyde | 6b | C21H18N4O | 14 | 88 |
3 | 4-Fluoro benzaldehyde | 6c | C20H15 FN4O | 11 | 87 |
4 | 4-Nitro benzaldehyde | 6d | C20H15N5O3 | 10 | 89 |
5 | 4-Bromo benzaldehyde | 6e | C20H15 BrN4O | 12 | 90 |
6 | 4-Chloro benzaldehyde | 6f | C20H15ClN4O | 14 | 87 |
7 | 4-Cyano benzaldehyde | 6g | C21H15N5O | 5 | 85 |
8 | 3-Nitro benzaldehyde | 6h | C20H15N5O3 | 10 | 88 |
9 | 2-Chloro benzaldehyde | 6i | C20H15 ClN4O | 12 | 87 |
10 | Benzaldehyde | 7a | C18H18N2O2 | 10 | 86 |
11 | 4-Methyl benzaldehyde | 7b | C19H20N2O2 | 15 | 83 |
12 | 4-Methoxybenzaldehyde | 7c | C19H20N2O3 | 15 | 88 |
13 | 4-Fluoro benzaldehyde | 7d | C18H17FN2O2 | 14 | 85 |
14 | 4-Nitro benzaldehyde | 7e | C18H17N3O4 | 5 | 90 |
15 | 4-Bromo benzaldehyde | 7f | C18H17BrN2O2 | 5 | 86 |
16 | N, N-dimethylaminobenzaldehyde | 7g | C20H23N3O2 | 14 | 88 |
17 | 4-Chloro benzaldehyde | 7h | C18H17ClN2O2 | 15 | 91 |
18 | 4-Cyano benzaldehyde | 7i | C19H17N3O2 | 15 | 88 |
19 | 2-Chloro benzaldehyde | 7j | C18H17ClN2O2 | 10 | 90 |
20 | 1-Napthaldehyde | 7k | C22H20N2O2 | 12 | 87 |
21 | Isatin | 7l | C19H17N3O3 | 10 | 89 |
22 | Benzaldehyde | 8a | C19H12N2O3 | 13 | 89 |
23 | 4-Methyl benzaldehyde | 8b | C20H14N2O3 | 14 | 86 |
24 | 4-Nitro benzaldehyde | 8c | C19H11N3O5 | 10 | 87 |
25 | 4-Methoxybenzaldehyde | 8d | C20H14N2O4 | 15 | 86 |
26 | 4-Cyano benzaldehyde | 8e | C20H11N3O3 | 6 | 87 |
27 | Isatin | 8f | C20H11N3O4 | 10 | 88 |
a Isolated yields.
2.4 Physical and spectroscopic data for the selected compound
2.4.1 4-Methyl benzaldehyde (6b)
IR (KBr): 3475, 3326, 2196, 1656 cm−1. 1H NMR (CDCl3 + DMSO-d6, 400 MHz): δ = 1.78 (s, 3H), 2.25 (s, 3H), 4.50 (s, 1H), 6.22 (s, 2H), 7.35 (t, J = 7.8 Hz, 5H), 7.65 (d, J = 8.4 Hz, 4H). 13 C NMR (CDCl3 + DMSO-d6, 100 MHz): δ = 12.4, 20.6, 36.6, 60.1, 98.1, 119.7, 120.2, 125.8, 127.3, 128.7, 128.8, 136.2, 137.3, 139.5, 143.6, 145.6, 158.9. ESI–MS m/z 343 [M + H]+. Anal calcd for C21H18N4O: C, 73.67; H, 5.30; N, 16.36. Found: C, 73.75; H, 5.36; N, 16.48 (Table 1, entry 2).
2.4.2 2-Chloro benzaldehyde (7k)
IR (KBr): 3317, 2380, 2183, 1659, 1593 cm−1. 1H NMR (CDCl3, 400 MHz): δ = 8.31(d, J = 8.4 Hz, 1H), 7.76 (d, J = 8.0 Hz, 1H), 7.65 (d, J = 8.4 Hz, 1H), 7.51 (t, J = 7.6 Hz, 1H), 7.42 (t, J = 7.4 Hz, 1H), 7.33 (t, J = 7.8 Hz, 1H), 7.17 (t, J = 7.2 Hz, 1H), 5.19 (s, 1H), 4.46 (s, 2H), 2.50–2.39 (m, 2H), 2.18–2.07 (m, 2H), 1.05 (s, 3H), 0.99 (s, 3H). ESI–MS m/z 345 [M + H]+. Anal calcd for C22H20N2O2: C, 76.72; H, 5.85; N, 8.13. Found: C, 76.64; H, 5.91; N, 7.96 (Table 1, entry 20).
2.4.3 4-Nitro benzaldehyde (8c)
IR (KBr): 3476, 2196, 1719, 1613 cm−1. 1H NMR (CDCl3 + DMSO-d6, 400 MHz): δ = 8.07–7.23 (m, 8H), 4.60 (s, 1H), 3.11 (brs, 2H). 13C NMR (CDCl3 + DMSO-d6, 100 MHz): δ = 164.9, 163.3, 159.1, 157.3, 154.7, 151.7, 137.8, 133.7, 129.5, 128.5, 127.7, 123.6, 121.4, 117.7, 107.9, 62.5, 41.9. ESI–MS m/z 362 [M + H]+. Anal calcd for: C19H11N3O5: C, 63.16; H, 3.07; N, 11.63. Found: C, 62.87; H, 2.93; N, 11.54 (Table 1, entry 24).
3 Result and discussion
As part of our continued activities in this area [22], we are reporting for the first time a simple and efficient method for the synthesis of pyran derivatives by a one-pot multi-component reaction, involving C–H activated compounds, (3–5), malononitrile (2), aryl aldehydes, using novel and reusable Ni nanoparticles in water under ultrasound irradiation. We started this synthesis by examining the reaction of pyrazolone (1 mmol), malonitrile (1 mmol) and benzaldehyde (1 mmol) in the presence of nickel nanoparticles in water under ultrasonic irradiation. The formation of the compound was confirmed by spectral data. IR spectra of compound 6b showed absorption peaks at 3475, 3326 cm−1, which are due to the NH stretching of the NH2 group; the peak at 2196 cm−1 corresponds to CN stretching. In the 1H NMR spectrum, the two methyl groups present in pyrazolone and in the benzene ring appeared as a singlet at δ 1.78 and 2.25, respectively. The CH (methine proton) was observed as a singlet at δ 4.50, while two protons corresponding to NH2 appear as a singlet at δ 6.22. The nine aromatic protons appeared at δ 7.35 and δ 7.65. The structures of all the compounds were confirmed from spectroscopic data and found to be comparable in all respect with those of an authentic sample. We have investigated the effect of different ultrasonic irradiations, such as low-, medium- and high-frequency ones, at different temperatures on this reaction (Fig. 2). From Fig. 2, it is evident that the maximum yield was obtained by using a higher irradiation level at 70 °C. To find out the effect of ultrasound irradiation on this reaction, the model reaction was performed under the same conditions in the absence of ultrasound irradiation. It was observed that the reaction time increased and that product yield decreased considerably. Thus, ultrasounds have a beneficial effect on the synthesis of pyran derivatives.
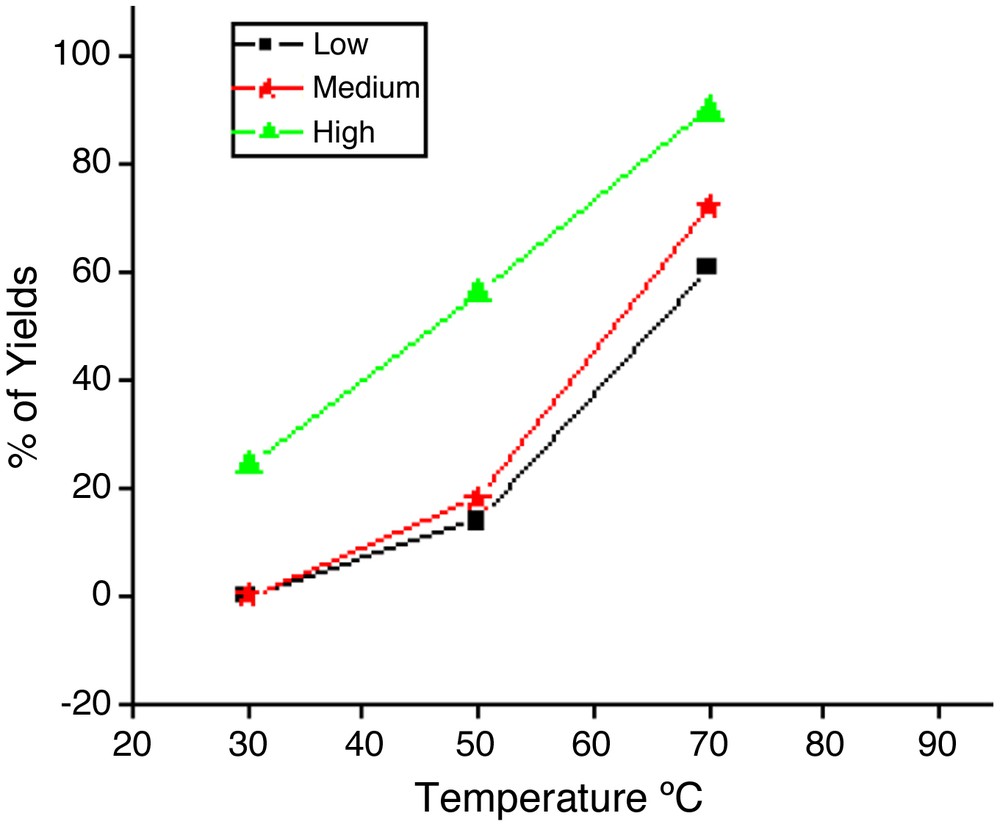
Optimization of the reaction conditions. Reagents and conditions: pyrazolone (1 mmol), malononitrile (1 mmol), benzaldehyde (1 mmol), Ni NPs (l0 mol%), water (2 mL), ultrasound irradiation.
To investigate the advantages and limitations of our methodology, other aldehydes having electron-donating or electron-withdrawing substituents in the aromatic ring were subsequently used and the results are reported in Table 1. From these results, we could conclude that the whole reaction proceeds smoothly to afford the corresponding products in satisfactory yield. Aromatic aldehydes carrying electron-donating or electron-withdrawing substituents showed similar reaction behaviour and reacted efficiently to yield the desired products.
Nickel nanoparticles were prepared by the reduction of nickel chloride hexahydrate (NiCl2·6H2O) with hydrazine hydride in an oil micro-emulsion of water/CTAB (cetyltrimethyl ammonium bromide)/1-pentanol at 80 °C for 2 h. CTAB is used as a stabilizer and hydrazine hydrate as a reducing agent. In this work, the Ni nanoparticles were prepared from the reported micro-emulsion method [23]. The reduction reaction could be expressed as:
2Ni2+ + N2H4 + 4HO− → 2Ni + N2 + 4H2O |
The growth of Ni nanoparticles was completed after 2 h. The particle size and structure of the resultant Ni nanoparticles have been characterized by transmission electron microscopy (TEM) (Fig. 3), scanning electron microscopy (SEM) (Fig. 4), and X-ray diffraction (XRD) (Fig. 5) analysis. XRD analysis further supported this result. Three peaks for Ni nanoparticles at 2θ = 44.78o, 52.20o, 76.68o, correspond to the (111), (200), (220) lattice planes, so that the resultant particles are pure elemental Ni nanoparticles with a face-centred cubic (FCC) structure. No obvious peak of nickel oxide or hydroxide was detected. The result is in complete agreement with the reported data [24].

TEM image of nickel nanoparticles (a) before use (b) after reuse (four times).
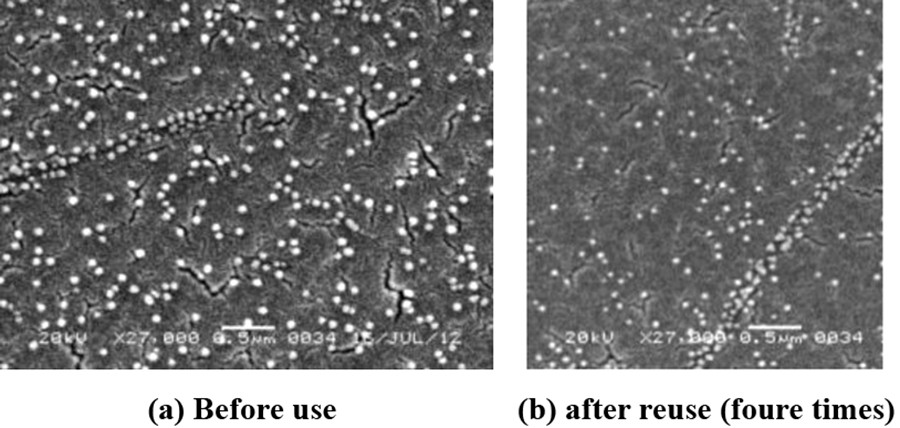
SEM image of nickel nanoparticles (a) before use (b) after reuse (four times).

Powder XRD pattern with characteristic dihedral angels at 44.78°, 52.20° and 76.68° corresponding to Ni(0) nanoparticles.
Our attention moved onto investigating the effect of solvent on the product yield. The reaction was carried out in various solvents, like water, DCM, THF, acetonitrile, and toluene. Significant improvement was achieved in DCM and acetonitrile, but the best result was observed when we used water as a reaction medium (Fig. 6). In case of 4-hydroxy coumarin, the reaction was sluggish; this may be due to the insolubility of 4-hydroxy coumarin in water. In order to increase the solubility, we have used a 1:1 water–ethanol mixture.

Effect of solvent on the rate of the reaction and the yield of the product. Reagent and condition: pyrazolone (1 mmol), malononitrile (1 mmol), benzaldehyde (1 mmol), Ni NPs (l0 mol%), ultrasound irradiation, solvent (2 mL).
We have studied the effect of the catalyst concentration on the model reaction. We have varied the concentration of catalyst from 2 to 10 mol%. The result revealed that when the reaction was carried out in the presence of 2 and 4 mol% of catalyst, it gave lower yields of the product. Notable improvement was achieved by using 6 and 8 mol% of catalyst, but the best result was obtained when we used 10 mol% of the catalyst. Further increase in the catalyst concentration did not improve the yield (Fig. 7). We also carried out the reaction without any catalyst, but the desired product was obtained in poor yields (28%).

Effect of catalyst loading on the reaction yield.
To further expand the scope of the present method, we investigated this one-pot reaction involving dimedone 4 and 4-hydroxy coumarin 5. To our delight, under the above optimized conditions, the reactions proceeded smoothly and a variety of the desired pyran derivatives were obtained in good yields (Scheme 1 and Table 1). This protocol could be applied to the aryl aldehydes with either electron-withdrawing groups or electron-donating groups. As it can be seen from Table 1, the electronic effect of the substituted benzaldehyde has an insignificant impact on the conversion. The reaction was also carried out with 1, 2-diketone (isatin) and the product was obtained in good yields. The feasibility of employing aliphatic aldehydes instead of aryl aldehydes in the reaction was also investigated. Unfortunately, aliphatic aldehydes could not afford the expected product: the starting material remained as such.

Furthermore, a crystal of 8b was obtained by careful recrystallization from a solution of DMF, and the structure was unambiguously confirmed by X-ray crystallography. Fig. 8 shows the ORTEP diagram of compound 8b with probability ellipsoids representation (30%).
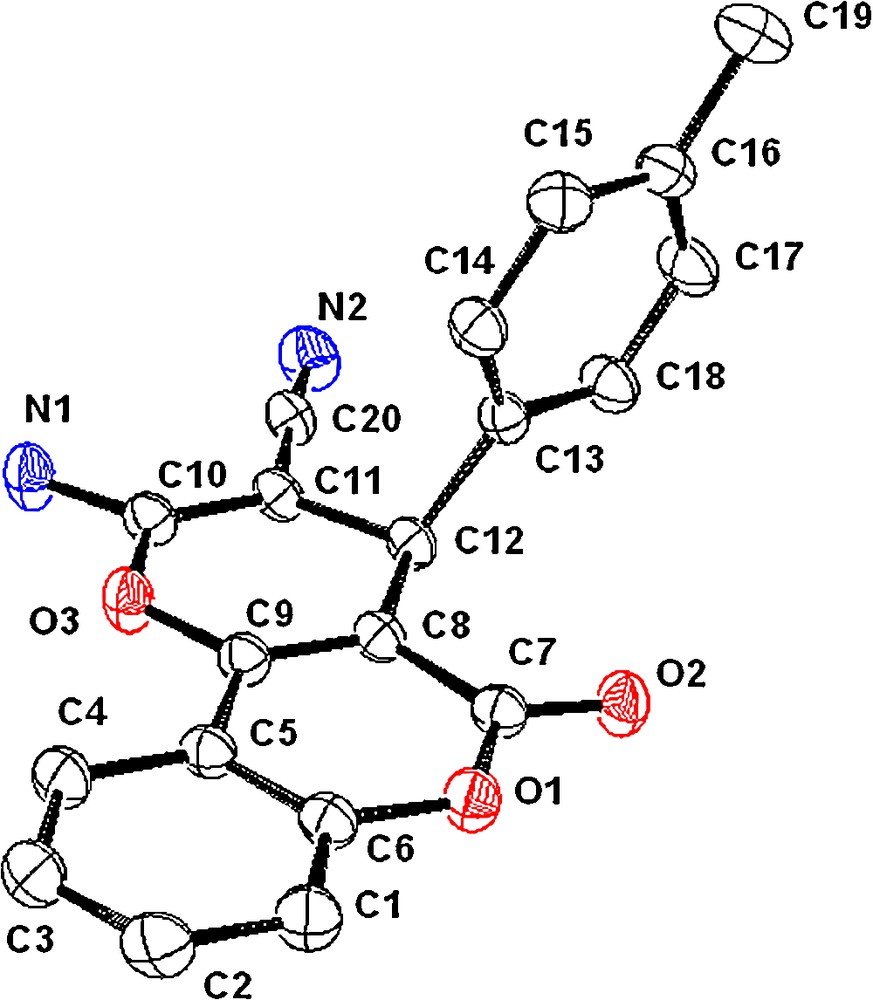
Showing the ORTEP diagram of 8b with ellipsoids presented at the 30% probability level; hydrogen atoms are removed for clarity (CCDC 836545).
A plausible mechanism for the reaction is depicted in Scheme 2. Nickel nanoparticles increase the electrophilicity of carbonyl carbon of C–H activated compounds as well as of aryl aldehydes. First, aryl aldehydes and malononitrile formed the Knoevenagel product 9. The methine carbon of 10 is activated by nanoparticles and it reacts with the C–H-activated compound in a Michael fashion, giving intermediate 11. Intermediate 11 undergoes intramolecular cyclization followed by tautomerization, affording the pyran derivatives 6.
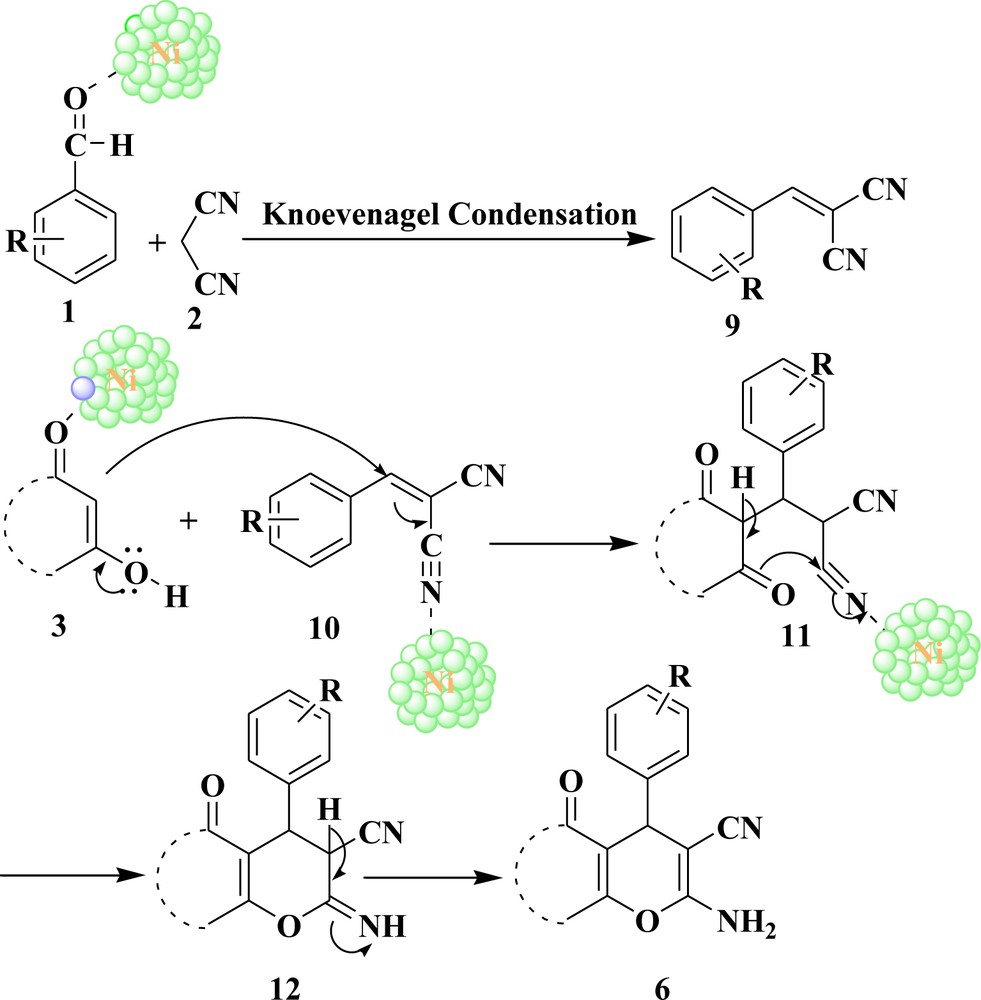
Plausible reaction mechanism.
For practical application of this heterogeneous system, recovery and reusability of the catalyst were very important factors. It was observed that, in our present work, Ni nanoparticles could be recycled and reused for another four consecutive runs without any noticeable change of the product yield. The recovered Ni NPs were analysed by TEM and SEM imaging (Figs. 3b and 4b). It was observed that the catalyst could be used up to four runs without any loss of efficiency. After four runs, the reactivity decreases, possibly due to the agglomeration of nanoparticles on each exposure. After each run, the reaction mixture was centrifuged at 4000 rpm for 3 min and filtered. The residue was washed with deionized water and dried. The recovered nanoparticles were used again for another set of reactions (Fig. 9).
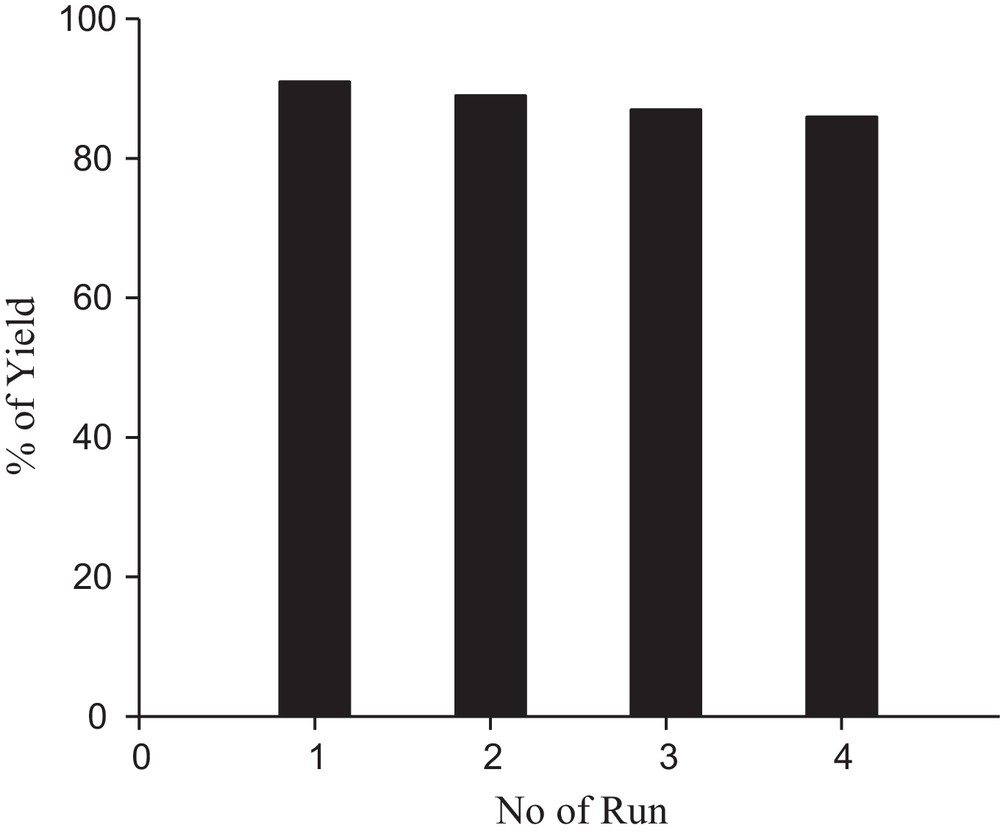
Reusability of the catalyst.
4 Conclusion
A novel and efficient three-component condensation reaction of aryl aldehydes, malononitrile and C–H-activated compounds has been developed for the synthesis of pyran annulated heterocycles. In general, improvements in the rates and product yields are observed when the reaction is carried out in the presence of Ni NPs under ultrasonication; the catalyst can be reused up to four successive runs without any noticeable change in the product yield.
Acknowledgements
We thank the Department of Chemistry, Sophisticated Analytical and Instrumentation Facility (SAIF) of North-Eastern Hill University and IIT (Guwahati). We acknowledge the financial support from DST-SERB (sanctioned No.: SERC/F/0293/2012-13). We are also thankful to Dr S. Mitra and DST-PURSE.