1 Introduction
Thiazolidinone derivatives have been shown to exhibit a wide range of interesting biological activities [1–3]. They are reported to have anti-tumor [4,5], anticonvulsant [6,7], antibacterial [8], antiviral [9], cardiotonic [10,11], and anti-diabetic [12,13] properties. In particular, the 2-amino-5-alkylidene-thiazol-4-one core is found in more than 15,000 molecules synthesized so far [14]. On the other hand, isatin (1H-indole-2,3-dione) derivatives are the privileged scaffold in the modern medicinal chemistry that has a broad spectrum of biological activities, such as anticancer [15], anti-tumor [16], anti-inflammatory, hypoglycemic, analgesic, and anti-pyretic properties [17]. Based on these observations, it could be anticipated that the combination of the two above-mentioned scaffolds in one molecule could produce an interesting series of compounds with enhanced biological activity. Some known examples of such hybrid molecules with anticancer activity are shown in Fig. 1 [18,19].
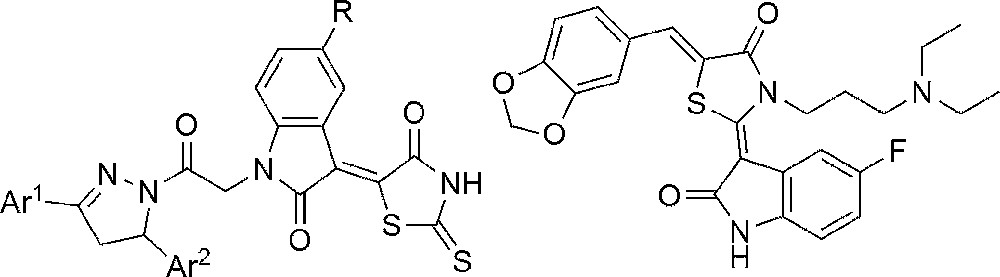
Structure of some isatin-thiazolidinone hybrid molecules with anticancer activity.
Metal oxide nanoparticles have found excellent applications as active adsorbents for gases, for the destruction of hazardous chemicals [20,21], and as catalysts for various organic transformations [22–24]. Their high reactivity is due to their limited size and a high density of corner or edge surface sites [25,26]. Among them, magnesium oxide nanoparticles (MgO–NPs) are most widely used as heterogeneous catalysts and exhibit high activities in numerous base-catalyzed organic reactions [27].
In continuation of our previous works for the synthesis of novel biologically active heterocyclic compounds and the use of green chemical techniques in organic synthesis [28–31], we report herein an efficient and convenient one-pot synthesis of a variety of novel isatin-based conjugates with 2-amino thiazol-4-ones using MgO–NPs as a heterogeneous catalyst in aqueous medium at room temperature (Scheme 1). It involves the displacement of rhodanine thiocarbonyl sulfur with the amine, the resulting aminothiazolone being condensed with the carbonyl group in position 3 of isatin via Knoevenagel condensation [32–34]. Alternatively, rhodanine is first condensed with the carbonyl, and the resulting alkylidene rhodanine is reacted with an amine [35–38]. It should be noted that the reaction of secondary amines with isatin derivatives leads to the formation of 3-dialkylamino-3-hydroxy-2,3-dihydro-1H-indol-2-ones and further indole cycle opening [39], so that these isatin-based conjugate compounds were synthesized by multi-step reactions under harsh reaction conditions [40]. Therefore, this is the first report of the use of a one-pot method for the synthesis of these compounds.
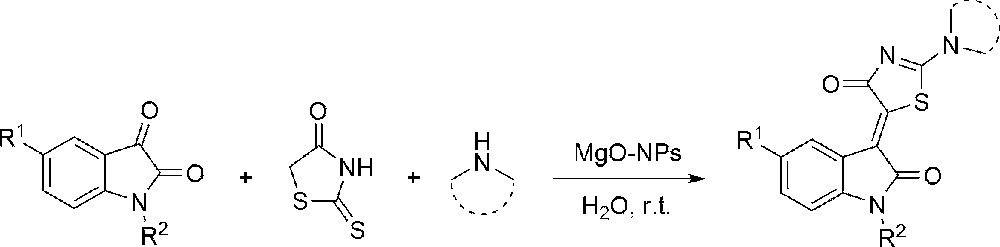
Preparation of isatin-based 2-amino thiazol-4-one conjugates.
2 Results and discussion
First of all, the model reaction of isatin (0.5 mmol), rhodanine (0.5 mmol), and morpholine (0.5 mmol) was optimized under a variety of conditions, and the results are summarized in Table 1. The reaction was first tried with an excess amount (2 equiv.) of morpholine at room temperature in water for 210 min. The yield (isolated) of the desired product was only 10%. However, 5% yield was obtained at 115 min under refluxing conditions. This is because of the nucleophilic attack of the amine to the carbonyl of isatin and the formation of the two-component by-products at high temperature and in the presence of an excess amount of amine [39]. Therefore, using an equivalent amount of amine at low temperature is an essential criterion for good yield and clean reaction. However, when we used 1 equiv. of each compound (rhodanine, isatin, and morpholine) under reflux and catalyst-free conditions, we isolated only 13% of the desired product. Therefore, the presence of a base catalyst is necessary for the Knoevenagel condensation between rhodanine and ketones, particularly, when equivalent quantities of amine are used.
Optimization of the reaction conditionsa.
Entry | Morpholine (equiv.) | Catalyst (equiv.) | Solvent | Temperature (°C) | Time (min) | Isolated yields (%) |
1 | 2 | – | H2O | RT | 210 | 10 |
2 | 2 | – | H2O | Reflux | 115 | 5 |
3 | 1 | – | H2O | RT | 240 | 13 |
4 | 1 | NaOH (0.1) | H2O | RT | 50 | 15 |
5 | 1 | K2CO3 (0.1) | H2O | RT | 60 | 22 |
6 | 1 | DBU (0.1) | H2O | RT | 75 | 27 |
7 | 1 | Et3N (0.1) | H2O | RT | 95 | 35 |
8 | 1 | TiO2 (0.5) | H2O | RT | 140 | 32 |
9 | 1 | Bulk MgO (0.5) | H2O | RT | 150 | 63 |
10 | 1 | MgO–NPs (0.1) | H2O | RT | 120 | 58 |
11 | 1 | MgO–NPs (0.3) | H2O | RT | 120 | 82 |
12 | 1 | MgO–NPs (0.5) | H2O | RT | 110 | 82 |
13 | 1 | MgO–NPs (0.3) | DCM | RT | 160 | 60 |
14 | 1 | MgO–NPs (0.3) | Dry EtOH | RT | 165 | 64 |
15 | 1 | MgO–NPs (0.3) | THF | RT | 210 | 58 |
16 | 1 | MgO–NPs (0.3) | H2O | Reflux | 110 | 82 |
a Reaction conditions: rhodanine (1 equiv., 0.5 mmol), isatin (1 equiv., 0.5 mmol), different amounts of morpholine, different catalysts, different temperatures, stirring.
Then, the reaction was examined in the presence of different base catalysts (Table 1). As it is indicated, the yield is decreased with increasing base strength. This is probably due to the minimization of two-component by-products with weaker base catalyst, though with a higher reaction time. However, MgO–NPs (20 nm diameter, SSA: > 60 M2/g) as a heterogeneous base catalyst gave a maximum 82% yield, whereas when we used bulk MgO, a lower yield of 63% was isolated in the presence of a larger equivalent amount of catalyst. Using 30 mol% of MgO–NPs in water at room temperature is sufficient to push this reaction forward and the yield did not increase largely with a higher amount of catalyst (Table 1, entry 12). The study of the effect of temperature on this reaction indicated that high temperatures could not improve the reaction rate (Table 1, entry 16). The effect of the solvent was also studied. It was observed that using water as the reaction solvent, the reaction time becomes shorter and the yield higher.
After optimization of the model reaction, a variety of isatin-based 2-amino thiazol-4-one conjugates were synthesized with the three-component reaction of rhodanine (1 equiv., 0.5 mmol), secondary amines (1 equiv., 0.5 mmol), and a variety of isatin derivatives (1 equiv., 0.5 mmol) according to Scheme 1. The products were obtained in excellent yields with high purity (Table 2). The structure of the products was confirmed by IR, 1H and 13C NMR spectroscopy, mass spectrometry, and by single-crystal X-ray diffraction analysis (see Fig. 2 and Supplementary data).
Preparation of 3-(2-amino-4-oxo-1-yl-4H-thiazol-5-ylidene)-1,3-dihydro-indol-2-onesa.
Entry | Product | R1 | R2 | Amine | Time (min) | Melting point (°C) | Yield (%) |
1 | 4a | H | H | Piperidine | 95 | 345–347 | 85 |
2 | 4b | H | H | Pyrrolidine | 45 | 250–252 | 80 |
3 | 4c | H | H | Piperazine | 105 | 296–298 | 83 |
4 | 4d | H | H | Morpholine | 120 | 368–370 | 82 |
5 | 4e | Br | H | Piperidine | 100 | 346–348 | 78 |
6 | 4f | Br | H | Pyrrolidine | 50 | 359–361 | 76 |
7 | 4g | Cl | H | Piperidine | 90 | 346–348 | 80 |
8 | 4h | Cl | H | Pyrrolidine | 60 | 347–349 | 79 |
9 | 4i | Cl | H | Diethyl amine | 35 | 289–291 | 84 |
10 | 4j | Cl | H | Morpholine | 110 | 350–352 | 86 |
11 | 4k | H | Benzyl | Piperidine | 85 | 224–226 | 76 |
12 | 4l | H | Benzyl | Pyrrolidine | 125 | 196–198 | 83 |
13 | 4m | H | Benzyl | Morpholine | 120 | 260–262 | 88 |
14 | 4n | H | CH2CO2Et | Piperidine | 105 | 214–216 | 87 |
15 | 4o | H | CH2CO2Et | Morpholine | 130 | 289–291 | 84 |
16 | 4p | Cl | CH2CO2Et | Piperidine | 95 | 225–227 | 81 |
a Reaction and conditions: isatin derivatives (1 equiv., 0. 5 mmol), rhodanine (1 equiv., 0. 5 mmol), amines (1 equiv., 0.5 mmol), MgO-NPs (0.3 equiv., 0.15 mmol), 3 mL H2O, room temperature, stirring.
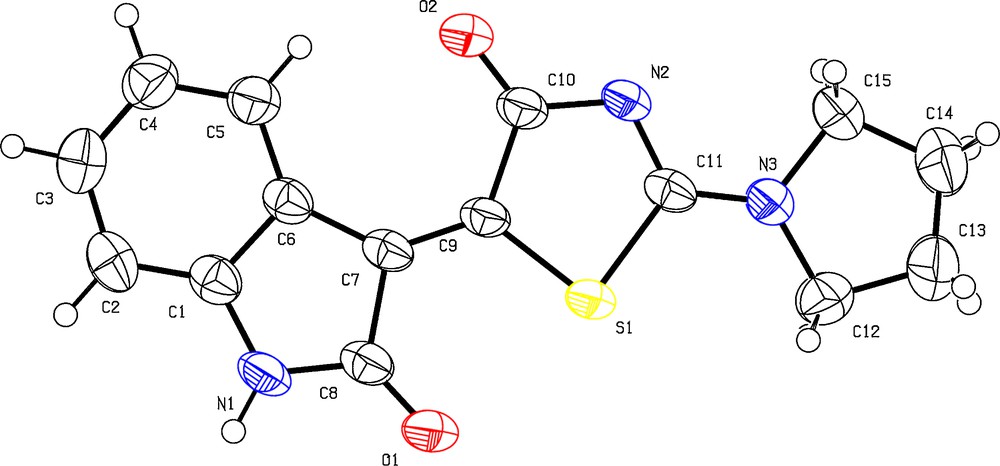
ORTEP diagram of compound 4b.
The mass spectrum of 4b displayed the molecular ion (M+·) peak at m/z 299, which was consistent with the product's structure. The 1H NMR spectrum of 4b in DMSO exhibited one multiplet and two triplet signals due to the four methylene groups of pyrrolidine (δ = 1.97–2.07, 3.68 and 3.74). The aromatic protons appeared as two doublets at 6.93 and 9.00, and two triplets at 7.06 and 7.35 (3JHH = 7.6 Hz). The proton-decoupled 13C NMR spectrum of 4b showed 15 distinct resonances, in agreement with the proposed structure. The 1H NMR spectrum of all the compounds indicated that one aromatic proton is more deshielded than the other aromatic ones (∼9 ppm). As indicated in the ORTEP diagram of 4b, this is probably because all the compounds have Z diastereochemistry, so, the anisotropic effects of the carbonyl group in the thiazolone ring deshielded the adjacent aromatic proton. The selected bond distances and angles for 4b are listed in Table 3.
Selected bond lengths (Å) and angles (°) for 4b.
C(7)–C(9) | 1.349(4) | O(2)–C(10)–C(9) | 123.7(3) |
C(11)–N(2) | 1.319(4) | C(7)–C(9)–C(10) | 127.7(3) |
C(11)–N(3) | 1.314(4) | C(9)–C(7)–C(6) | 134.8(3) |
C(11)–S(1) | 1.776(3) | C(11)–N(3)–C(15) | 121.3(3) |
C(10)–O(2) | 1.209(4) | N(3)–C(11)–S(1) | 119.8(3) |
C(5)–H(5) | 0.9300 | O(1)–C(8)–C(7) | 126.5(3) |
C(6)–C(7) | 1.467(5) | O(2)–C(10)–C(9) | 123.7(3) |
A plausible mechanism for the formation of product 4 is given in Scheme 2. Here, the condensation of rhodanine with the amine preceded the Knoevenagel condensation, as confirmed by the 1H NMR spectra of the isolated product obtained by quenching the reaction of rhodanine, morpholine and isatin after few minutes. The quenching was done by simply removing the catalyst from the reaction mixture through filtration. This three-component reaction proceeds via the dual activation of substrates by MgO–NPs, which have a number of anionic oxidic Lewis basic (O2−/O−) and hydroxylic Brønsted basic (OH) sites along with Mg2+ as a Lewis acid site [27]. The Lewis base moiety of the catalyst activates the methylene group of rhodanine. The carbonyl oxygen of isatin coordinates with the Lewis acid moiety, increasing the electrophilicity of the carbonyl carbon and thereby, making it possible to carry out the reaction at room temperature and in a short time.
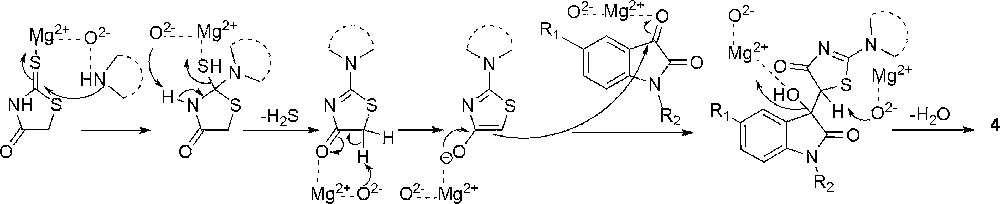
Proposed mechanism for the synthesis of compounds 4a–p.
3 Experimental
3.1 Materials and techniques
All the chemicals and reagents were purchased from Fluka and Merck and used without further purification. Magnesium oxide nanoparticles were obtained from US Research Nanomaterials, Inc. (USA). Melting points were measured on an Electrothermal 9100 apparatus. NMR spectra were recorded with a Bruker DRX-400 AVANCE instrument (400.1 MHz for 1H, 100.6 MHz for 13C) with DMSO as the solvent. Chemical shifts (δ) are given in parts per million (ppm) relative to TMS, and coupling constants (J) are reported in hertz (Hz). IR spectra were recorded on an FT–IR Bruker vector 22 spectrometer. Mass spectra were recorded on a Finnigan-Matt 8430 mass spectrometer, operating at an ionization potential of 70 eV. Elemental analyses were carried with a PerkinElmer 2400II CHNS/O Elemental Analyzer.
3.2 General procedure for the synthesis of compounds 4a–p
A mixture of rhodanine (0.5 mmol), amine (0.5 mmol), and isatin derivatives (0.5 mmol) in water (3 mL) in the presence of 6 mg (30 mol%) of MgO as a catalyst was stirred at room temperature for the specified time period. The completion of the reaction was indicated by the disappearance of the starting material, evidenced by thin layer chromatography (EtOAc/n-hexane, 14:6). After completion of the reaction, the precipitate was filtered out, washed with water, taken in hot methanol and filtered to separate the products from the catalyst. Finally, the solvent was evaporated from product in a rotary evaporator.
3.3 Physical and spectra data for compounds 4a–p
3.3.1 3-(4-Oxo-2-piperidin-1-yl-4H-thiazol-5-ylidene)-1,3-dihydro-indol-2-one (4a)
Orange powder, mp: 345–347 °C; yield (0.13 g, 85%); IR (KBr) νmax: 3353 (NH), 1694 (CO), 1612 (CN) cm−1; 1H NMR (400 MHz, DMSO-d6) δ: 1.65 (brs, 2H, CH2), 1.70 (brs, 4H, 2CH2), 3.67 (brs, 2H, CH2), 3.93 (m, 2H, CH2), 6.93 (d, 3JHH = 7.2, 1H, CHAr), 7.05 (td, 3JHH = 7.6, 4JHH = 1.2, 1H, CHAr), 7.35 (td, 3JHH = 7.6, 4JHH = 1.2, 1H, CHAr), 8.99 (d, 3JHH = 7.60, 1H, CHAr), 11.14 (s,1H, NH); 13C NMR (100 MHz, DMSO-d6) δ: 23.8, 25.7, 26.3, 49.5, 49.9, 110.5, 120.9, 122.3, 125.4, 128.6, 132.0, 138.2, 143.4, 169.4, 176.1, 179.6; MS, m/z: 313.1 (M+·). Anal. calcd for C16H15N3O2S (313.09): C, 61.32; H, 4.82; N, 13.41; S, 10.23. Found: C, 61.07; H, 4.79; N, 13.60; S, 10.28.
3.3.2 3-(4-Oxo-2-pyrrolidin-1-yl-4H-thiazol-5-ylidene)-1,3-dihydro-indol-2-one (4b)
Orange powder, mp: 250–252 °C; yield (0.12 g, 80%); IR (KBr) νmax: 3200 (NH), 1696 (CO), 1616 (CN) cm−1; 1H NMR (400 MHz, DMSO-d6) δ: 1.97–2.07 (m, 4H, 2CH2), 3.68 (t, 3JHH = 6.8, 2H, CH2), 3.74 (t, 3JHH = 6.8, 2H, CH2), 6.93 (d, 3JHH = 7.6, 1H, CHAr), 7.06 (td, 3JHH = 7.6, 4JHH = 1.2, 1H, CHAr), 7.35 (td, 3JHH = 7.6, 4JHH = 1.2, 1H, CHAr), 9.00 (d, 3JHH = 7.6, 1H, CHAr), 11.14 (s, 1H, NH); 13C NMR (100 MHz, DMSO-d6) δ: 24.8, 25.4, 48.9, 51.2, 110.6, 120.9, 122.3, 125.5, 128.6, 132.0, 138.4, 143.4, 169.4, 174.2, 179.0; MS, m/z: 299.0 (M+·). Anal. calcd for C15H13N3O2S (299.07): C, 60.18; H, 4.38; N, 14.04; S, 10.71. Found: C, 60.37; H, 4.29; N, 14.11; S, 10.80. Crystal data for 4b C15H13N3O2S (CCDC 928872): Mw: 299.35, Monoclinic, space group P21/c, unit cell dimensions a = 10.2332(10) Å, b = 8.3723(14) Å, c = 16.2241(17) Å, α = β = γ = 90°, volume = 1385.5(3) Å3, Z = 4, DCalc. = 1.435 mg/m3, F(000) = 624, crystal dimension 0.23 × 0.20 × 0.15 mm, radiation, Mo Kα (λ = 0.71073 Å), θ range: 2.00–29.28, Index ranges:–14 ≤ h ≤ 13,–11 ≤ k ≤ 9,–22 ≤ l ≤ 22, absorption correction: numerical, data/restraints/parameters: 3728/0/190, goodness-of-fit on F2: 1.050, final R indices [I > 2 σ(I)]: R1 = 0.0805, wR2 = 0.1285, largest diffraction peak and hole: 0.250 and –0.257 e Å−3.
3.3.3 3-(4-Oxo-2-piperazin-1-yl-4H-thiazol-5-ylidene)-1,3-dihydro-indol-2-one (4c)
Orange powder, mp: 296–298 °C; yield (0.13 g, 83%); IR (KBr) νmax: 3362 (NH), 1697 (CO), 1617 (CN) cm−1; 1H NMR (400 MHz, DMSO-d6) δ: 2.83–2.88 (m, 4H, 2CH2), 3.61 (m, 2H, CH2), 3.88, (m, 2H, CH2), 6.93 (d, 3JHH = 7.6, 1H, CHAr), 7.05 (t, 3JHH = 7.6, 1H, CHAr), 7.35 (t, 3JHH = 7.6, 1H, CHAr), 8.98 (d, 3JHH = 7.6, 1H, CHAr), 11.13 (s, 1H, NH); 13C NMR (100 MHz, DMSO-d6) δ: 45.5, 46, 49.9, 50.0, 110.6, 120.9, 122.3, 125.5, 128.6, 132.0, 137.8, 143.4, 169.4, 176.5, 179.4; MS, m/z: 314.1 (M+·). Anal. calcd for C15H14N4O2S (314.08): C, 57.31; H, 4.49; N, 17.82; S, 10.20. Found: C, 57.70; H, 4.42; N, 17.68; S, 10.12.
3.3.4 3-(2-Morpholin-4-yl-4-oxo-4H-thiazol-5-ylidene)-1,3-dihydro-indol-2-one (4d)
Orange powder, mp: 368–370 °C; yield (0.13 g, 82%); IR (KBr) νmax: 3404 (NH), 1700 (CO), 1615 (CN) cm−1; 1H NMR (400 MHz, DMSO-d6) δ: 3.73–3.77 (m, 6H, 3CH2), 3.96 (m, 2H, CH2), 6.93 (d, 3JHH = 7.6, 1H, CHAr), 7.06 (t, 3JHH = 7.6, 1H, CHAr), 7.35 (t, 3JHH = 7.6, 1H, CHAr), 9.00 (d, 3JHH = 7.6, 1H, CHAr), 11.15 (s, 1H, NH); 13C NMR (100 MHz, DMSO-d6) δ: 48.6, 48.7, 66.0, 66.2, 110.6, 121.0, 122.3, 125.8, 128.6, 132.2, 137.5, 143.5, 169.4, 177.2, 179.4; MS, m/z: 315.0 (M+·). Anal. calcd for C15H13N3O3S (315.07): C, 57.13; H, 4.16; N, 13.33; S, 10.17. Found: C, 56.86; H, 4.07; N, 13.52; S, 9.94.
3.3.5 5-Bromo-3-(4-oxo-2-piperidin-1-yl-4H-thiaz ol-5-ylidene)-1,3-dihydro-indol-2-one (4e)
Orange powder, mp: 346–348 °C; yield (0.15 g, 78%); IR (KBr) νmax: 3399 (NH), 1702 (CO), 1615 (CN) cm−1; 1H NMR (400 MHz, DMSO-d6) δ: 1.62–1.75 (m, 6H, 3CH2), 3.68 (brs, 2H, CH2), 3.95 (m, 2H, CH2), 6.90 (d, 3JHH = 8.4, 1H, CHAr), 7.53 (dd, 3JHH = 8.4, 4JHH = 2, 1H, CHAr), 9.19 (d, 4JHH = 2, 1H, CHAr), 11.10 (s, 1H, NH); 13C NMR (100 MHz, DMSO-d6) δ: 23.8, 25.8, 26.4, 49.7, 50.0, 112.4, 113.9, 122.7, 124.1, 130.6, 134.1, 140.1, 142.5, 168.8, 175.9, 179.3; MS, m/z: 393.1 (M+·+2) 391.1 (M+·). Anal. calcd for C16H14BrN3O2S (391.00): C, 48.99; H, 3.60; N, 10.71; S, 8.17. Found: C, 48.76; H, 3.60; N, 10.60; S, 8.31.
3.3.6 5-Bromo-3-(4-oxo-2-pyrrolidin-1-yl-4H-thiazol-5-ylidene)-1,3-dihydro-indol-2-one (4f)
Brown powder, mp: 359–361 °C; yield (0.14 g, 76%); IR (KBr) νmax: 3325 (NH), 1698 (CO), 1591 (CN) cm−1; 1H NMR (400 MHz, DMSO-d6) δ: 1.94–2.09 (m, 4H, 2CH2), 3.68 (t, 3JHH = 6.8, 2H, CH2), 3.74 (t, 3JHH = 6.8, CH2), 6.90 (d, 3JHH = 8.4, 1H, CHAr), 7.52 (dd, 3JHH = 8.4, 4JHH = 2, 1H, CHAr), 9.18 (d, 4JHH = 2, 1H, CHAr), 10.97 (s, 1H, NH); 13C NMR (100 MHz, DMSO-d6) δ: 24.8, 25.3, 49.1, 51.3, 112.5, 113.9, 122.5, 124.3, 130.6, 134.1, 135.8, 142.5, 168.8, 173.9, 179.3; MS, m/z: 378.9 (M+·+2), 376.9 (M+·). Anal. calcd for C15H12BrN3O2S (376.98): C, 47.63; H, 3.20; N, 11.11; S, 8.48. Found: C, 47.80; H, 3.35; N, 11.18; S, 8.03.
3.3.7 5-Chloro-3-(4-oxo-2-piperidin-1-yl-4H-thiazol-5-ylidene)-1,3-dihydro-indol-2-one (4g)
Orange powder, mp: 346–348 °C; yield (0.14 g, 80%); IR (KBr) νmax: 3402 (NH), 1701 (CO), 1618 (CN) cm−1; 1H NMR (400 MHz, DMSO-d6) δ: 1.60–1.73 (m, 6H, 3CH2), 3.67 (brs, 2H,–CH2), 3.94 (m, 2H, CH2), 6.94 (d, 3JHH = 8.4, 1H, CHAr), 7.40 (dd, 3JHH = 8.4, 4JHH = 2, 1H, CHAr), 9.05 (d, 4JHH = 2, 1H, CHAr), 11.27 (s, 1H, NH); 13C NMR (100 MHz, DMSO-d6) δ: 23.8, 25.8, 26.4, 49.7, 50.0, 111.9, 111.9, 122.2, 126.1, 127.9, 131.3, 142.0, 142.1, 169.2, 175.9, 179.6; MS, m/z: 349.1 (M+·+2), 347.1 (M+·). Anal. calcd for C16H14ClN3O2S (347.05): C, 55.25; H, 4.06; N, 12.08; S, 9.22. Found: C, 55.49; H, 3.93; N, 12.37; S, 9.40.
3.3.8 5-Chloro-3-(4-oxo-2-pyrrolidin-1-yl-4H-thiazol-5-ylidene)-1,3-dihydro-indol-2-one (4h)
Orange powder, mp: 347–349 °C; yield (0.13 g, 79%); IR (KBr) νmax: 3210 (NH), 1705 (CO), 1617 (CN) cm−1; 1H NMR (400 MHz, DMSO-d6) δ: 1.96–2.08 (m, 4H, 2CH2), 3.69 (t, 3JHH = 6.8, 2H, CH2), 3.75 (t, 3JHH = 6.8, 2H, CH2), 6.94 (d, 3JHH = 8.4, 1H, CHAr), 7.40 (dd, 3JHH = 8.4, 4JHH = 2.4, 1H, CHAr), 9.06 (d, 4JHH = 2.4, 1H, CHAr), 11.27 (s, 1H, NH); 13C NMR (100 MHz, DMSO-d6) δ: 24.8, 25.4, 49.1, 51.3, 111.9, 122.2, 124.4, 126.1, 127.9, 131.3, 131.3, 142.2, 169.1, 174.0, 178.9; MS, m/z: 335.0 (M+·+2), 333.0 (M+·). Anal. calcd for C15H12ClN3O2S (333.03): C, 53.97; H, 3.62; N, 12.59; S, 9.61. Found: C, 54.26; H, 3.58; N, 12.75; S, 9.49.
3.3.9 5-Chloro-3-(2-diethylamino-4-oxo-4H-thiazol-5-ylidene)-1,3-dihydro-indol-2-one (4i)
Orange-red powder, mp: 289–291 °C; yield (0.14 g, 84%); IR (KBr) νmax: 3483 (NH), 1689 (CO), 1651 (CN) cm−1; 1H NMR (400 MHz, DMSO-d6) δ: 1.151 (t, 3JHH = 7.2, 3H, CH3), 2.92 (q, 3JHH = 7.2, 2H, CH2), 6.88 (d, 3JHH = 8.4, 1H, CHAr), 7.31 (dd, 3JHH = 8.4, 4JHH = 2.4, 1H, CHAr), 9.12 (d, 4JHH = 2.4, 1H, CHAr), 10.95 (s, 1H, NH); 13C NMR (100 MHz, DMSO-d6) δ: 11.7, 41.9, 111.3, 117.4, 123.0, 125.6, 127.0, 129.7, 141.8, 148.8, 169.1, 176.0, 184.1; MS, m/z: 337.1 (M+·+2), 335.1 (M+·). Anal. calcd for C15H14ClN3O2S (335.05): C, 53.65; H, 4.20; N, 12.51; S, 9.55. Found: C, 53.92; H, 4.39; N, 12.07; S, 9.83.
3.3.10 5-Chloro-3-(2-morpholin-4-yl-4-oxo-4H-thiazol-5-ylidene)-1,3-dihydro-indol-2-one (4j)
Brick-red powder, mp: 350–352 °C; yield (0.15 g, 86%); IR (KBr) νmax: 3409 (NH), 1705 (CO), 1617 (CN) cm−1; 1H NMR (400 MHz, DMSO-d6) δ: 3.67–3.79 (m, 6H, 3CH2), 3.96 (m, 2H, CH2), 6.95 (d, 3JHH = 8, 1H, CHAr), 7.41 (dd, 3JHH = 8.4, 4JHH = 2, 1H, CHAr), 9.03 (d, 4JHH = 2, 1H, CHAr), 11.29 (s, 1H, NH); 13C NMR (100 MHz, DMSO-d6) δ: 48.8, 48.9, 66.0, 66.2, 112.0, 122.1, 124.6, 126.1, 127.8, 131.4, 139.4, 142.2, 169.1, 177.0, 179.4; MS, m/z: 351.0 (M+·+2), 349.0 (M+·). Anal. calcd for C15H12ClN3O3S (349.03): C, 51.51; H, 3.46; N, 12.01; S, 9.17. Found: C, 51.36; H, 3.41; N, 12.29; S, 9.12.
3.3.11 1-Benzyl-3-(4-oxo-2-piperidin-1-yl-4H-thiazol-5-ylidene)-1,3-dihydro-indol-2-one (4k)
Orange powder, mp: 224–226 °C; yield (0.15 g, 76%); IR (KBr) νmax: 1689 (CO), 1653 (CN) cm−1; 1H NMR (400 MHz, DMSO-d6) δ: 1.50–1.58 (m, 2H, CH2), 1.58–1.63 (m, 4H, 2CH2), 2.98 (m, 4H, 2CH2), 5.01 (s, 2H, CH2), 6.97 (d, 3JHH = 8, 1H, CHAr), 7.04 (t, 3JHH = 8, 1H, CHAr), 7.22–7.35 (m, 6H, 6CHAr), 9.11 (d, 3JHH = 8, 1H, CHAr); 13C NMR (100 MHz, DMSO-d6) δ: 22.4, 23.1, 43.4, 44.5, 109.4, 117.3, 121.1, 122.4, 127.6, 127.9, 129.1, 130.2, 137.0, 138.9, 143.1, 148.3, 168.1, 176.2, 183.9; MS, m/z: 403.1 (M+·). Anal. calcd for C23H21N3O2S (403.14): C, 68.46; H, 5.25; N, 10.41; S, 7.95. Found: C, 68.22; H, 5.37; N, 10.17; S, 7.83.
3.3.12 1-Benzyl-3-(4-oxo-2-pyrrolidin-1-yl-4H-thiazol-5-ylidene)-1,3-dihydro-indol-2-one (4l)
Yellow powder, mp: 196–198 °C; yield (0.16 g, 83%); IR (KBr) νmax: 1686 (CO), 1655 (CN) cm−1; 1H NMR (400 MHz, DMSO-d6) δ: 1.79–1.87 (m, 4H, 2CH2), 3.10 (m, 4H, 2CH2), 5.01 (s, 2H, CH2), 6.97 (d, 3JHH = 7.6, 1H, CHAr), 7.04 (td, 3JHH = 7.6, 4JHH = 0.8, 1H, CHAr), 7.23–7.35 (m, 6H, 6CHAr), 9.11 (d, 3JHH = 7.6, 1H, CHAr); 13C NMR (100 MHz, DMSO-d6) δ: 24.1, 43.4, 45.4, 109.4, 117.3, 121.1, 122.4, 127.6, 127.9, 129.1, 129.1, 130.2, 137.0, 143.1, 148.3, 168.0, 177.8, 183.9; MS, m/z: 389.1 (M+·). Anal. calcd for C22H19N3O2S (389.12): C, 67.84; H, 4.92; N, 10.79; S, 8.23. Found: C, 67.60; H, 5.00; N, 10.84; S, 8.36.
3.3.13 1-Benzyl-3-(2-morpholin-4-yl-4-oxo-4H-thiazol-5-ylidene)-1,3-dihydro-indol-2-one (4m)
Yellow powder, mp: 260–262 °C; yield (0.18 g, 88%); IR (KBr) νmax: 1681 (CO), 1612 (CN) cm−1; 1H NMR (400 MHz, DMSO-d6) δ: 3.71–3.80 (m, 6H, 3CH2), 3.97 (m, 2H, CH2), 5.03 (s, 2H, CH2), 7.06 (d, 3JHH = 8, 1H, CHAr), 7.11 (t, 3JHH = 8, 1H, CHAr), 7.24–7.35 (m, 5H, 5CHAr), 7.37 (t, 3JHH = 8, 1H, CHAr), 9.04 (d, 3JHH = 8, 1H, CHAr); 13C NMR (100 MHz, DMSO-d6) δ: 43.6, 48.7, 48.8, 66.0, 66.2, 110.0, 120.3, 123.1, 124.6, 127.8, 128.0, 128.1, 129.2, 132.0, 136.5, 138.9, 143.5, 168.1, 176.8, 179.1; MS, m/z: 405.1 (M+·). Anal. calcd for C22H19N3O3S (405.11): C, 65.17; H, 4.72; N, 10.36; S, 7.91. Found: C, 65.01; H, 4.67; N, 10.41; S, 7.80.
3.3.14 [2-Oxo-3-(4-oxo-2-piperidin-1-yl-4H-thiazol-5-ylidene)-2,3-dihydro-indol-1-yl]-acetic acid ethyl ester (4n)
Yellow powder, mp: 214–216 °C; yield (0.17 g, 87%); IR (KBr) νmax: 1745, 1674 (CO) 1611 (CN) cm−1; 1H NMR (400 MHz, DMSO-d6) δ: 1.21 (t, 3JHH = 6.8, 3H, CH3), 1.61–1.74 (m, 6H, 3CH2), 3.67 (brs, 2H, CH2), 3.94 (brs, 2H, CH2), 4.16 (q, 3JHH = 6.8), 4.71 (s, 2H, CH2), 7.15 (m, 2H, 2CHAr), 7.43 (t, 3JHH = 7.6, 1H, CHAr), 9.07 (d, 3JHH = 7.6, 1H, CHAr); 13C NMR (100 MHz, DMSO-d6) δ: 14.5, 23.8, 25.7, 26.3, 41.9, 49.7, 50.0, 61.8, 109.7, 120.2, 123.2, 123.8, 126.7, 128.4, 131.9, 143.4, 168.2, 175.5, 179.2, 179.4; MS, m/z: 399.3 (M+·). Anal. calcd for C20H21N3O4S (399.46): C, 60.13; H, 5.30; N, 10.52; S, 8.03. Found: C, 60.31; H, 5.32; N, 10.37; S, 7.89.
3.3.15 [3-(2-Morpholin-4-yl-4-oxo-4H-thiazol-5-ylidene)-2-oxo-2,3-dihydro-indol-1-yl]-acetic acid ethyl ester (4o)
Yellow powder, mp: 289–291 °C; yield (0.17 g, 84%); IR (KBr) νmax: 1744, 1679 (CO) 1611 (CN) cm−1; 1H NMR (400 MHz, DMSO-d6) δ: 1.21 (t, 3JHH = 7, 3H, CH3), 3.68–3.80 (m, 6H, 3CH2), 3.97 (m, 2H, CH2), 4.16 (q, 3JHH = 7, 2H, CH2), 4.72 (s, 2H, CH2), 7.16 (m, 2H, 2CHAr), 7.44 (td, 3JHH = 7.6, 4JHH = 1.2, 1H, CHAr), 9.06 (d, 3JHH = 7.6, 1H, CHAr); 13C NMR (100 MHz, DMSO-d6) δ: 14.5, 42.0, 48.7, 48.8, 61.8, 66.0, 66.1, 109.7, 120.2, 123.2, 124.2, 128.4, 132.1, 139.1, 143.5, 168.1, 168.2, 176.7, 179.1; MS, m/z: 401.1 (M+·). Anal. calcd for C19H19N3O5S (401.10): C, 56.85; H, 4.77; N, 10.47; S, 7.99. Found: C, 56.61; H, 4.72; N, 10.27; S, 7.94.
3.3.16 [5-Chloro-2-oxo-3-(4-oxo-2-piperidin-1-yl-4H-thiazol-5-ylidene)-2,3-dihydro-indol-1-yl]-acetic acid ethyl ester (4p)
Red powder, mp: 225–227 °C; yield (0.18 g, 81%); IR (KBr) νmax: 1742, 1694 (CO), 1612 (CN) cm−1; 1H NMR (400 MHz, DMSO-d6) δ: 1.20 (t, 3JHH = 7.2, 3H, CH3), 1.52–1.59 (m, 2H, CH2), 1.60–1.69 (m, 4H, 2CH2), 3.01 (m, 4H, 2CH2), 4.15 (q, 3JHH = 7.2, 2H, CH2), 4.68 (s, 2H, CH2), 7.11 (d, 3JHH = 8.8, 1H, CHAr), 7.40 (dd, 3JHH = 8.6, 4JHH = 2.4, 1H, CHAr), 9.05 (d, 4JHH = 2.4, 1H, CHAr); 13C NMR (100 MHz, DMSO-d6) δ: 14.5, 22.1, 22,7, 41.9, 44.2, 61.7, 110.6, 122.2, 122.6, 126.6, 126.9, 129.5, 139.2, 141.8, 167.7, 168.3, 172.8, 184.1; MS, m/z: 435.3 (M+·+2), 433.3 (M+·). Anal. calcd for C20H20ClN3O4S (433.09): C, 55.36; H, 4.65; N, 9.68; S, 7.39. Found: C, 55.00; H, 4.68; N, 9.47; S, 7.49.
4 Conclusion
In conclusion, we have proposed an efficient, clean, economic and one-pot procedure for the synthesis of novel isatin-based 2-aminothiazol-4-one conjugates by the three-component coupling of isatin, amine, and rhodanine over MgO nanoparticle catalyst in water as a green reaction medium. Mild reaction conditions, short reaction time, excellent yields of the products make this methodology highly significant. It represents a powerfully green technology procedure using an environmentally friendly solvent and avoiding unwanted waste production for the synthesis of new derivatives of isatin. The products were isolated without any further purification process like column chromatography.
Acknowledgments
This research was supported by the Research Council of the University of Mazandaran, Iran.