1 Introduction
The mine water and the wastewater usually discharge various metal ions into rivers, estuaries, streams, and lakes [1]. Natural water contains a series of common cations, such as Ca2+, Mg2+, K+, and Na+, which confer the taste and quality of water and also some trace heavy metals, like Pb2+, Fe+2, Fe+3, Zn2+, Cd2+, Cu2+, Ni2+, and Al3+, etc., which determine the toxicity of water [2–5]. It is well known that heavy metals are very harmful because of their non-biodegradable nature, long biological half-lives and their potential to accumulate in different body parts. Metal toxicity produces adverse biological effects on the organism survival, activity, growth, metabolism, or reproduction. Metals can be lethal or harmful for the organism without killing it directly [6].
In Romania for instance, in the Rosia Montana area, the mine waters discharged from gold and silver exploitations have a high content of metal ions, especially Ca2+, Al3+, Pb2+, Ni2+, Mn2+, and Cu2+, etc., and a pH range from 2 to 4.5 [7]. Concentration of metal ions in mine waters ranges between 100 and 400 mg/L for Ca2+, between 5 and 40 mg/L for Pb2+ ion, between 30 and 300 mg/L for Al3+ ion, and between 5 and 20 mg/L for Ni2+ ion [8]. For selective recovery of toxic ions from acidic waters, appropriate selective ion exchangers can be used, such as Amberlite IRC-718, Purolite® S930, Dowex A 1, etc. [9]. These resins have a high ion exchange capacity for Ni2+, Pb2+, and Al3+, and rather low for Ca2+, providing treated mine water of appropriate quality in compliance with regulations in force, HG No. 188/2002.
Aluminium and its salts are being extensively used in the present industrialized world in various industries based on food and beverages, drugs, packing materials, and dye industry [10]. A high level of aluminium in wastewaters inhibits the optimal growth of microorganisms and stabilizes the organic materials in water. Elevated concentrations of Al3+ ions in water bodies are highly objectionable as they are reported to be neurotoxins [11,12], causing harmful effects on crop production, fish [13], zooplankton, [14], cyanobacteria [15] and algae [16]. Moreover, it is implicated in dialysis, dementia, Parkinson and Alzheimer's diseases [17,18], bone softening, renal insufficiency, pulmonary fibrosis and microcytic, anaemia in human beings [19].
Nickel is easily accumulated in the biota, particularly in the phytoplankton or other aquatic plants, which are sensitive bioindicators of water pollution. It can be deposited in the sediments by processes, such as precipitation, complexation, and adsorption on clay particles and via uptake by biota [20]. Nickel exposure is frequently responsible for allergic skin reactions. Nickel hypersensitivity also causes asthma, conjunctivitis, inflammatory reactions to nickel-containing prostheses and implants, and systemic reactions after parenteral administration of nickel-contaminated fluids and medications [21]. Epidemiological investigations and experimental studies have demonstrated that nickel metal dusts and some nickel compounds are extremely potent carcinogens after inhalation, but also that the carcinogenic risk is limited to conditions of occupational exposure [22].
Lead has become widely distributed and mobilized in the environment, and human exposure and uptake of this non-essential element have consequently increased [23]. Lead is an important toxicant that can exert adverse effects in humans, after sufficient exposure and accumulation in the body. Many parts of the organisms are known to be susceptible to adverse effects of high exposure, including neurological, reproductive, renal, and hematological systems. Children are more sensitive than adults to the effects of lead, and precautions should be taken to limit childhood exposure and keep blood lead levels below the recommended level of 10 μg/dL [24,25].
The main procedures commonly used to reduce the heavy metals content of effluents include chemical precipitation [26], ion exchange [27], adsorption [28], adsorption on non-ionic microemulsions [29], membrane processes [30–32] and electrolytic methods [33].
This paper aims at studying the removal of Ca2+, Ni2+, Pb2+ and Al3+ ions on ion exchange resin S930 from both mono-component and quaternary aquatic systems. The equilibrium of ion exchange processes in mono-component systems has been studied as an adsorption process and assessed by using Langmuir and Freundlich equations. Sorption of these cations and their selectivity on S930 resin has been investigated as a function of pH in these mono- and quaternary systems. The intimate sorption mechanism has been interpreted based on the structural characteristics of pores in the initial resin and the resin after contact with solutions at various pH values by using SEM and EDAX techniques.
2 Experimental
2.1 Materials
Mono- and poly-component aqueous solutions of metals were prepared from corresponding salts: CaCl2, NiCl2, Pb(NO3)2, Al2(SO4)3·18H2O (all p.a. Fluka).
The S930 resin used in this study is a cationic ion exchange resin offered by Purolite SA Romania. Purolite® S930 is a macroporous copolymer (styrene–divinylbenzene) based on chelating resin, with iminodiacetic groups designed for the removal of cations of heavy metals from industrial effluents [34]. These cations may be separated from high concentrations of univalent cations (typically sodium) and also from common divalent cations (such as calcium). Metal removal can be achieved both from weakly acidic or basic solutions, depending on the cation species to be removed. Purolite® S930 is used in processes for extraction and recovery of metals from ores, galvanic plating solutions, pickling baths, and effluents, even in the presence of alkaline earth metals (calcium and magnesium). Fig. 1 illustrates the chemical structure of Purolite® S930 [34].
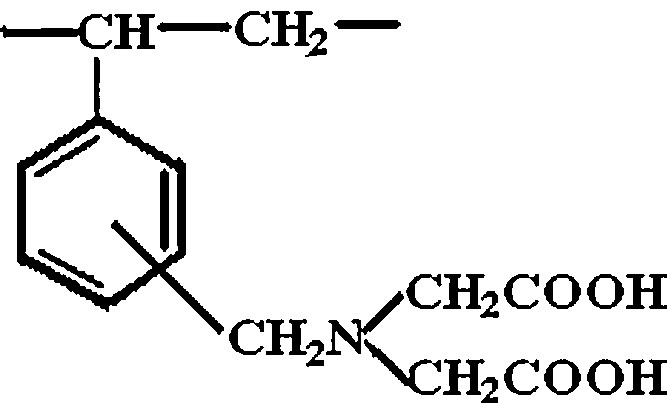
Chemical structure of Purolite® S930.
2.2 Adsorption experiments
For equilibrium studies, the initial concentration of metals was varied between 1 and 10 mequiv/L.
Sorption of Ca2+, Ni2+, Pb2+ and Al3+ on the ion exchange resin has been performed in batch experiments at 20.0 ± 0.1 °C, in glass vessels immersed in a thermostatic waterbath. The concentration of metals in the supernatant was determined by flame atomic absorption spectroscopy for Ca2+, Ni2+, Pb2+, (PerkinElmer 1100B) and using atomic absorption spectroscopy technique with a graphite furnace for Al3+ (Zeenit-700, Analytic Jena AG).
Rotating magnetic bars ensured vigorous stirring of the suspension for equilibration with an interim adjustment of their pH. The desired pH was controlled by using the suitable buffer solution (Merck, titrisol). According to a previous kinetic study, a 24-h reaction period was chosen [35]. All measurements were run in triplicate. The reported values represent the average values. In all the sorption experiments, the solid/liquid ratio was 1 g/L.
The amount of adsorbed metal was calculated by the difference between the initial and final solution concentrations. The mass balance equation for ion uptake is:
a = (CiVi – CeVf)/m | (1) |
The selectivity coefficient of Purolite® S930 resin for each studied metal (Ni2+, Pb2+, Al3+) has been calculated by reference to Ca2+, using the equation:
(2) |
2.3 Morphostructural characterization
The scanning electron microscopy (SEM) was used to identify the structural characteristics of pores. An electronic microscope HITACHI S2600N microscope equipped with EDAX detector has been used.
3 Results and discussion
3.1 Adsorption isotherms
The experimental data obtained for sorption isotherms in mono-component systems at two pH values, 3.03 and 6.5, are illustrated in Figs. 2 and 3, together with their fitting on Langmuir and Freudlich models [36]. One can observe that at lower pH (Fig. 2), the maximum uptake capacity is obtained for Al3+, 1.25 mequiv/g, as compared to Ni2+, Pb2+, and Ca2+, with lower sorption values of 0.61 mequiv/g, 0.39 mequiv/g, 0.14 mequiv/g, respectively. At pH = 6.5, the resin exhibits higher uptake capacities than for pH = 3.03, and higher affinities for Ni2+, and Pb2+, 1.36 mequiv/g, 1.01 mequiv/g respectively, than for Ca2+, 0.5715 mequiv/g. Adsorption capacities obtained in the present work are comparable to those obtained by Bulai et al. in 2008 for copper and nickel on S930 [37]. One may notice that the sorption of Al3+ is not possible to be observed at pH > 4.3 due to its precipitation.
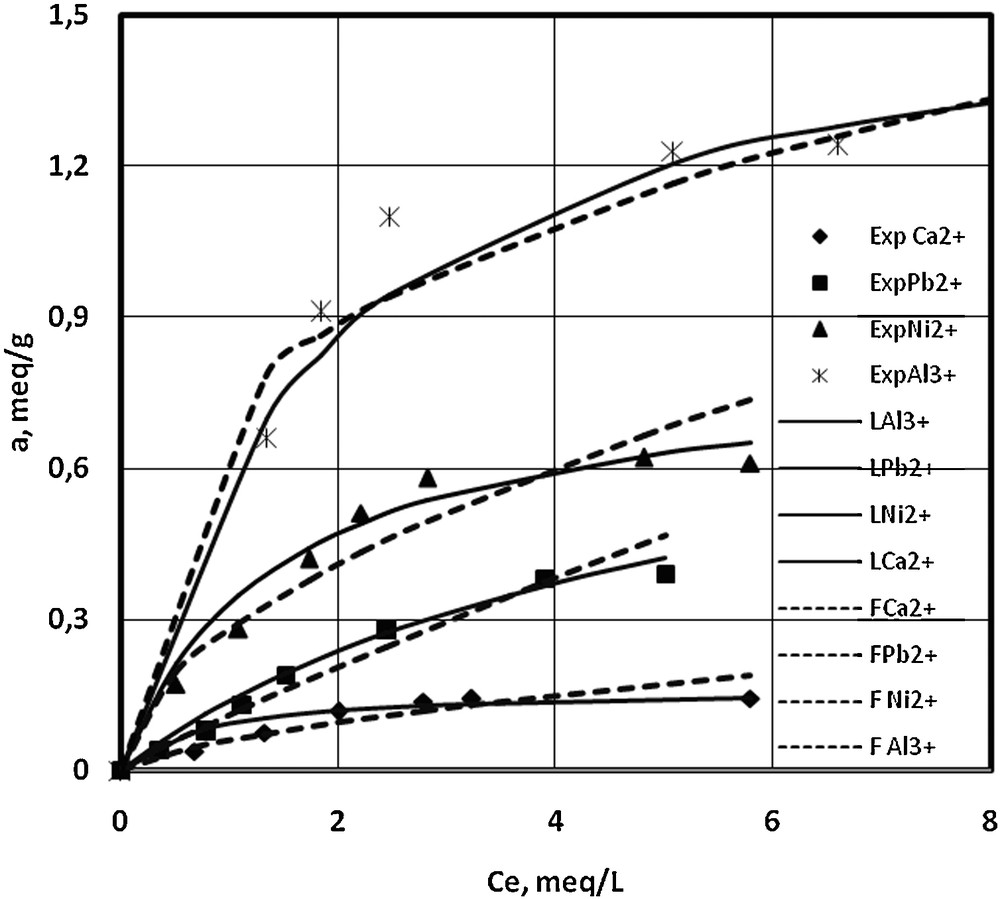
Sorption isotherms of Ca2+, Ni2+, Pb2+ and Al3+ on S930 resin, in mono-component systems, pH = 3.03 (experimental data, Langmuir and Freundlich models).
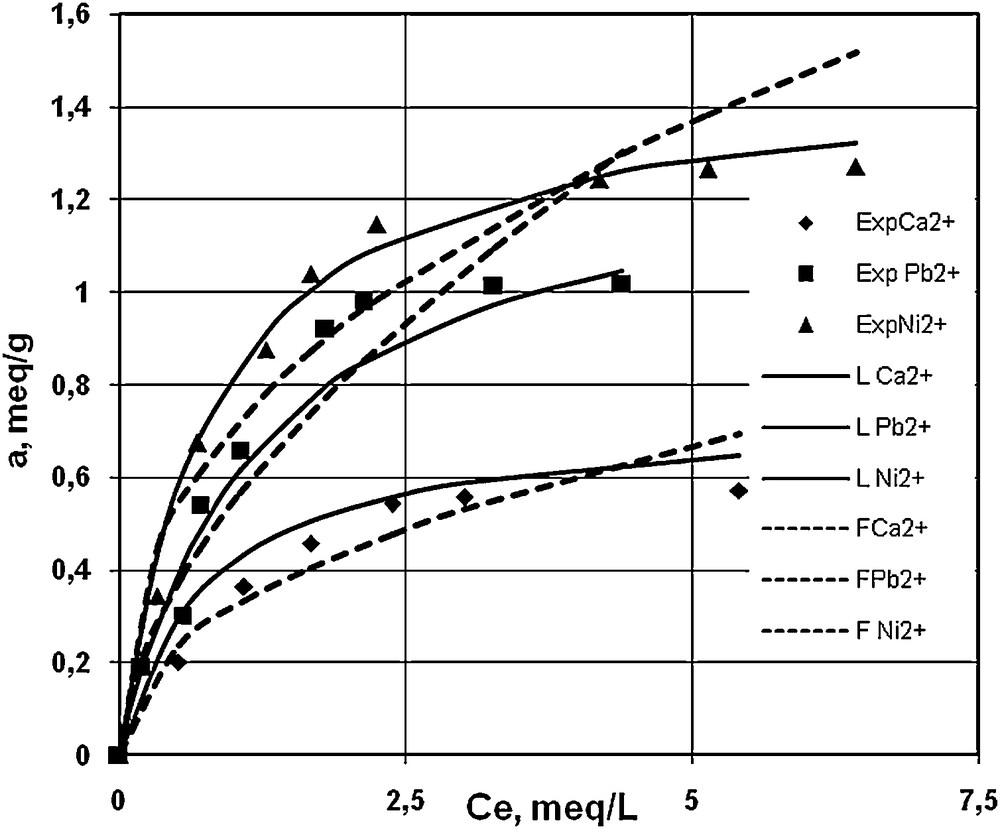
Sorption isotherms of Ca2+, Ni2+, and Pb2+ on S930 resin, in mono-component systems, pH = 6.5 (experimental data, Langmuir and Freundlich models).
In order to assess the sorption mechanism, we used the characteristic equation of the Langmuir isotherm:
(3) |
The linearized form of the Langmuir equation, after rearrangement of terms, is:
(4) |
Based on this linear dependence, the constant b and the maximal sorption capacity am can be easily determined in order to estimate the degree of fitting of this model.
In a similar manner, the characteristic equation of the Freundlich isotherm is:
ae = KCe1/n | (5) |
log ae = log K + (1/n) log Ce | (6) |
The values of the equilibrium parameters for the studied cations on the S930 resin in the cases of both Langmuir and Freundlich equations are collected in Table 1.
Equilibrium parameters for fitting Langmuir and Freundlich equations.
Cation | Langmuir isotherm | Freundlich isotherm | ||||
am | b | R2 | k | n | R2 | |
pH = 3.03 | ||||||
Ca2+ | 0.21 | 1.28 | 0.758 | 0.06 | 1.53 | 0.852 |
Pb2+ | 0.845 | 0.19 | 0.988 | 0.11 | 1.11 | 0.977 |
Ni2+ | 0.81 | 0.69 | 0.878 | 0.28 | 1.82 | 0.914 |
Al3+ | 1.64 | 0.556 | 0.889 | 0.718 | 3.355 | 0.785 |
pH = 6.5 | ||||||
Ca2+ | 0.67 | 1.273 | 0.974 | 0.32 | 2.19 | 0.871 |
Pb2+ | 1.34 | 0.802 | 0.937 | 0.547 | 1.7 | 0.910 |
Ni2+ | 1.49 | 1.22 | 0.985 | 0.706 | 2.43 | 0.865 |
Comparing Figs. 2 and 3 and the values presented in Table 1, one can affirm that the Langmuir model satisfactorily characterizes the sorption isotherms of Ca2+, Ni2+ and Pb2+ at pH = 6.5, in accordance with the surface homogeneity of the resin, even though the uptake capacity is higher than for lower pHs; some repulsions among adsorbed species could appear. However, at pH = 3.03, the Freundlich model seems to be better fitted for these three cations, while Al3+ shows an atypical behaviour, suggesting a more complex mechanism.
3.2 Influence of solution pH on cation removal
The effect of the solution pH on the cation removal in mono-components systems is presented in Fig. 4. The pH was varied within the range 2.02–6.5 for Ca2+, Ni 2+, Pb2+ and the range 2.02–5.03 for Al3+. The lower limit of the possible pH value for Ca2+ or Pb2+ sorption was imposed by the dissolution of resin (pH < 2). The upper pH limit was selected to avoid precipitation of pure or mixed Ca2+, Ni 2+, Pb2+ and Al3+ carbonates or hydroxides (pH > 7.0 for Pb2+; pH > 10 for Ca2+, pH > 7.8 for Ni2+, pH > 4.3 for Al3+) [38,39]. One can notice that the increase of pH results in increasing the adsorption capacity of resin. A similar behavior was observed for Amberlite IRC-718, an iminodiacetic ion exchange resin for Ni ions, [40] and on S930 for copper ions [41].
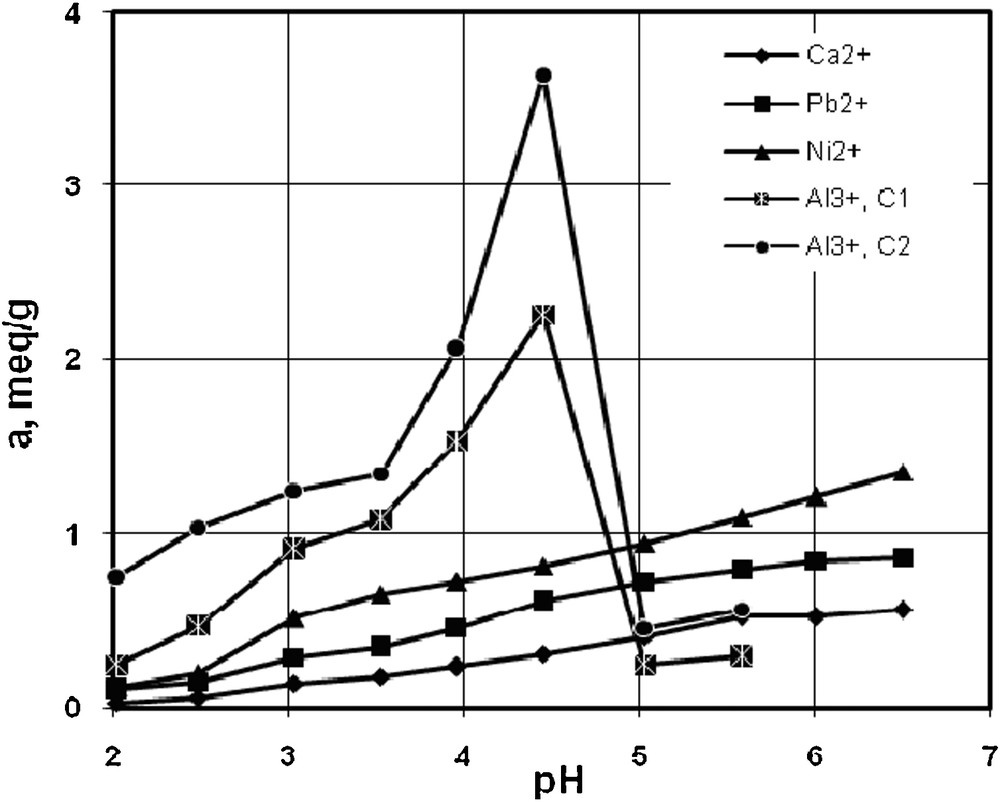
The influence of pH on sorption capacity by S930 resin, in mono-component systems: Ci for Ca2+, 2.73 mequiv/L, Ni2+ and Pb2+, 2.72 mequiv/L, and Al3+, C1 = 2.75 mequiv/L, C2 = 7.83 mequiv/L.
It is supposed that while the pH increases, the proton concentration inside the aqueous medium is lowered and the dissociation processes of hydroxyl and carboxyl active groups are intensified, and thus, the metal ions can be more easily bound on the resin active centres.
In the pH range of 2.02–6.5, the ion exchange capacity for Ca2+, Ni2+, Pb2+ increases relatively linearly with pH. According to the literature studies, Al3+ precipitation starts at pH 4.3 and stops at 4.8 [42,43]. One can observe that the sorption capacity of Al3+ at the initial concentration of 2.75 mequiv/g increases rapidly at pH = 3.95 until the uptake of 1.76 mequiv/L, and at pH = 4.45, a maximum value of 2.23 mequiv/L is reached. For a higher initial concentration of Al3+, 7.83 mequiv/L, the increase of the resin's sorption capacity is even steeper, reaching a higher maximum uptake of 3.66 mequiv/L, much above the total sorption capacity of the resin.
We can explain this phenomenon by the fact that the precipitation of Al3+ at pH = 3.95 starts probably by microprecipitation of metals on the resin surface. At pH = 4.45, the amount of Al3+ determined is 2.5 times the maximum capacity of resin. By rising further the pH at 5.03, the sorption capacity for Al3+ decreases sharply at 0.57 mequiv/L.
Simultaneous sorption of Ca2+, Ni2+, Pb2+and Al3+ from their quaternary mixed solutions is shown as a function of pH in Fig. 5, the ratio concentrations of metals in mequiv/L being 1:1:1:1. A similar trend, with a maximum uptake at pH = 4.45, is obtained in the cation mixture for the total sorption capacity of the resin, following the Al3+ pattern. However, as expected, the individual sorption capacity for metals is lower in the quaternary system as compared to mono-component systems, due to the competition of the four cations for the same active sites of the resin.
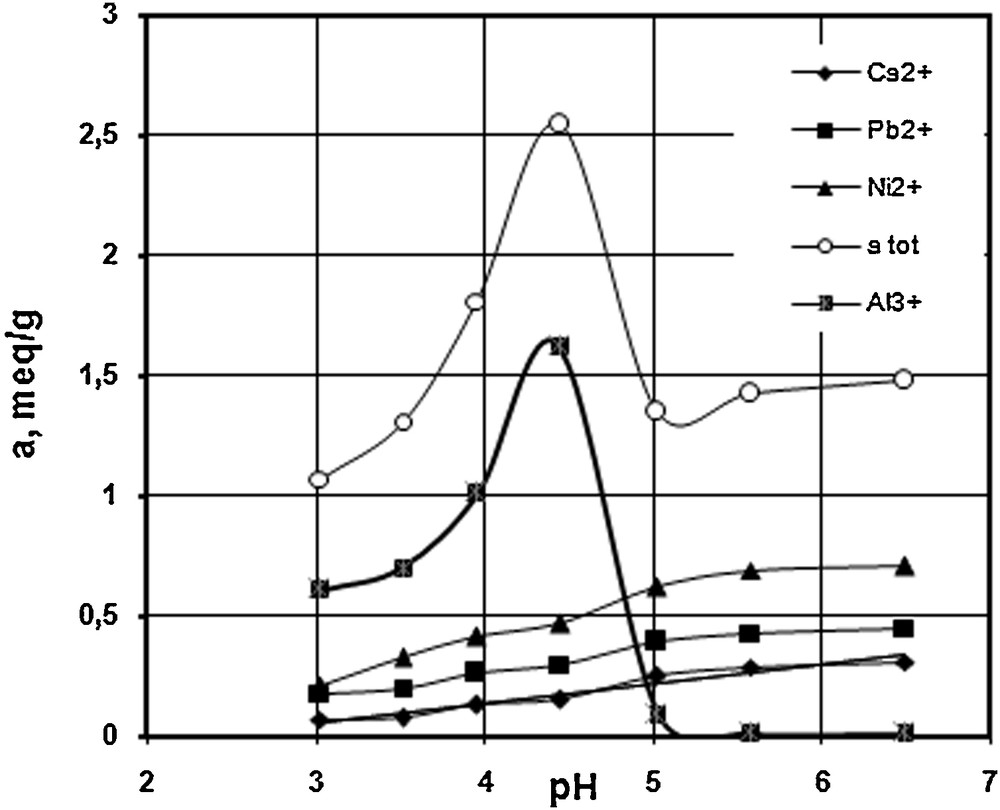
The influence of pH on sorption capacity by S930 resin, in a quaternary system ratio 1:1:1:1: Ci for Ca2+, 2.92 mequiv/L, Ni2+ and Pb2+, 2.7 mequiv/L, and Al3+, 2.75 mequiv/L.
3.3 Separation efficiency
The selectivity coefficient, KM/Ca, of S930 resin related to Ca2+ affinity in the studied pH range is illustrated in Fig. 6. When KM/Ca > 1, the resin exhibits selectivity for metals cations, while for KM/Ca < 1, the resin exhibits selectivity for Ca2+ [42]. One can observe that the highest selectivity of the resin is manifested against Ni2+, followed by Pb2+, while for Al3+ a similar pattern with a maximum at pH = 4.45 is noticed as in the case of a change of sorption capacity in the same pH range, attributed to the microprecipitation of aluminium on the lattice, which contributes to increase the resin's selectivity.
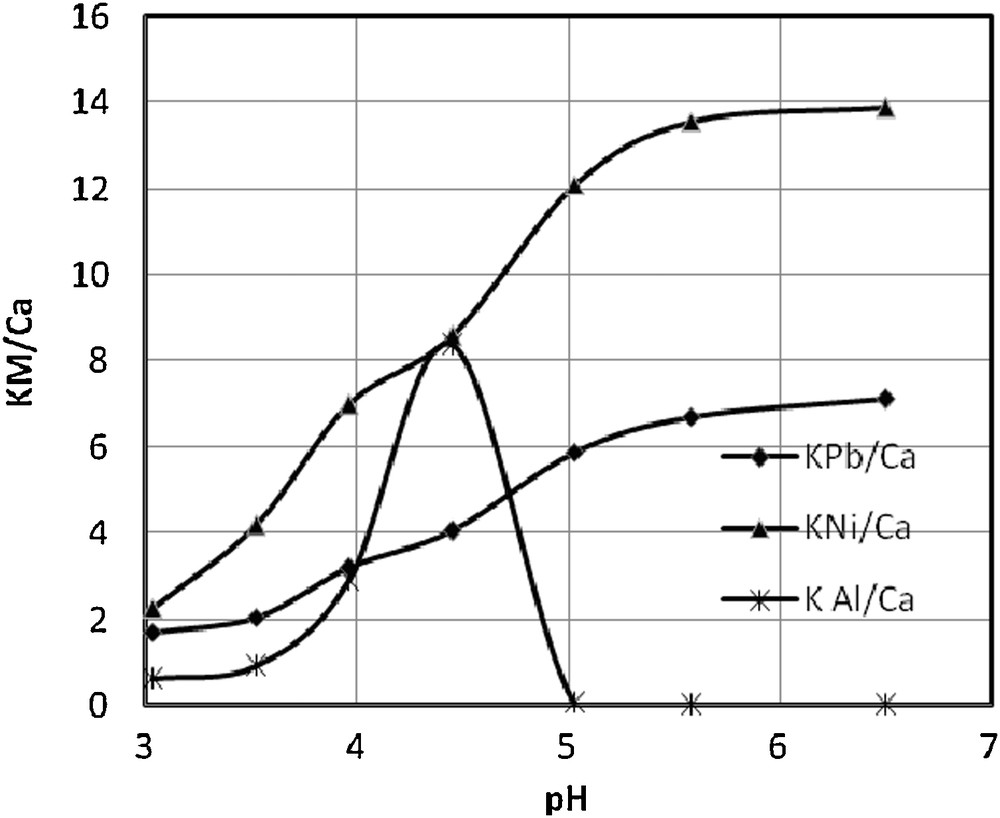
The pH influence on the selectivity coefficient in a quaternary system.
The results obtained in this paper are comparable to those obtained for commercial ion exchange resins, such as Chelex 100, Dowex A 1, CR-20, Lewatit TP 207, Lewatit TP 208, Purolite S 930, Amberlite IRC, Wofatit 718 and MC-50, chelating ion exchangers with the iminodiacetic functional groups. The selectivity of resins for metal ions varies in the order: Cr3+ > Cu2+>Ni2+ > Zn2+ > Co2+ > Cd2+ > Fe2+ > Mn2+ > Ca2+ >> Na+ [43].
Moreover, the selectivity of the resin can be also analyzed by means of structural characteristics of metal ions: the electrical load (charge), crystallographic radius of ions (volume of hydrated ions), ionic, van der Waals or covalent radius, ionization energy and electronegativity on Pauling scale, on the one hand, and the characteristics of functional groups of the resin, on the other hand. An overview of the atomic properties for the studied metal ions in aqueous solution is given in Table 2.
Overview of the atomic properties of the studied metal ions in aqueous solution [46,48].
Aqua complex | M–O distance (Å) | Hydrated radius (Å) | Ionic radius (Å) | Van der Waals radius (Å) [46] | Covalent radius (Å) | Ionization energy (kJ/mol) [47] | Electronegativity (Pauling) [48] | Configuration |
Ca(H2O)82+ | 2.46 | 1.14 | 1.12 | 2.31 | 1.76 ± 0.01 | 1st: 589.8 2nd: 1145.4 3rd: 4912.4 | 1.0 | Square antiprism |
Pb(H2O)62+ | 2.54 | 1.33 | 1.20 | 2.02 | 1.46 ± 0.05 | 1st: 715.6 2nd: 1450.5 3rd: 3081.5 | 2.33 | |
Ni(H2O)62+ | 2.055 | 0.83 | 0.715 | 1.63 | 1.24 ± 0.04 | 1st: 737.1 2nd: 1753.0 3rd: 3395 | 1.91 | Octahedron green |
Al(H2O)63+ | 1.89 | 0.673 | 0.55 | 1.84 | 1.21 ± 0.04 | 1st: 577.5 2nd: 1816.7 3rd: 2744.8 | 1.61 | Octahedron |
It is expected that the selectivity of the resin is enhanced at higher ionic charge and lower volume of the hydrated ions, which is corroborated by the high tendency of COOH group to dissociate and form ionic and coordination bonds [44,45]. Indeed, the sorption selectivity of aqua complex metals is influenced by the coordinative energy of bonds (responsible for the stability of the resulting complex); Mendes et al. [49] draw at a similar conclusion. Therefore, the resin has the best selectivity for aluminium and nickel since they have the smallest hydrated radius, ionic radius and covalent radius. It is also worth noting that the nickel ion has the smallest van der Waals radius among the studied metals, in accordance with its best selectivity.
3.4 Morphostructural characterization
These thermodynamic and structural data on the selective adsorption of the cations upon the S930 resin have been completed by the morphostructural information provided by SEM and EDAX analyses (Figs. 7 and 8) in order to get complementary arguments in supporting the sorption mechanism.
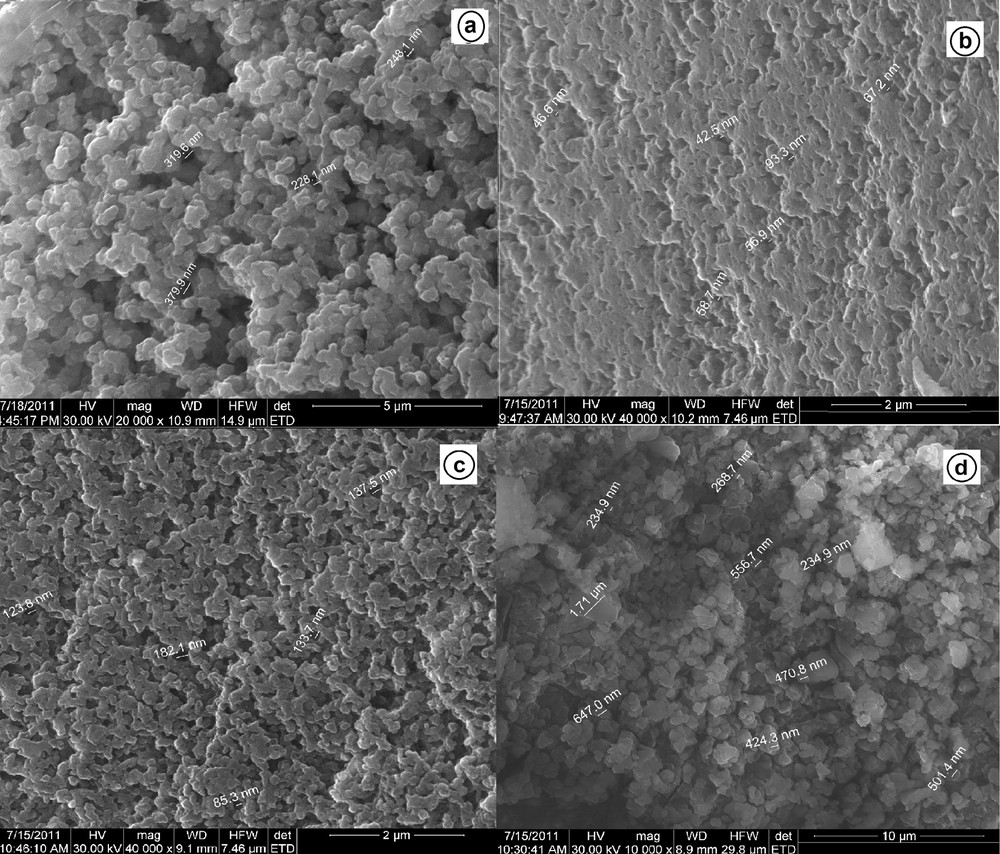
SEM images for S930: (a) dry standard resin; (b) wet standard resin on the surface; (c) after sorption in quaternary systems at pH 3.03; (d) idem at pH = 3.95.
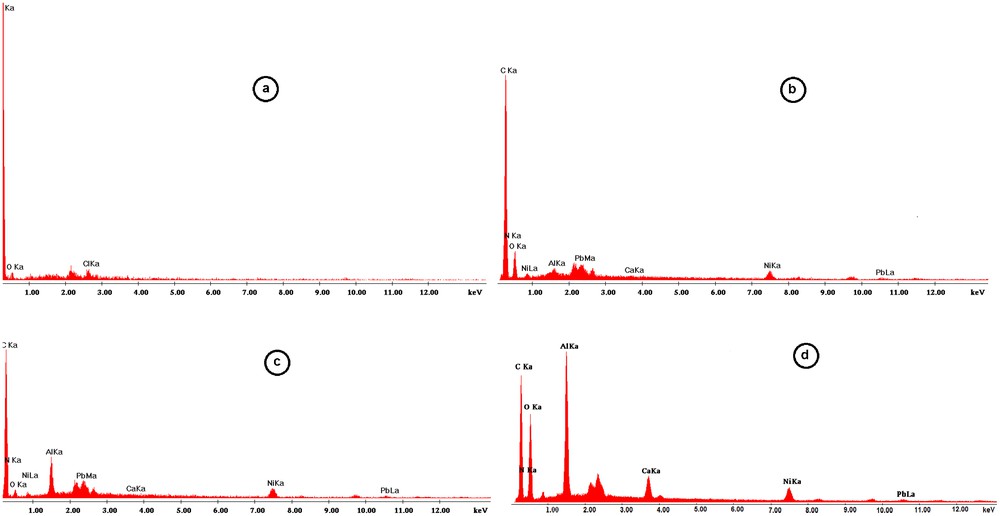
EDAX analysis for S930 resin: (a) standard resin, (b) after sorption in quaternary systems at pH 3.02; (c) idem at pH = 3.95 inside the grain, (d) idem at pH = 3.95 on the grain surface.
The morphologic characterization of dry and wet standard resin before sorption is shown in Fig. 7a and b, respectively. The pore size varied from 200 to 400 nm for the dry resin (Fig. 7a), and between 40 and 100 nm for the wet resin on the surface (Fig. 7b). In case of sorption from the quaternary system at pH 3.03, the pore size is in the 80–200-nm range (Fig. 7c), while at pH = 3.95, the dimensions of the crystals of aluminum salts range from 200 to 1700 nm (Fig. 7d). This observation is in agreement with the previous results, confirming that in quaternary systems the aluminium has precipitated in the 3.95–4.45 pH range, which is similar to the behaviour noticed in mono-component systems.
The elemental analyses provided by EDAX measurements for the reference resin and the resin at pH 3.03 and 3.95 after sorption of the cation mixture is illustrated in Fig. 8.
The reference resin (standard resin) does not contain any heavy metal ions according to Fig. 8a. After contact with the solution containing the quaternary system of heavy metals, one can observe a variation with pH of the amounts of metal ions retained by the resin, Fig. 8b–d, the quantity of aluminum retained by the resin increasing at higher pHs.
Moreover, a difference between the amounts of aluminum retained on the surface and inside the resin grain could be noticed at pH 3.95, being much higher on the grain surface than inside as a result of aluminum microprecipitation, thus, confirming previous results.
4 Conclusion
The simultaneous removal of four cations usually present in wastewater effluents, Ca2+, Ni2+, Pb2+and Al3+, has been investigated by sorption on Purolite® S930 resin. The equilibrium data have been fitted to Langmuir and Freundlich isotherm models, and the pH influence has been studied in details.
The ion exchange capacity for Ca2+, Ni2+, Pb2+ increases relatively linearly in the pH range between 2.02 and 6.5, while for a higher initial concentration of Al3+, the increase of the sorption capacity of the resin is reaching a bigger maximum uptake of 3.66 mequiv/g, much above the total sorption capacity of the resin. This phenomenon has been explained by the fact that Al3+ precipitation starts at pH = 3.95 probably by microprecipitation of metals on the resin surface. Indeed, at pH = 4.45, the determined amount of Al3+ is 2.5 times the maximum capacity of the resin.
It was also observed that the individual sorption capacity for metals is lower in the quaternary system as compared to mono-component systems, as expected, due to the competition of the four cations for the same active sites of the resin.
The highest selectivity of the resin is against Ni2+, followed by Pb2+, while for Al3+ a similar pattern with maximum at pH = 4.45 is noticed, as in the case of a change of sorption capacity in the same pH range, accounted for by microprecipitation of aluminium on the resin lattice which contributes to increase the resin selectivity.
The selectivity of the resin has been also analyzed by means of structural characteristics of metal ions. The best selectivity for aluminium and nickel has been attributed to the smallest hydrated radius, ionic radius and covalent radius; it has to be noted that nickel ion has the smallest van der Waals radius among the studied metals.
These thermodynamic and structural data on the selective adsorption of cations on the S930 resin have been completed by the morphostructural information provided by SEM and EDX analyses, thus, allowing us to obtain complementary arguments supporting the proposed sorption mechanism.