1 Introduction
Quinones are prevalent structural components in various natural products, which are associated with diverse biological activities, including antibacterial, antimalarial, antifungal, antitumor activities [1–5]. The biological activity of the quinones originates from the redox chemistry of the quinone system [6]. A great deal of research has been conducted in recent years on the charge transfer complexes of quinones because of their wide applications ranging from chemistry, medicine to biology [7,8]. Despite the fact that natural products are important sources of new biologically active molecules for drug discovery, their structural complexity and poor availability make greatly difficult natural product-based drug discovery. The discovery of new synthetic methodologies for the preparation of organic compounds is a prime focus of the researches in the field of current organic, bioorganic, and medicinal chemistry [9–12]. In recent years, the concept of privileged medicinal structures or scaffolds that commonly consist of quinone moiety, which contains heteroatoms as substituents, has emerged as one of the guiding principles of drug discovery process [3,13]. Quinone derivatives have been widely investigated for cancer therapy and many drugs containing a quinone moiety, such as anthracyclines, daunorubicin, mitomycin, and saintopin have received clinical approval for cancer treatment [13–16].
Structure–activity relationship of quinone compounds showed that the ring number, the position, and number of heteroatoms in the side chain or inside the ring with various substituents are very crucial to affect the biological activities [17]. Especially, we have known incorporation of a heteroatom such as a sulfur or nitrogen atom into the ring of the quinone skeleton can change the physicochemical properties and create a new pharmacophore with a different biological profile [17]. Chemical structures of biologically important compounds that include the benzoquinone core and sulfur or nitrogen atoms in the side chain or inside the ring with various substituents are shown in Fig. 1 [1,3,11,18–20]. The interesting biological profile resulting from the presence of a heteroatom, sulfur or nitrogen, prompted us to synthesize quinone derivatives possessing nitrogen and sulfur atoms.
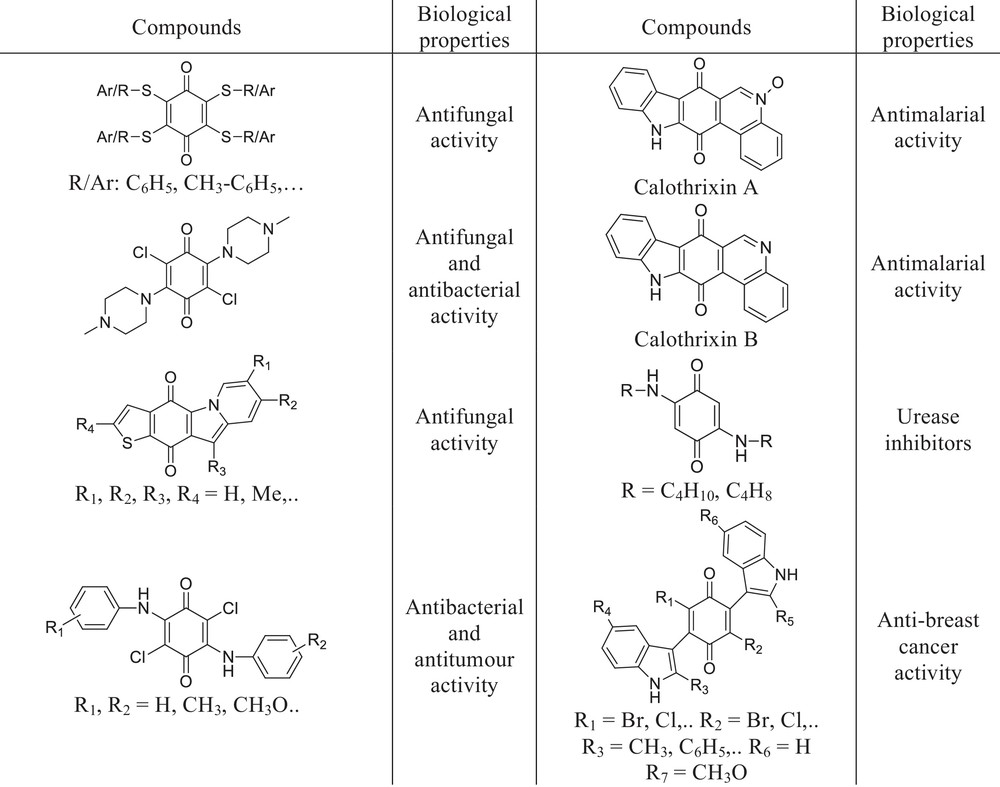
Chemical structures of biologically active 1,4-benzoquinones.
There have been new studies about charge transfer complexes of a number of quinones with a variety of donors [21–23] and the mechanism behind the interaction of quinone with drugs. In recent years, the spectral, thermodynamic, and kinetic aspects of the interaction of 1,4-benzoquinones with variable alkyl chain of alkoxy groups such as 2,3,5-trichloro-6-methoxycyclohexa-2,5-diene-1,4-dione, 2,3,5-trichloro-6-ethoxycyclohexa-2,5-diene-1,4-dione, and 2,3,5-trichloro-6-butoxycyclohexa-2,5-diene-1,4-dione with sulfamethoxazole drug were investigated for understanding the mechanism of the interaction and to characterize the structures of the products formed in these interactions [24].
The reactivity of quinones towards nucleophiles is dependent to a large degree on the π-acceptor character and electron affinity of the quinones. To the best of our knowledge, LCAO-MO calculations for 1,4-benzoquinone have shown that the distribution of π-electron density is very changeable. This class of compounds has a diverse range of activities because of a wide variation in the electron density of quinones [25]. Possible variations in substitution models on the quinone ring affect its capability to accept electrons and so its capacity to reconcile biological reactions [26,27]. As shown in Fig. 2, electron affinity can be increased by appropriate substitution of the quinone. The electron affinity of unsubstituted 1,4-benzoquinone, which has a low value (1.91 eV), can be changed by replacement of hydrogen by other atoms [28].
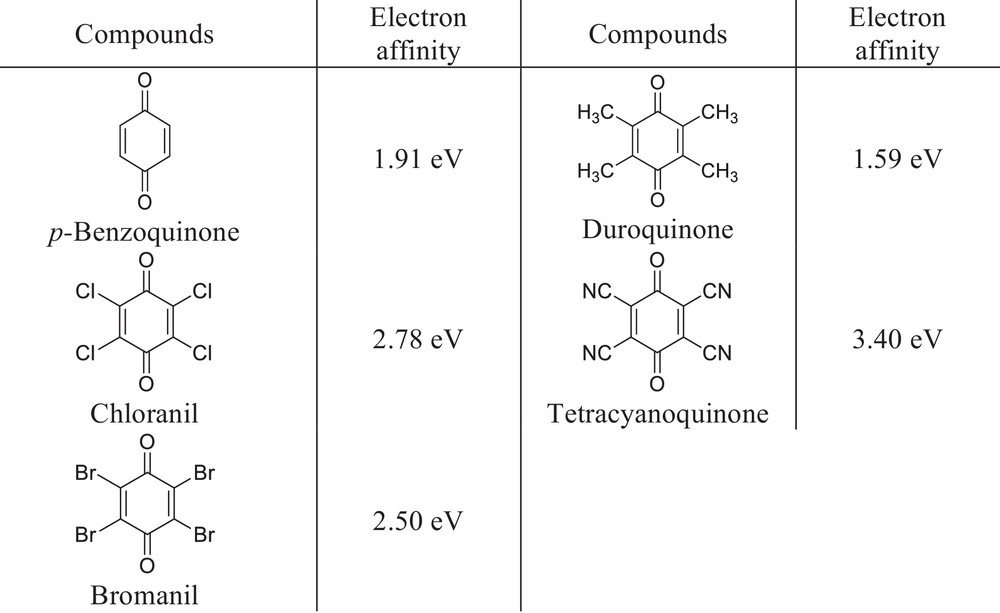
Electron affinity of 1,4-benzoquinones.
We aimed to synthesize new 1,4-benzoquinone compounds, which not only may give promising candidates with respect to biological activity but also may be potent receptors for interaction with drugs. Although there were a lot of studies investigating features and reactions of quinones, there is a notable lack of information about the structural aspects on the nucleophilic substitution reactions of 1,4-quinone derivatives. Additionally, we have focused on nucleophilic reactions of 1,4-quinones and spectroscopic, structural aspects of the reactions of quinones with sulfur and nitrogen nucleophiles. We also observed the behavior of the two isomers of alkoxy, chloro substituted-1,4-benzoquinones on nucleophilic reactions.
2 Results and discussion
1,4-Benzoquinones are known to be easily reacted with various nucleophiles in different conditions [29,30]. Earlier studies show that when chloranil (1) reacts with thiol in the presence of Na2CO3 and ethanol, alcohol can act as a nucleophile along with thiol, and replace chloro atoms [31]. We have observed this situation in the reaction of chloranil (1) with n-octylthiol in the presence of Na2CO3 and ethanol (Scheme 1).
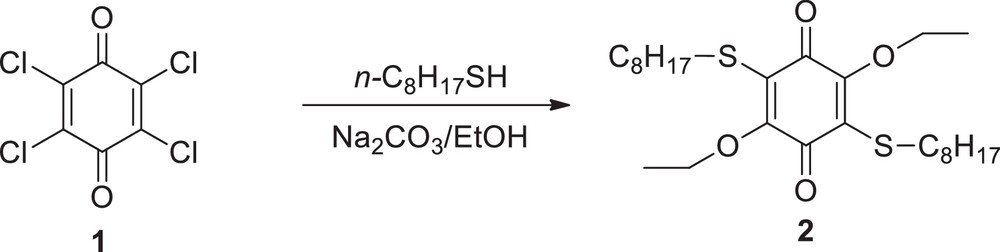
In the beginning of our studies, we studied the chloranil compound to obtain new quinone derivatives but due to high reactivity of chlorine atoms in the structure, a lot of by-products were obtained. This situation suffers from the low yields and serious difficulties in purification. We decided to assist p-chloranil by two ethoxy groups to enhance the regioselectivity towards nucleophiles in order to overcome these problems. In light of such reactions, we decided to synthesize 2,5-dichloro-3,6-diethoxy-1,4-benzoquinone and 2,6-dichloro-3,5-diethoxy-1,4-benzoquinone separately. Investigations concerning how these isomers act towards the different nucleophiles and what structural aspects of the reactions of these compounds were made. Firstly, we synthesized starting materials namely 2,5-dichloro-3,6-diethoxy-1,4-benzoquinone (3) and 2,6-dichloro-3,5-diethoxy-1,4-benzoquinone (4) prepared from chloranil (1) with ethanol in the presence of triethylamine as indicated in the literature [32] (Scheme 2).
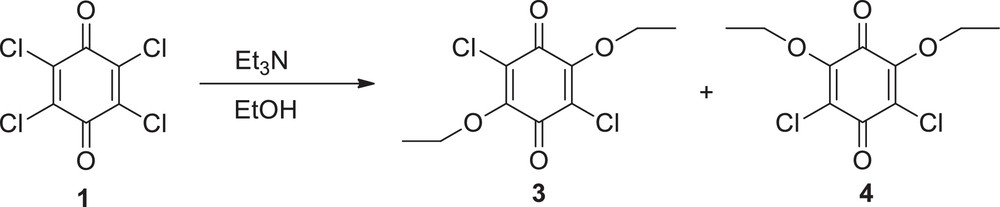
The ethoxy, chloro substituted-1,4-benzoquinones (3, 4) with various thiol and amine nucleophiles (Schemes 3 and 4) gave 5–13 products by nucleophilic substitution reactions. The reactions have been performed with different sulfur and nitrogen nucleophiles in ethanol or CHCl3, in the presence of a base.
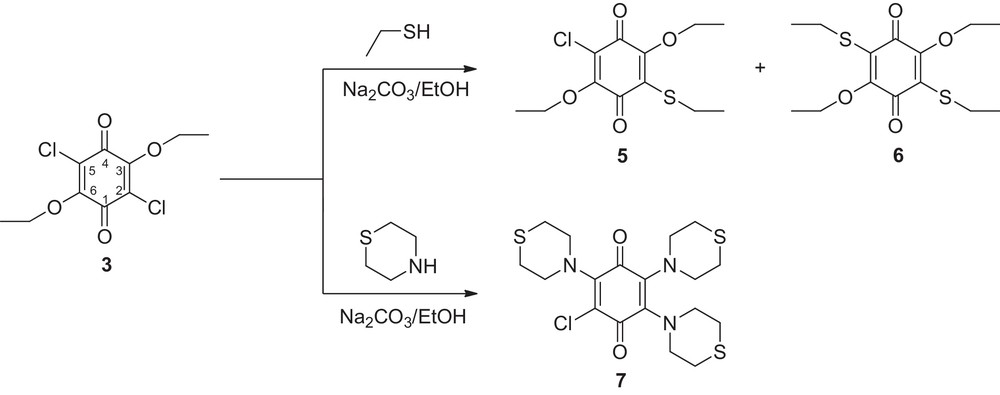
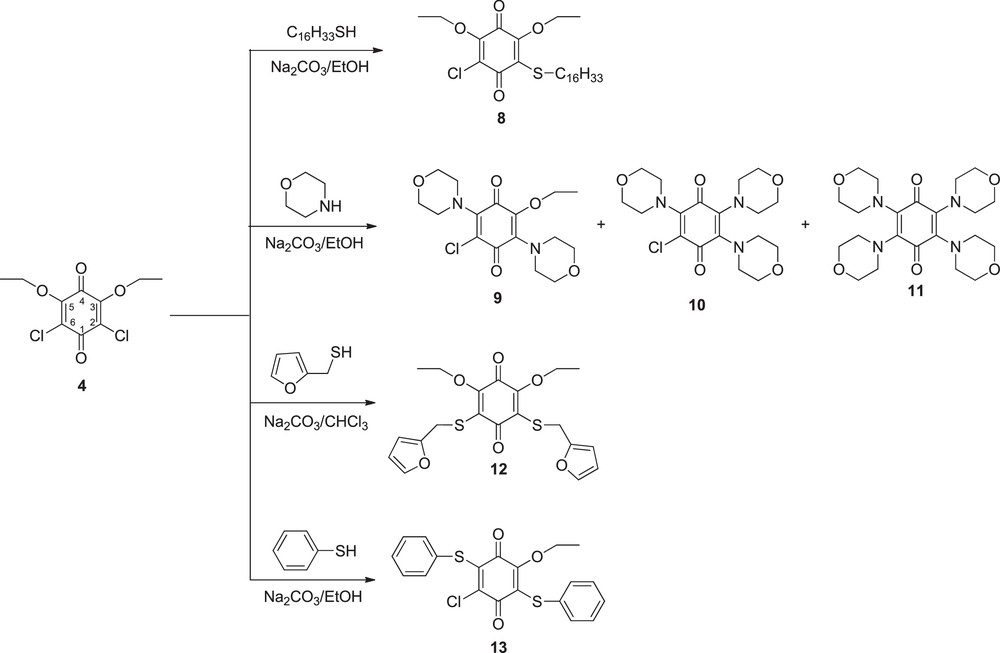
In the reaction of 3 with ethanethiol, the compounds 5 and 6 were obtained in a one-pot fashion. While in the forming compound 5, nucleophile chose to attend 2 position so one chlorine atom exchanged by nucleophile. In this reaction, it has also been determined that the initially formed monoadduct 5 may undergo further nucleophilic addition to give disubstituted adduct 6 resulting in a regioselectivity for the 5 position that contains second chlorine atom. In the reaction of 3 with thiomorpholine, most probably, nucleophile attended firstly in 2 position to give monoadducts and this compound may undergo nucleophilic addition to give trisubstituted adduct 7.
In the 1H NMR spectra, compounds 5 and 6 exhibited the methyl protons of thiol groups in the range 1.18–1.22 ppm and methyl protons of ethoxy groups at 1.31–1.36 as triplet. While the SCH2 protons were observed at 2.99–3.09 ppm, the OCH2 protons were observed around 4.22–4.29 ppm as quartet. 13C spectra of the 5 showed two carbon signals at 174.58 and 176.99 ppm for carbonyl groups, compound 6 gave only one carbon signal at 175.44 ppm due to the symmetry of the molecule. Mass spectra of 5 and 6 confirmed the proposed structure. The molecular ion peak [M]+ was identified at m/z 290.14 with strong abundance (100%) for 5 and at m/z 316.33 for 6.
In the reaction of 4, which is the isomer structure of 3 with hexadecanethiol, compound 8 was obtained by the nucleophilic substitution reaction in the 2 position. It was also observed that the nucleophile replaced the chlorine atom in 2 position, like in the formation of the 5 structure. The reaction of 4 with morpholine gave three products 9, 10, and 11 that is a known compound [33]. We defined by the construction of 9 that nucleophile preferred one chlorine atom in 2 position and ethoxy group in 5 position. 9 may undergo nucleophilic addition to give trisubstituted adduct 10 and tetrasubstituted adduct 11 [33]. It was determined in the structure of 12 that the nucleophile substituted two chlorine atoms. In the formation of 13, it was seen that the nucleophile chose one chlorine atom in 2 position and an ethoxy group in 5 position.
In the 1H NMR spectra of 8, the methyl protons of thiol groups have shown typical ppm values within the range 0.80–0.82 as triplet and methyl protons of ethoxy groups at 1.16–1.24 as multiplet. While the SCH2 protons were observed at 3.01–3.04 ppm, the methylene protons of ethoxy group were observed around 4.21–4.43 ppm as quartet. 13C spectra of the 8 showed two carbon signals at 174.58, 176.97 ppm for carbonyl groups. In the mass spectra of 8 the molecular ion peak [M]+ was identified at m/z 486.75 with strong abundance (100%) for 8.
9 gave methyl protons of ethoxy groups at 1.27–1.30 ppm as multiplet, NCH2 protons of morpholine ring at 3.40–3.51 ppm, OCH2 protons of morpholine ring at 3.71–3.76 ppm and methylene protons of ethoxy groups at 3.84–3.88 ppm in the 1H NMR spectra. In the compound 10, while NCH2 protons of morpholine ring at 3.05–3.48 ppm, OCH2 protons of morpholine ring at 3.66–3.75 ppm. Carbonyl groups in the 13C spectra showed two carbon signals at 178.41 and 216.2 ppm for 9, and 178.73 and 180.77 ppm for 10.
Compounds 12 gave methyl protons of ethoxy groups at 1.25–1.28 ppm as triplet, CH2 protons of ethoxy groups at 4.16–4.21 ppm as multiplet, CHproton at 6.04–6.19 ppm as doublet, OCH proton at 7.22–7.23 as doublet. 13C spectra of the 12 showed two carbon signals at 174.41 and 180.51 ppm for carbonyl groups.
In the 1H NMR spectra of 13, methyl protons of ethoxy groups at 0.68–0.94 as multiplet. The CH2 protons of ethoxy groups were observed around 3.95–4.06 ppm as multiplet and aromatic protons of phenyl ring at 6.91–7.30 as multiplet. 13C spectra of the 13 showed two carbon signals at 174.16 and 178.72 ppm for carbonyl groups. The molecular ion peak [M + Na]+ was identified at m/z 425.0 with strong abundance (100%) for 13.
3 Conclusions
There can be no doubt that the quinone compounds deserve the great prominence by exhibiting a broad spectrum of biological activities and forming the charge transfer complexes that have enormous applications ranging from sensors, magnetic materials to chemistry of drugs. Even if conjugate addition reaction of p-quinones is well studied, many aspects regarding the regioselectivity observed in the reaction of substituted quinones still remain obscure. In the context of our ongoing interest to prepare new derivatives of quinones by nucleophilic substitution and characterize these compounds, differences in regioselectivity were observed. It can be concluded that nucleophiles choose chlorine atoms to replace in the 2 position both isomer structures in the formation of monoadducts 5 and 8 with thiol nucleophiles, whereas nucleophiles usually prefer 5 position for second substitution in the formation of bis adducts 2, 6, and 9. The trisubstituted products 7 and 10 were obtained by substitution in 2, 3, and 5 position and nucleophile preferred the ethoxy groups for third substitution in both isomers. In conclusion, we have synthesized a series of new 1,4-benzoquinone derivatives that are the promising candidates with respect to biological activity and as new receptors for drugs.
4 Experimental
All chemicals were reagent grade and used without further purification. Progress of the reactions and purity of compounds were monitored by thin layer chromatography (TLC), which was performed on Merck silica gel plates (60F254), and compounds were detected with ultraviolet light (254 nm). Products were isolated by column chromatography on silica gel (Fluka silica gel 60, particle size 63–200 μm). Melting points were measured on a Buchi B-540 melting point apparatus and are uncorrected. 1H NMR (500 MHz) and 13C NMR (125 MHz) spectra were recorded in CDCl3 on a Varian UNITY INOVA spectrometer. Infrared (IR) spectra were recorded on a Thermo Scientific Nicolet 6700 spectrometer. Microanalyses were performed on a Carlo Erba 1106 elemental analyzer. Mass spectra were obtained on a Thermo Finnigan LCQ Advantage MAX LC/MS/MS spectrometer using ion-trap mass analyzer for either APCI or ESI source.
4.1 General procedure for the synthesis of new derivatives of 1,4-benzoquinones
Sodium carbonate (1.52 g) was dissolved in ethanol or chloroform (68.75 mL) and into the resulting solution, firstly ethoxy, chloro 1,4-benzoquinone and then thiol/amine were added in small portions at room temperature. The mixture was stirred until completion of the reaction (TLC). The residue was extracted with chloroform. The organic layer was separated and washed with water (4 × 30 mL), and dried with Na2SO4. The solvent was evaporated and the residue was purified by column chromatography on silica gel.
4.1.1 2,5-diethoxy-3,6-bis(octylthio)cyclohexa-2,5-diene-1,4-dione (2)
Compound 2 was synthesized by the reaction of 1.845 g (7.5 mmol) p-chloranil (1) with 4.39 g (30 mmol) octanethiol.
2: yield 0.54 g (15%); brown oil; IR (Film) ν 2988 (CHaliphatic), 1680 (CO), 1475 (CC) cm−1; 1H NMR (499.74 MHz, CDCl3) δ 0.85–0.89 (m, 6H, 2CH3CH2S), 1.23–1.67 (m, 30H, 12CH2; 6H, 2CH3CH2O), 2.61–3.06 (m, 4H, 2CH3CH2S), 4.12–4.37 (m, 4H, 2CH3CH2O); 13C NMR (125 MHz, CDCl3) δ 13.86, 14.27, 26.72, 69.48, 124.65, 129.40, 154.36, 178.54; MS m/z (%): 486.3 (M+), C26H44O4S2 (M, 484.77); anal. calcd. for C26H44O4S2: C 64.42, H 9.15; found: C, 64.11, H 9.18.
4.1.2 2-Chloro-3,6-diethoxy-5-(ethylthio)cyclohexa-2,5-diene-1,4-dione (5) and 2,5-diethoxy-3,6-bis(ethylthio)cyclohexa-2,5-diene-1,4-dione (6)
Compounds 5 and 6 were synthesized by the reaction of 0.150 g (0.57 mmol) 2,5-dichloro-3,6-diethoxy-1,4-benzoquinone (3) with 0.035 g (0.57 mmol) ethanethiol.
5: yield 0.041 g (25%); brown oil; IR (Film) ν 2945 (CHaliphatic), 1650 (CO), 1500 (CC) cm−1; 1H NMR (499.74 MHz, CDCl3) δ 1.19–1.22 (t, J = 7.32, 3H, 1CH3CH2S), 1.32–1.36 (t, J = 6.83, 6H, 2CH3CH2O), 3.05–3.09 (q, J = 7.32, 2H, 1CH3CH2S), 4.22–4.26 (q, J = 6.83, 2H, 1CH3CH2O), 4.38–4.43 (q, J = 7.32, 2H, 1CH3CH2O); 13C NMR (125 MHz, CDCl3) δ 14.18, 14.70, 14.86, 26.60, 69.03, 69.50, 124.87, 130.60, 152.25, 153.07, 174.58, 176.99; MS m/z (%): 290.14 (M+), C12H15ClO4S (M, 290.76); anal. calcd. for C12H15ClO4S: C 49.57, H 5.20; found: C, 49.51 H 5.16.
6: yield 0.088 g (40%); dark brown oil; IR (Film) ν 2973 (CHaliphatic), 1645 (CO), 1489 (CC) cm−1; 1H NMR (499.74 MHz, CDCl3) δ 1.18–1.21 (t, J = 7.32, 6H, 2CH3CH2S), 1.31–1.34 (t, J = 7.32, 2CH3CH2O), 2.99–3.04 (q, J = 7.32, 4H, 2CH3CH2S), 4.23–4.30 (q, J = 6.83, 4H, 2CH3CH2O); 13C NMR (125 MHz, CDCl3) δ 15.42, 15.98, 27.71, 70.06, 131.84, 154.99, 175.44; MS m/z (%): 316.33 (M+), C14H20O4S2 (M, 316.44); anal. calcd. for C14H20O4S2: C 53.14, H 6.37; found: C 53.32, H 6.20.
4.1.3 2-Chloro-3,5,6-trithiomorpholinocylohexa-2,5-diene-1,4-dione (7)
Compound 7 was synthesized by the reaction of 0.10 g (0.38 mmol) 2,5-dichloro-3,6-diethoxy-1,4-benzoquinone (3) with 0.078 g (0.76 mmol) thiomorpholine.
7: yield 0.084 g (50); black oil; IR (Film) ν 2954 (CHaliphatic), 1655 (CO), 1493 (CC) cm−1; 1H NMR (499.74 MHz, CDCl3) δ 2.67–2.69 (t, J = 4.88, 12H, 6CH2S), 3.51–3.53 (t, J = 4.88, 12H, 6CH2N); 13C NMR (125 MHz, CDCl3) δ 27.12, 52.36, 121.32, 146.84, 172.9, 179.22; anal. calcd. for C18H24ClN3O2S3: C 48.47, H 5.42; found: C 48.24 H 5.37.
4.1.4 2-Chloro-3,5-diethoxy-6-(hexadecylthio)cyclohexa-2,5-diene-1,4-dione (8)
Compound 8 was synthesized by the reaction of 0.180 g (0.68 mmol) 2,6-dichloro-3,5-diethoxy-1,4-benzoquinone (4) with 0.176 g (0.68 mmol) hexadecanethiol.
8: yield 0.2 g (60%); brown oil; IR (Film) ν 2946 (CHaliphatic), 1663 (CO), 1476 (CC) cm−1; 1H NMR (499.74 MHz, CDCl3) δ 0.80–0.82 (t, J = 6.83, 3H, 1CH3), 1.16–1.24 (m, 28H, 14CH2), 1.32–1.36 (t, J = 7.32, 6H, 2CH3CH2O), 3.01–3.04 (t, J = 7.32, 2H, 1CH2S), 4.21–4.25 (q, J = 6.83, 2H, 1CH3CH2O), 4.34–4.43 (q, J = 7.32, 2H, 1CH3CH2O); 13C NMR (125 MHz, CDCl3) δ 13.10, 14.71, 14.86, 21.68, 27.66, 28.11, 28.35, 28.46, 28.55, 28.64, 28.67, 28.69, 29.12, 30.91, 32.32, 69.00, 69.48, 124.88, 131.10, 152.24, 153.07, 174.58, 176.97; MS m/z (%): 486.75 (M+), C26H43ClO4S (M, 487.14); anal. calcd. for C26H43ClO4S: C 64.11, H 8.90; found: C 64.24 H 8.87.
4.1.5 2-Chloro-5-ethoxy-3,6-dimorpholinocyclohexa-2,5-diene-1,4-dione (9), 2-chloro-3,5,6-trimorpholinocyclohexa-2,5-diene-1,4-dione (10), and 2,3,5,6-tetramorpholinocyclohexa-2,5-diene-1,4-dione (11)
Compounds 9, 10, and 11 were synthesized by the reaction of 0.100 g (0.38 mmol) 2,6-dichloro-3,5-diethoxy-1,4-benzoquinone (4) with 0.066 g (0.76 mmol) morpholine.
9: yield 0.043 g (32%); brown oil; IR (Film) ν 2978 (CHaliphatic), 1649 (CO), 1496 (CC) cm−1; 1H NMR (499.74 MHz, CDCl3) δ 1.27–1.30 (t, J = 7.32, 3H, 1CH3CH2O), 3.40–3.42 (t, J = 4.88, 4H, 2CH2N), 3.49–3.51 (t, J = 4.88, 4H, 2CH2N), 3.71–3.76 (t, J = 4.88, 8H, 4CH2O), 3.84–3.88 (q, J = 7.32, 2H, 1CH3CH2O); 13C NMR (125 MHz, CDCl3) δ 15.51, 51.68, 52.01, 67.71, 67.80, 116.20, 140.07, 141.17, 147.31, 178.41, 216.2; MS m/z (%): 356.82 (M+), C16H21ClN2O5 (M, 356.80); anal. calcd. for C16H21ClN2O5 C 53.86, H 5.93; found: C 53.78, H 5.90.
10: yield 0.045 g (30%); dark green oil; IR (Film) ν 2974 (CHaliphatic), 1651 (CO), 1489 (CC) cm−1; 1H NMR (499.74 MHz, CDCl3) δ 3.05–3.07 (t, J = 4.88, 4H, 2CH2N), 3.38–3.40 (t, J = 4.88, 4H, 2CH2N), 3.46–3.48 (t, J = 4.88, 4H, 2CH2N), 3.66–3.68 (t, J = 4.88, 4H, 2CH2O) 3.71–3.75 (m, 8H, 4CH2O); 13C NMR (125 MHz, CDCl3) δ 50.78, 52.07, 67.76, 67.77, 116.94, 134.59, 144.61, 148.05, 178.73, 180.77; MS m/z (%): 420.24 (M + Na+), C18H24ClN3O5 (M, 397.85); anal. calcd. for C18H24ClN3O5 C 54.34, H 6.08; found: C 54.12, H 6.15.
11: yield 0.034 g (20%); brown oil [33]; IR (Film) ν 2925 (CHaliphatic), 1672 (CO), 1479 (CC) cm−1; MS m/z (%): 449.70 (M+), C22H32N4O6 (M, 448.51); anal. calcd. for C22H32N4O6 C 58.91, H 7.19; found: C, 58.84 H 7.08.
4.1.6 2,6-Bis((furan-2-yl)methylthio)-3,5-diethoxycyclohexa-2,5-diene-1,4-dione (12)
Compound 12 was synthesized by the reaction of 0.19 g (0.71 mmol) 2,6-dichloro-3,5-diethoxy-1,4-benzoquinone (4) with 0.16 g (1.42 mmol) furanthiol.
12: yield 0.11 g (38%); brown oil; IR (Film) ν 2961 (CHaliphatic), 1656 (CO), 1549 (CC) cm−1; 1H NMR (499.74 MHz, CDCl3) δ 1.25–1.28 (t, J = 7.32, 6H, 2CH3CH2O), 4.16–4.21 (m, 8H; 4H, 2CH3CH2O, 4H, 2SCH2), 6.04–6.05 (d, J = 2.44, 2H, CH), 6.17–6.19 (t, J = 2.68, 2H, CH), 7.22–7.23 (d, 2H, OCH); 13C NMR (125 MHz, CDCl3) δ 14.67, 28.82, 69.11, 107.26, 109.53, 128.49, 141.35, 149.88, 154.95, 174.41, 180.51; MS m/z (%): 443.2 (M + Na+), C20H20O6S2 (M, 420.5); anal. calcd. for C20H20O6S2 C 57.13, H 4.79; found: C 57.48, H 4.71.
4.1.7 2-Chloro-5-ethoxy-3,6-bis(phenylthio)cyclohexa-2,5-diene-1,4-dione (13)
Compound 13 was synthesized by the reaction of 0.19 g (0.71 mmol) 2,6-dichloro-3,5-diethoxy-1,4-benzoquinone (4) with 0.078 g (0.71 mmol) phenylthiol.
13: yield 0.041 g (30%); brown oil; IR (Film) ν 3025 (CHaromatic), 1657 (CO), 1478 (CC) cm−1; 1H NMR (499.74 MHz, CDCl3) δ 0.68–0.94 (m, 3H, 1CH3CH2O), 3.95–4.06 (m, 2H, 1CH3CH2O), 6.91–7.30 (m, 10H, 2 C6H5); 13C NMR (125 MHz, CDCl3) δ 15.28, 46.92, 128.13, 128.93, 132.33, 152.48, 174.16, 178.72, MS m/z (%): 425.0 (M + Na+), C20H15ClO3S2 (M, 402.9); anal. calcd. for C20H15ClO3S2 C 59.62, H 3.75; found: C, 59.32 H 3.87.