1 Introduction
The chemistry of heterocycles has acquired immense importance in recent years. Tetrazoles are nitrogen-rich compounds, covering a wide range of applications, such as in material sciences, catalysis, information recording systems, explosives, and possible application in high-energy chemistry [1]. Tetrazole derivatives are well known compounds with a high level of biological activity, such as anti-inflammatory properties, antibiotic, antagonists, antihypertensive, and antiviral activity; they are used in pharmacomodulation because of their bioisostere/carboxylic acid properties [2].
The conventional methods for the synthesis of 5-substituted tetrazoles suffer from drawbacks such as the use of strong Lewis acids, or expensive and toxic metals, and the in situ-generated hydrazoic acids, which are highly toxic and explosive, or severe reaction conditions [3,4]. In general, the most direct and versatile method for the synthesis of 5-substituted 1H-tetrazoles is via [3 + 2] cycloaddition of azide to nitriles. Various homogeneous catalysts such as AlCl3 [5], BF3·OEt2 [6], TBAF [7], Pd(OAc)2/ZnBr2 [8a], Yb(OTf)3 [8b], Zn(OTf)3 [8c], and Pd(PPh3)4 [8d] were used for the synthesis of tetrazoles. However, a drawback of these homogeneous catalytic processes is the difficulty in separation and recovery of the catalysts. Therefore, heterogeneous alternatives are highly desirable and have attracted increasing attention. Heterogeneous catalysis is particularly attractive as it allows the production and ready separation of large quantities of products with the use of a small amount of catalyst. Recently several heterogeneous catalytic systems [9,20] using nanocrystalline ZnO [9a], Zn/Al hydrotalcite [9b], Zn hydroxyapatite [9c], Cu2O [10a], FeCl3–SiO2 [10b], CdCl2 [10c], γ-Fe2O3 [10d], BaWO4 [10e], natural natrolite zeolite [10f], mesoporous ZnS nanospheres [11], CoY Zeolite [12], zeolite and sulfated zirconia [13], CuFe2O4 nanoparticles [14], SSA [15], ZrOCl2.8H2O [16], and montmorillonite K-10 clay [17] were reported. Most of these methods suffer from disadvantages such as the fact they require a large excess of sodium azide, higher temperatures, and longer reaction times. In spite of this, in most of previously reported methods, the cycloaddition is too slow to be synthetically useful. Thus, the development of more sustainable methods is desirable for the synthesis of tetrazoles.
An important objective of chemistry is to adapt classical processes so that pollution effects are kept to a minimum, with both reduction in energy and consumption of raw materials. Solid acid catalysts play a prominent role in organic synthesis under heterogeneous conditions. Recently we have reported the synthesis and characterization of nano TiO2/SO42− having high specific surface area with excellent catalytic activity towards organic reactions [18]. Now, in the present study, we exploit nano TiO2/SO42− for the synthesis of 5-substituted-1H-tetrazoles from a wide variety of aromatic nitriles with sodium azide in DMF (Scheme 1).

Synthesis of 5-substituted-1H-tetrazoles from a wide variety of aromatic nitriles with sodium azide in DMF.
2 Results and discussion
In our previous papers [18], we have reported the preparation and characterization of nano TiO2/SO42− and its catalytic activity for the synthesis of fatty amides and Beckmann rearrangement. So, the textural properties of nano TiO2/SO42−, used in the present study, are given in brief. Analysis of the SEM and TEM images of this catalyst reveals that particles are uniformly distributed in spherical shape. Hence, SO42− ion modification preferably retarded the aggregation. This facilitates the organic transformations. It was found from XRD and TEM that the catalyst is in nano particles (40–50 nm) and that the anatase and rutile phases of TiO2 have been confirmed; and sulfation of the TiO2 does not change the phases. The surface area was found to be 218 m2/g.
In continuation of our recent work on applications of heterogeneous catalysts for the development of synthetic methodologies, herein we report a simple, green, and efficient protocol for the synthesis of tetrazoles with environmentally friendly nano TiO2/SO42− as a catalyst.
To optimize the reaction conditions, benzonitrile (1a) and sodium azide (2) were used as test substrates to yield 5-phenyl-1H-tetrazole (3a), and the results are summarized in Table 1. The influence of experimental parameters such as solvent, temperature, amount of catalyst, and reaction time were investigated. The solvent has a prominent influence on the yield of product 3a (Table 1, entries 2–8). The experimental results show that among the solvents tested (using 0.2 g of nano TiO2/SO42−),–gave a good yield of 3a but with a long reaction time whereas DMF is the best one in the synthesis of tetrazole 3a (entry 8). Other solvents are not suitable for this reaction.
Screening of reaction parameters for the formation of 5-phenyl-1H-tetrazole 3a.
Entry | Amount of catalyst (g) | Solvent | Temperature (°C) | Time (h) | Yield (%)a |
1 | 0.2 | None | 120 | 12 | 0 |
2 | 0.2 | H2O | Reflux | 12 | 0 |
3 | 0.2 | EtOH | Reflux | 12 | 0 |
4 | 0.2 | CH3CN | Reflux | 12 | 0 |
5 | 0.2 | THF | Reflux | 12 | 0 |
6 | 0.2 | Toluene | Reflux | 12 | 0 |
7 | 0.2 | – | 120 | 10 | 90 |
8 | 0.20 | DMF | 120 | 1.5 | 95 |
9 | 0.20 | DMF | 100 | 2 | 95 |
10 | 0.20 | DMF | 80 | 3 | 90 |
11 | 0.20 | DMF | r.t | 12 | 0 |
12 | 0.10 | DMF | 120 | 2 | 90 |
13 | 0.05 | DMF | 120 | 4 | 90 |
14 | None | DMF | 120 | 12 | 50 |
15 | 0.2 | DMF | 120 | 1.5 | 93b |
a Isolated yield.
b Yields after third cycle.
By increasing the temperature from 25 to 80 °C and 120 °C, the yield of product 3a was also increased (Table 1, entries 8–11). The effect of the amount of catalyst on the reaction was also evaluated (entries 12 and 13). It is reasonable to assume that the interaction of the reactant molecules with the active sites of the nano sulfated titania catalyst is commensurate with an increase in the amount of catalyst in the reaction mixture and hence the availability of a higher number of active sites. The controlled reaction conducted under identical conditions devoid of nano TiO2/SO42− gave a poor yield of addition product, despite prolonged reaction time (entry 14). Nano TiO2/SO42− was recovered quantitatively by simple centrifugation and reused for three cycles with consistent activity (entry 15).
Under the optimized reaction conditions, we chose a variety of structurally divergent benzonitriles to understand the scope and generality of the nano sulfated titania promoted [3 + 2] cycloaddition reaction to form 5-substituted 1H-tetrazoles and the results are presented in Table 2. The aromatic benzonitriles give moderate to good yields (Table 2, entries 1–14). Better results were obtained with nitriles containing an electron-withdrawing group at the para- or meta-position compared to an electron-donating substituent. Heteroaromatic nitriles such as 2-pyridinecarbonitrile and 4-pyridinecarbonitrile gave the corresponding tetrazoles in excellent yields (Table 2, entries 5 and 6). Interestingly, o-, m-, and p-dicyanobenzenes afforded mono-addition products (entries 2–4), whereas with Zn(II) salts di-addition products were reported in the literature [19]. All synthesized tetrazoles were characterized by 1H NMR and 13C NMR, and FT–IR spectroscopy.
Synthesis of 5-substituted-1H-tetrazoles using nano TiO2/SO42−a.
Entry | Nitrile | Tetrazole | Time (h) | Yield (%)b |
1 | 1.5 | 97 | ||
2 | 2 | 90 | ||
3 | 0.75 | 95 | ||
4 | 0.75 | 95 | ||
5 | 1.5 | 95 | ||
6 | 6 | 90 | ||
7 | 2:20 | 95 | ||
8 | 2:20 | 95 | ||
9 | 5 | 90 | ||
10 | 0.75 | 95 | ||
11 | 2 | 92 | ||
12 | 2 | 85 | ||
13 | 7 | 60 | ||
14 | 7 | 60 |
a Reaction conditions: nitrile (1.0 mmol), NaN3 (1.0 mmol), nano TiO2/SO42− (0.2 g, 0.015 mol%), in an oil bath at 120 °C, in DMF.
b Isolated yield.
A comparison of the present protocol, using nano TiO2/SO42−, with selected previously known protocols is collected in Table 3 to demonstrate that the present protocol is indeed superior to several of the other protocols. According to Table 3, the synthesis of 5-phenyl-1H-tetrazole 3a is completed in 1.5 h at 120 °C in 97% isolated yield by using NaN3/benzonitrile in molar ratio 1:1, by using the present protocol. Most of the other protocols listed either take a longer reaction time for completion or require prior preparation of the catalyst support, whereas they do not use NaN3/benzonitrile in molar ratio 1:1 with generally reduced isolated yields. Thus, nano TiO2/SO42− was found to be the better choice for this reaction.
Synthesis of 5-phenyl-1H-tetrazoles 3a catalyzed by various solid acid catalystsa.
Entry | Catalyst | Amount of catalyst | Molar ratio of NaN3 to benzonitriles | Time (h) | Yield (%)b [ref] |
1 | Nano TiO2/SO42− | 0.2 g | 1:1 | 1:30 | 97 |
2 | CuFe2O4 | 40 mol% | 1.5:1 | 12 | 82 [14] |
3 | γ-Fe2O3 | 0.20 g | 5.3:2.5 | 36 | 81 [10d] |
4 | CoY | 0.02 g | 2:1 | 14 | 90 [12] |
5 | Zeolite | 0.05 g | 6:2 | 24 | 90 [13] |
6 | Sulfated ZrO2 | 0.05 g | 6:2 | 24 | 90 [13] |
7 | FeCl3–SiO2 | 0.09 g | 3:2 | 12 | 79 [10b] |
8 | Nano ZnO | 0.1 g | 2.75:2.5 | 14 | 72 [9a] |
9 | Zn/Al hydrotalcite | 0.1 g | 3:2 | 12 | 84 [9b] |
a Reaction conditions: at 120 °C, in DMF.
b Isolated yield.
A plausible mechanism for the synthesis of tetrazoles in the presence of nano TiO2/SO42− is shown in Scheme 2. Commonly, in sulfated metallic oxides, there may be many Lewis acid sites as well as Brønsted acid sites. The superacidity of these materials is attributed to the Brønsted acid sites, created or already existing, whose acidity is increased by the presence of neighboring strong Lewis acid sites. The strength of these Lewis acid sites is due to an inductive effect exercised by sulfate on the metallic cation, which becomes more deficient in electrons [19], as seen in Scheme 2. Thus, Lewis acid sites of nano TiO2/SO42− coordinated readily to the nitrile, and this is the dominant factor influencing [3 + 2] cycloaddition. It should be noted that, previously, Sharpless et al. [20] reported that coordination of nitrile substrate to the Lewis acidic Zn2+ is the source of the catalytic effect in the formation of 1H-tetrazoles, which is supported by model calculations using density functional theory. Subsequently, nucleophilic attack by azide followed by hydrolysis affords tetrazole.
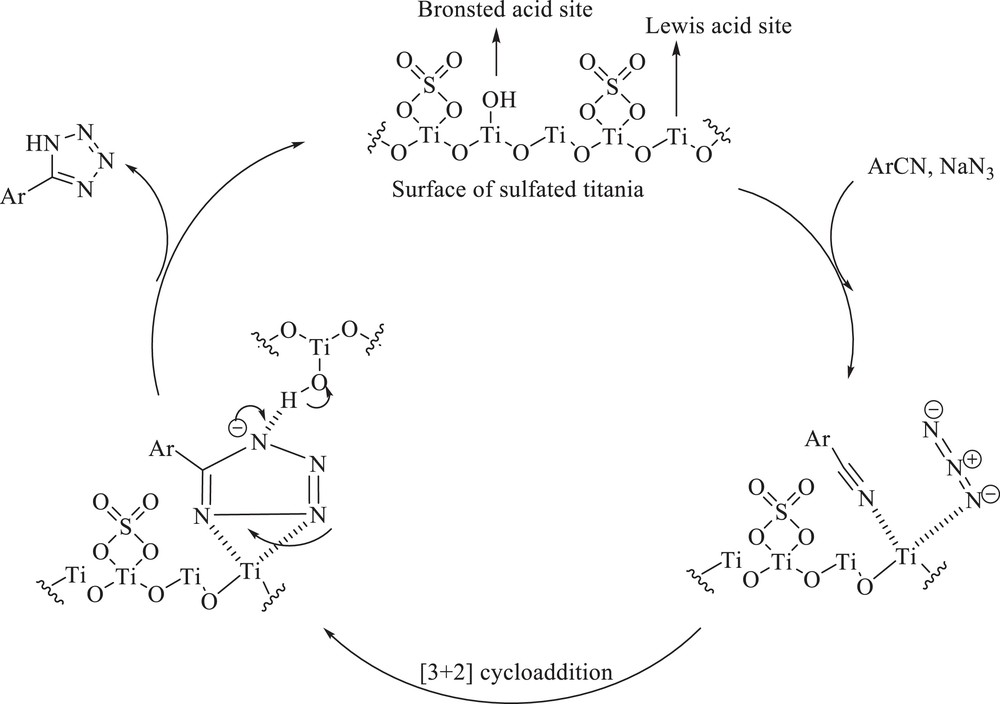
Two-step mechanism for the formation of 5-substituted-1H-tetrazoles.
In conclusion, we have developed a simple, ecofriendly, and efficient method for the preparation of 5-substituted-1H-tetrazoles via [3 + 2] cycloaddition using nano TiO2/SO42− as a heterogeneous catalyst. Various nitriles reacted with NaN3 in DMF to yield the corresponding 5-substituted-1H-tetrazoles with moderate to good yields. The catalyst can be readily recovered and reused. The significant advantages of this methodology are high yields, simple methodology, easy work-up, a simple work-up procedure, and easy preparation and handling of the catalyst. The catalyst can be recovered by filtration and reused. This methodology may find widespread use in organic synthesis for the preparation of 5-substituted-1H-tetrazoles.
3 Experimental
3.1 Catalyst preparation
Nano TiO2/SO42− powder was prepared by a sol–gel process. Titanium isobutoxide (98% Fluka) was used as the source of TiO2. An amount of 14.3 mL of Ti(OC4H9)4 was hydrolyzed in 150 mL of water containing 1.25 mL of nitric acid 65% (Merck); the aqueous solution was stirred continuously at room temperature for 2 h to form a highly dispersed sol, and then the sol was concentrated and dried at 60 °C. Sulfation was done using a 0.5 M sulfuric acid solution (2.0 g·mL−1). The samples, after having been dried for 2 h at 110 °C, were calcined for 5 h at 500 °C.
3.2 General procedure for the synthesis of tetrazoles using nano TiO2/SO42−
In a round-bottom flask, benzonitrile (1 mmol), sodium azide (1 mmol), and nano TiO2/SO42− (0.2 g) were charged. Then the reaction mixture was stirred in distilled dimethylformamide (1 mL) at 120 °C. The progress of the reaction was followed by TLC (75:25 ethyl acetate:n-hexane). After completion of the reaction, the catalyst was separated by centrifugation, washed with doubly distilled water and acetone, and the centrifugate was treated with 5 N HCl (20 mL) under vigorous stirring. The aqueous solution finally obtained was extracted twice with ethyl acetate. The combined organic phase was washed with water and concentrated to precipitate the crude crystalline solid. All products were characterized by NMR, IR, mass spectra, and CHN analysis and the data for the known compounds were found to be identical with the literature. The complete spectroscopic data are described in the supporting information.
4 5-Phenyl-1H-tetrazole (3a)
Yield: 97%. White solid. M.p. 215–216 °C (lit. [11] 215–216 °C). 1H NMR (250 MHz, DMSO-d6): δ = 3.92 (brs, 1 H), 7.55–7.61 (m, 3 H), 8.04–8.10 (m, 2 H) ppm. 13C NMR (62.9 MHz, DMSO-d6): δ = 124.1, 127.0, 129.4, 131.2, 155.2 ppm. IR (KBr): ν = 3419, 2924, 2827, 2717, 2656, 1620, 1600, 1460, 1350, 1383, 1163, 1057, 765 cm−1.
5 2-(1H-Tetrazol-5-yl)benzonitrile (3b)
Yield: 90%. White solid. M.p. 208–210 °C (lit. [12] 212–214 °C). 1H-NMR (250 MHz, DMSO-d6): δ = 5.02 (brs, 1 H), 7.74 (m, 1 H), 7.92 (m, 1 H), 8.05 (d, J = 8.04 Hz, 2 H) ppm. 13C-NMR (62.9 MHz, DMSO-d6): δ = 110.1, 117.2, 127.4, 129.6, 131.3, 133.7, 134.8, 155.4 ppm. IR (KBr): ν = 3096, 2531, 2110, 2023, 1632, 1436, 845 cm−1.
6 3-(1H-Tetrazol-5-yl)benzonitrile (3c)
Yield: 95%. White solid. M.p. 214–216 °C (lit. [12] 214–216 °C.). 1H-NMR (250 MHz, DMSO-d6): δ = 3.93 (brs, 1 H), 7.75–8.40 (m, 4 H) ppm. 13C-NMR (62.9 MHz, DMSO-d6): δ = 117.9, 125.7, 130.2, 130.7, 131.4, 134.4, 136.7, 154.8 ppm. IR (KBr): ν = 3113, 2981, 2780, 2442, 2237, 1476, 870, 780 cm−1.
7 4-(1H-Tetrazol-5-yl) benzonitrile (3d)
Yield: 95%. White solid. M.p. 258–260 °C (lit. [12] 258–260 °C). 1H-NMR (250 MHz, DMSO-d6): δ = 4.20 (brs, 1 H), 7.93 (d, J = 8.50 Hz, 2 H), 8.16 (d, J = 8.50 Hz, 2 H) ppm. 13C-NMR (62.9 MHz, DMSO-d6): δ = 113.3, 118.1, 127.5, 128.7, 133.27, 155.2 ppm. IR (KBr): ν = 3100, 2848, 2750, 2250, 1480, 781 cm−1.
8 4-(1H-Tetrazole-5-yl) pyridine (3e)
Yield: 95%. White solid. M.p. 254–257 °C (lit. [12] 254–258 °C). 1H-NMR (250 MHz, DMSO-d6): δ = 7.27 (brs, 1 H), 7.98 (s, 2 H), 8.78 (s, 2 H) ppm. 13C-NMR (62.9 MHz, DMSO-d6): δ = 120.9, 133.2, 150.1, 155.3 ppm. IR (KBr): ν = 3414, 2814, 2725, 1626, 1597, 1512, 1384, 1350, 1039, 833 cm−1.
9 2-(1H-Tetrazol-5-yl)pyridine (3f)
Yield: 90%. White solid. M.p. 208–210 °C (lit. [11] 210–213 °C). 1H-NMR (250 MHz, DMSO-d6): δ = 3.93 (brs, 1 H), 7.64–7.58 (m, 1 H), 8.03–8.09 (m, 1 H), 8.19 (d, J = 8.76 Hz, 1H), 8.74 (d, J = 4.86 Hz, 1 H) ppm. 13C-NMR (62.9 MHz, DMSO-d6): δ = 122.7, 126.1, 138.5, 143.3, 149.7, 154.7 ppm. IR (KBr): ν = 3417, 2818, 2724, 1623, 1599, 1512, 1384, 1350, 1039, 833 cm−1.
10 5-(4-Trifluoromethyl)phenyl)-1H-tetrazole (3 g)
Yield: 95%. White solid. M.p. 218–219 °C (lit. [14] 220–222 °C). 1H-NMR (250 MHz, DMSO-d6): δ = 4.71 (brs, 1H), 7.61 (d, J = 8.00 Hz, 2H), 7.97 (d, J = 8.00 Hz, 2 H) ppm.; 13C-NMR (62.9 MHz, DMSO-d6): δ = 125.7, 127.5, 127.6, “129.8; 125.4; 121.1; 116.8” (–CF3, J = 272.35 Hz), “131.6; 131.1; 130.6; 130.1” (–C–CF3, J = 32.07 Hz), 155.0 ppm.
11 5-(3-Bromophenyl)-1H-tetrazole (3 h)
Yield: 95%. White solid. M.p. 151–153 °C (lit. [14] 155–156 °C). 1H-NMR (250 MHz, DMSO-d6): δ = 4.27 (brs, 1H), 7.41 (d, J = 9.66 Hz, 1 H), 7.57 (d, J = 9.6 Hz, 1 H) 7.98 (s, 1 H), 7.84 (d, J = 7.50 Hz, 1 H) ppm. 13C-NMR (62.9 MHz, DMSO-d6): δ = 122.1, 125.8, 128.9, 131.0, 133.5, 140.2, 154.4, 162.8 ppm.
12 5-(2-Chlorophenyl)-1H-tetrazole (3i)
Yield: 90%. Yellow solid. M.p. 180–181 °C (lit. [15] 180–181 °C). 1H-NMR (250 Hz, DMSO-d6): δ = 4.21 (brs, 1 H), 7.58 (s, 1H), 7.65 (s, 1 H), 7.72 (s, 1 H), 7.83 (s, 1 H) ppm. 13C-NMR (125 Hz, DMSO-d6): δ = 124.6, 128.3, 130.9, 132.2, 132.4, 133.1 ppm. IR (KBr): ν = 1470, 1563, 1602, 2923 cm−1.
13 5-(3-Chlorophenyl)-1H-tetrazole (3j)
Yield: 95%. White solid. M.p. 137–139 °C (lit. [14] 138–139 °C). 1H-NMR (250 MHz, DMSO-d6): δ = 4.80 (brs, 1 H), 7.59 (s, 2 H), 7.95–8.00 (m, 2 H), ppm. 13C-NMR (125 Hz, DMSO-d6): δ = 125.5, 126.4, 129.3, 130.8, 131.3, 133.9, 154.7 ppm. IR (KBr): ν = 3068, 2968, 2430, 1558, 1473, 891 cm−1.
14 5-(4-Chlorophenyl)-1H-tetrazole (3k)
Yield: 92%. White solid. M.p. 262–263 °C (lit. [11] 261–263 °C) 1H-NMR (250 MHz; DMSO-d6): δ 8.04 (d, 2H, J = 8.52 Hz, Ar–H), 7.62 (d, 2H, J = 8.52 Hz, Ar–H), 4.15 (brs, 1H,–NH); 13C-NMR (62.9 MHz, DMSO-d6): δ 154.8, 135.8, 129.4, 128.6, 123.1; IR (KBr): ν = 3419, 2927, 2816, 2723, 1602, 1457, 1437, 1383, 1350, 1161, 1095, 1055, 875, 765 cm−1.
15 5-(4-Tolyl)-1H-tetrazole (3l)
Yield: 85%. White solid. M.p. 248–250 °C (lit. [8a] 248–249 °C). 1H-NMR (250 MHz, DMSO-d6): δ = 2.36 (s, 3 H), 3.61 (brs, 1 H), 7.38 (d, J = 8.25 Hz, 2 H), 7.90 (d, J = 8.25 Hz, 2 H) ppm. 13C-NMR (62.9 MHz, DMSO-d6): δ = 20.95, 121.12, 126.82, 129.90, 141.23, 155.01 ppm. IR (KBr): ν = 3062, 2983–2400, 2972, 1590, 1492, 823 cm−1.
16 5-(2-Tolyl)-1H-tetrazole (3 m)
Yield: 60%. White solid. M.p. 249–251 °C (lit. [8a] 248–249 °C). 1H-NMR (250 MHz, DMSO-d6): δ = 7.67 (d, J = 7.43 Hz, 1 H), 7.33–7.46 (m, 3 H), 4.65 (brs, 1 H) 2.45 (s, 3 H) ppm. 13C-NMR (62.9 MHz, DMSO-d6): δ = 20.4, 123.8, 126.2, 129.3, 130.6, 131.2, 137.0, 155.2 ppm.
17 5-(4-Methoxyphenyl)-1H-tetrazole (3n)
Yield: 60%. White solid. M.p. 230–231 °C (lit. [10a] 231–233 °C). 1H-NMR (250 MHz, DMSO-d6): δ = 7.97 (d, J = 10.28 Hz, 2 H), 7.14 (d, J = 10.27 Hz, 2 H), 3.80 (s, 3 H), 3.91 (brs, 1 H) ppm. 13C-NMR (62.9 MHz, DMSO-d6): δ = 55.3, 114.7, 116.1, 128.5, 154.6, 161.3 ppm. IR (KBr): ν = 3078, 2983, 1612, 1500, 1267, 1182, 835 cm−1.