1 Introduction
Wheat is a major cereal crop grown in the EU, with over 2 million hectares, generating approximately 7.5 million tonnes of straw annually in the UK [1]. Wheat straw has been demonstrated as a low value, high volume agricultural by-product idea for exploitation in a biorefinery [2,3]. The first stage of an integrated biorefinery is the extraction of valuable secondary metabolites such as lipids [3].
Lipids are traditionally extracted with volatile organic solvents. These solvents are unselective, toxic and environmentally problematic. Hexane has been recognised as a hazardous air pollutant by the US EPA and the EPA Toxic Release Inventory (TRI) indicated that more than 20,000 tonnes of hexane are released to atmosphere from extraction [4,5]. Hexane is also reported to be neurotoxic and greatly affecting the central nervous system [6,7]. Supercritical CO2 can be used as an environmentally friendly alternative solvent for the extraction of these valuable waxes [8].
Previous work on wheat straw demonstrated that a 1% yield of waxy cuticle lipids can be selectively extracted using hexane [9]. The polarity of scCO2 is similar to that of hexane and therefore scCO2 can be utilised as a suitable replacement for traditional organic solvents in extractions [8]. Due to its high diffusivity and low viscosity, scCO2 is an ideal solvent for extractions.
In addition, scCO2 extraction leaves no solvent residues, due to its low surface tension and ease of removal. Use of scCO2 can be considered energy intensive and costly but the environmental benefits and the tuneable properties can be a distinct advantage in natural products extraction.
Straw waxes have many potential applications such as flavourings, cosmetics, food supplements, coatings etc [10–13]. Many of these applications are strictly regulated, for example legislation is already in place restricting extraction solvent usage and residues in cosmetics, flavour and fragrances [14–19].
Herein, the extraction of valuable long chain aliphatic molecules was achieved from wheat straw with scCO2 and components were identified as β-diketones, wax esters, fatty acids, alkanes, fatty alcohols, aldehydes, sterols and sterones. Modelling of scCO2 extractions is important for the optimisation of processes and to achieve a commercial success at scale up. It is demonstrated that the Chrastil equation, which was established using empirical models can be used to effectively predict the crude wax yield and important groups of compounds such as wax esters in straw wax extraction [20].
2 Experimental
2.1 Supercritical carbon dioxide and conventional solvent extraction
Wheat straw (Viscount 09) (Triticum aestivum) was provided by Velcourt Group Plc. and milled using a Glen Creston Ltd. SM1 hammer mill to pass through a 2.5 mm screen.
For supercritical carbon dioxide extraction, about 130 g of air-dried milled wheat straw was extracted using a Thar SFE 500 system (Thar technologies). The straw can be loaded into the extractor and the CO2 supplied from a gas cylinder, which is liquefied through a cooling unit to avoid cavitation. The liquefied CO2 was then sent to the pre-heater and converted into a supercritical fluid prior to entering the extractor. Temperature (305–373 K), pressure (7.5–40 MPa) and flow rate of 0.04 kg.min−1 were selected according to the experiment. The total extraction time was 4 hours. An hour interval tapping was taken to collect different extracts through the course of the extraction. The extracts were all collected at 313 K and at atmospheric pressure in the separator. The wax extracts were collected by washing with the minimal amount of dichloromethane in the separator. The dichloromethane was evaporated off and the extracts were dried in vacuo until constant weight was achieved and the percentage crude yield was calculated.
For conventional solvent extraction, about 13 g of the air-dried milled wheat straw was extracted with 300 mL of selected organic solvent in a standard Soxhlet extraction apparatus for 5 hours. The recovered extracts were immediately filtered using fluted filter paper to remove any straw residues and concentrated to dryness in vacuo. To eliminate moisture and traces of residual solvent, the extracts were air-dried at room temperature. The extracts were weighed periodically until constant weight was achieved.
2.2 Gas Chromatography (GC) analysis
Approximately 25 mg of the crude extracts were dissolved in 1 cm3 of dichloromethane and analysed using an Agilent Technologies 6890N gas chromatograph. Exactly 0.5 μL of the sample was injected using the Agilent Technologies 7683B autosampler at 10:1 split mode. The system was fitted with a capillary column (ZB-5HT, 30 m × 0.25 mm I. D. X 0.25 μm film thickness, Phenomenex). The oven was temperature-programmed from 333 K (1 min hold) to 633 K at 8 °C·min−1 (10 min hold). The injector and the flame ionisation detector (FID) were both set at 573 K. Helium was used as the carrier gas and the flow rate was maintained at 2.2 cm3·min−1 in constant flow mode.
Fatty acid methyl esters (FAME) analysis was carried out by dissolving about 90 mg of crude extracts in 3 mL of hexane (containing approximately 2 mg·mL−1 internal standard tetradecane) and followed by the addition of 150 μL of 1 N methanolic sodium hydroxide. The solution was stirred vigorously for 20 minutes and left at room temperature until the organic and aqueous layers were separated. The organic layer was analysed using GC method as above and FAME were quantified using GCMS and fatty acid as standard.
Quantification of the wax compounds were carried out using a series of authentic standards. The standards were used as a representative of each wax group (e.g.: stearic acid was used for all fatty acids quantification). The standards used were stigmasterol for sterols, hentriacontane for alkanes, octacosanol for fatty alcohols, stearic acids for fatty acids and stearyl hexadecanoate for wax esters. As there is no authentic standard available for β-diketones, the Effective Carbon Number (ECN) method was used to quantify this group of compounds [21]. Tetradecane at a concentration of about 2 cm3·mg−1 was used as an internal standard for the samples and standards. The quantification analysis were carried out at least two times and the average was calculated.
Elmer Clarus 500 GC-MS with an autosampler, which was coupled with a Perkin Elmer Clarus 560S mass spectrometer. The mass spectrometer was operated in the electron impact (EI) mode at 70 eV, a source temperature of 573 K, quadrupole at 573 K, scanned over the mass range of 30–1200 amu.
The wax compounds were identified using GC-MS and confirmed using Kovats Index (KI) [22]. An ASTM® D5442 C12–C60 qualitative retention time mix (containing docosane, dodecane, dotriacontane, eicosane, hexacontane, hexacosane, hexadecane, hexatriacontane, octacosane, octadecane, pentacontane, tetracontane, tetracosane, tetradecane, tetratetracontane and triacontane) was used as an alkane standard mix to calculate the KI. The calculated KI was compared with literature KI for clarification.
3 Results and discussion
The crude yields of scCO2 extracted wheat straw waxes ranged from 0.1 to 1.8% depending on the extraction conditions indicating changes in chemical compositions under different solvent properties. A 2 × 2 factorial experimental design was adopted for the optimisation of supercritical extraction (Fig. 1). The temperature range for the study was selected to be between 305–373 K and the selected pressure range was between 7.5–40 MPa.
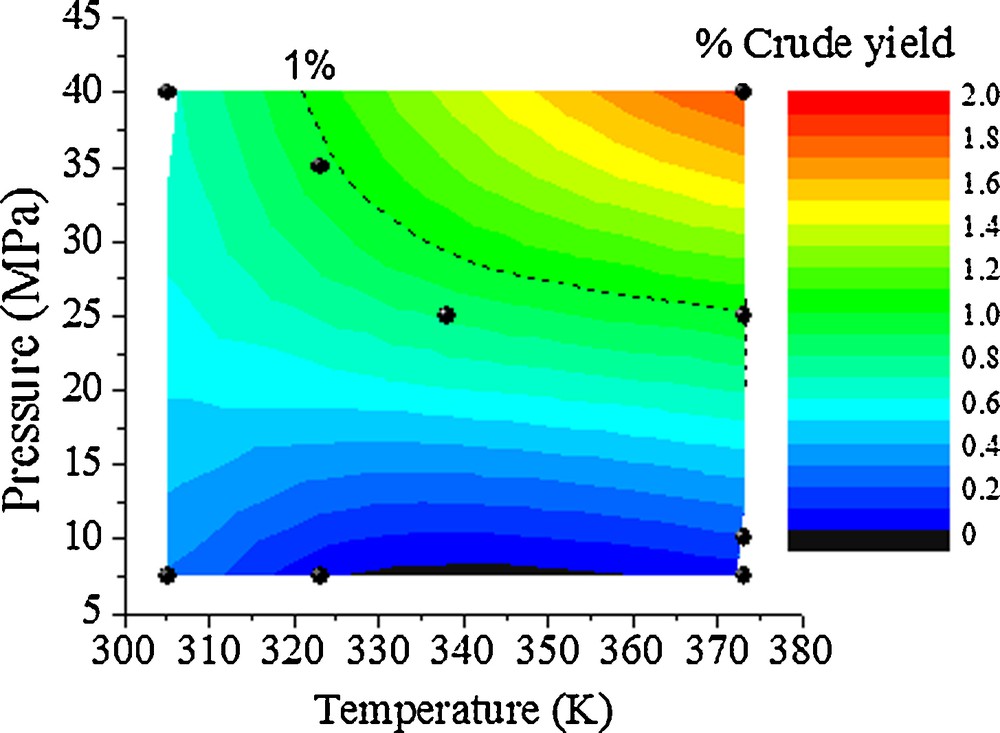
Percentage crude yields of wheat straw waxes from scCO2 extraction (red represents high yield of 2% and black represents no yield 0%).
The percentage crude yields of the different conditions vary as there is a compositional change during the course of extraction. The various extraction conditions were based on a single extraction time at 4 hours to give a fair and accurate comparison. The maximum percentage crude yields can be achieved at 4 hours extraction and an example of the changes with percentage crude yields over extraction times can be demonstrated in Fig. 2.

An example of the changes in percentage total accumulated crude yield against extraction times under supercritical CO2 extraction (338 K, 25 MPa).
The content of the wax in the straw is low and only comprised of 0.5–1.0% of the dry weight but with the huge volume of this agricultural by-product worldwide, a potential of 7.5–25 million tons of valuable waxes can be obtained each year [23]. To achieve 1% crude yield, the extraction data showed that a minimum temperature of 320 K and pressure of 25 MPa (density = 848.7 g.L−1) is required for the extraction. It is a combination of high pressure and temperature that enhanced the percentage crude yield and these results are in agreement with other scCO2 straw wax extractions [24]. At 305 K and a pressure of 40 MPa results in a CO2 density of 982.4 g.L−1 and only gives a low yield of 0.67% confirming that the density of CO2 is important, however there are other factors that dictate the solubility of wax constitutes in CO2. Both temperature and pressure dictate the density and dielectric constant of the CO2, which influence the solvating power, thereby increasing the solubility of solutes in the extracting fluid [25]. Cuticular wax is semi-crystalline and enough thermal energy is required in order to increase the solubility of wax components [26].
The extraction of wheat straw waxes by supercritical CO2 can be compared with traditional organic solvents as shown on Fig. 3. Extraction of CO2 is commonly being compared with hexane or heptane extraction and compositional comparisons between supercritical and heptane extracts can be observed in the supporting information (Table S1, Supplementary data). The yield for heptane is between the two supercritical CO2 extraction conditions indicating the versatility of carbon dioxide as a solvent, therefore careful optimisation of CO2 conditions must be carried out for all extractions. Optimisation of supercritical CO2 extraction, the favourable chemical compositions can be achieved and giving the desirable physical properties for the applications.

Comparison of percentage crude yields on organic solvents and supercritical carbon dioxide.
Wheat straw waxes are complex mixtures of compounds with a large range of molecular weight and structures so the solubility of these molecules would be different in various scCO2 conditions. To investigate the relationship between the composition of extract and scCO2 conditions, individual compounds were identified and quantified using GC and GCMS (Table 1). Seven main groups of molecules were found: fatty acids, alkanes, sterols, β-diketones, fatty alcohols, aldehydes and wax esters (Fig. 4). However, to fully understand the impact of each extraction parameter on the crude yields and individual groups of compounds, the Chrastil model was adapted further.
Identification and quantification of wheat straw wax of various scCO2 conditions.
Identification | Calculated KI | Literature KI | μg.g−1 of wheat straw | ||||||||
373 K/40 MPaa | 373 K/25 MPaa | 323 K/35 MPaa | 338 K/25 MPaa | 305 K/40 MPaa | 305 K/7.5 MPaa | 373 K/10 MPaa | 323 K/7.5 MPaa | 373 K/7.5 MPaa | |||
Tetradecanoic acid | 1750 | 1751 [54] | 78 ± 12 | 76 ± 11 | 47 ± 4 | 76 ± 12 | 63 ± 1 | 36 ± 5 | 48 ± 5 | 11 ± 5 | 4 ± 1 |
Hexadecanoic acid | 1951 | 1950 [55] | 476 ± 13 | 642 ± 34 | 398 ± 34 | 560 ± 29 | 361 ± 24 | 232 ± 3 | 267 ± 16 | 77 ± 14 | 96 ± 14 |
Octadecadienoic acid | 2126 | 2132 [56] | 142 ± 16 | 333 ± 23 | 77 ± 19 | 69 ± 12 | 53 ± 14 | 62 ± 5 | 79 ± 13 | 35 ± 4 | 9 ± 3 |
Octadecenoic acid | 2128 | 2137 [57] | 271 ± 23 | 574 ± 12 | 158 ± 12 | 191 ± 10 | 264 ± 3 | Tr | 121 ± 4 | 63 ± 7 | 18 ± 2 |
Octadecanoic acid | 2148 | 2168 [58] | 58 ± 9 | 129 ± 45 | 56 ± 3 | 76 ± 11 | 47 ± 5 | 31 ± 7 | 24 ± 8 | 14 ± 2 | 11 ± 3 |
Total fatty acids | – | – | 1026 ± 73 | 527 ± 125 | 736 ± 72 | 344 ± 74 | 787 ± 47 | 361 ± 20 | 539 ± 46 | 589 ± 32 | 139 ± 23 |
Heptacosane | 2685 | 2700 [22] | 109 ± 10 | 88 ± 10 | 74 ± 8 | 75 ± 11 | 55 ± 5 | 15 ± 1 | 29 ± 1 | 15 ± 5 | 7 ± 2 |
Nonacosane | 2884 | 2900 [22] | 280 ± 18 | 281 ± 24 | 218 ± 13 | 231 ± 14 | 190 ± 7 | 33 ± 3 | 51 ± 5 | 33 ± 14 | 10 ± 2 |
Hentriacontane | 3083 | 3100 [22] | 1214 ± 45b | 1176 ± 37b | 414 ± 45 | 883 ± 35b | 342 ± 15 | 22 ± 5b | 24 ± 2b | 22 ± 4 | 16 ± 3b |
Triatriacontane | 3282 | 3300 [22] | Tr | Tr | 74 ± 4 | 106 ± 5 | 60 ± 3 | Tr | Tr | Tr | Tr |
Total alkanes | – | – | 1603 ± 73 | 465 ± 71 | 779 ± 70 | 459 ± 65 | 647 ± 30 | 207 ± 9 | 104 ± 8 | 207 ± 23 | 33 ± 7 |
Campesterol | 3238 | 3193d | 153 ± 13 | 239 ± 11 | 102 ± 10 | 155 ± 6 | 83 ± 5 | 30 ± 7 | Tr | 2 ± 1 | Tr |
Stigmasterol | 3272 | 3249 [59] | 191 ± 16 | 229 ± 4 | 140 ± 11 | 106 ± 7 | 110 ± 15 | 36 ± 1 | Tr | 10 ± 3 | Tr |
β-sitosterol | 3334 | 3408 [60] | 401 ± 34 | 544 ± 30 | 327 ± 15 | 245 ± 16 | 247 ± 4 | 89 ± 5 | Tr | 29 ± 6 | 4 ± 1 |
Sterol 1c | 3400 | – | 72 ± 7 | 141 ± 8 | 83 ± 15 | 87 ± 10 | 83 ± 6 | 27 ± 6 | Tr | 29 ± 7 | Tr |
β-sitosterone | 3476 | – | 313 ± 8 | 304 ± 19 | 241 ± 12 | 246 ± 6 | 208 ± 13 | Tr | 4 ± 1 | 35 ± 4 | Tr |
Sterol 2c | 3636 | – | Tr | 202 ± 10 | 146 ± 34 | 130 ± 7 | 103 ± 8 | 29 ± 5 | 7 ± 1 | 35 ± 4 | Tr |
Total sterols | – | – | 1130 ± 78 | 1191 ± 82 | 1040 ± 97 | 968 ± 52 | 834 ± 51 | 274 ± 24 | 11 ± 2 | 37 ± 25 | 4 ± 1 |
14,16 hentriacontanedione | 3377 | 3375e | 1505 ± 56 | 1231 ± 56 | 1052 ± 56 | 1120 ± 56 | 1111 ± 19 | 994 ± 18 | 17 ± 4 | 309 ± 23 | 4 ± 1 |
16,18 triatriacontanedione | 3581 | – | 195 ± 16 | 135 ± 4 | 217 ± 16 | 125 ± 22 | 144 ± 14 | Tr | Tr | 31 ± 1 | Tr |
Total β-diketones | – | – | 1700 ± 72 | 1366 ± 60 | 1270 ± 72 | 1245 ± 78 | 1254 ± 33 | 994 ± 18 | 17 ± 4 | 339 ± 24 | 4 ± 1 |
Tetracosanyl hexadecanoate | 4137 | – | 113 ± 11 | 51 ± 4 | 126 ± 16 | Tr | 79 ± 5 | Tr | Tr | 3 ± 1 | 26 ± 5 |
Hexacosanyl hexadecanoate | 4335 | – | 274 ± 15 | 137 ± 8 | 233 ± 18 | 49 ± 10 | 194 ± 14 | 40 ± 3 | 10 ± 4 | 6 ± 2 | 17 ± 5 |
Octacosanyl hexadecanoate | 4513 | – | 1160 ± 45 | 526 ± 19 | 857 ± 39 | 123 ± 8 | 863 ± 6 | Tr | 9 ± 4 | 4 ± 2 | 13 ± 4 |
Octacosanyl octadecanoate | 4699 | – | 630 ± 25 | 314 ± 19 | 577 ± 27 | 507 ± 24 | 358 ± 35 | Tr | 9 ± 3 | 22 ± 4 | 29 ± 7 |
Octacosanyl eicosanoate | 4953 | – | 438 ± 33 | 225 ± 15 | 445 ± 26 | 231 ± 17 | 156 ± 3 | Tr | 6 ± 1 | 11 ± 1 | 52 ± 18 |
Octacosanyl docosanoate | f | – | 378 ± 31 | 229 ± 20 | 417 ± 24 | 158 ± 13 | 83 ± 5 | Tr | Tr | Tr | 24 ± 4 |
Octacosanyl tetracosanoate | f | – | 137 ± 13 | Tr | 131 ± 13 | 176 ± 18 | 53 ± 8 | Tr | Tr | Tr | Tr |
Octacosanyl hexacosanoate | f | – | 72 ± 11 | Tr | 56 ± 19 | 89 ± 5 | Tr | Tr | Tr | Tr | Tr |
Octacosanyl octacosanoate | f | – | Tr | Tr | 63 ± 19 | Tr | Tr | Tr | Tr | Tr | Tr |
Total wax esters | – | – | 3203 ± 184 | 430 ± 85 | 2906 ± 182 | 472 ± 95 | 1787 ± 76 | 40 ± 3 | 33 ± 12 | 46 ± 10 | 160 ± 43 |
Octacosanol | 3083 | 3118d | 1570 ± 78b | 1521 ± 56b | 733 ± 26 | 1142 ± 37b | 303 ± 24 | 185 ± 17b | 31 ± 5b | 22 ± 3 | 16b ± |
Total fatty alcohol | – | – | 1570 ± 78 | 1521 ± 56 | 733 ± 26 | 1142 ± 37 | 303 ± 24 | 185 ± 17 | 31 ± 5 | 22 ± 3 | 16 ± |
Octacosanal | 3024 | – | 108 ± 6 | 207 ± 33 | 168 ± 15 | 182 ± 17 | 86 ± 11 | 33 ± 4 | Tr | 16 ± 3 | 6 ± 1 |
Total aldehyde | – | – | 108 ± 6 | 207 ± 33 | 168 ± 15 | 182 ± 17 | 86 ± 11 | 33 ± 4 | Tr | 16 ± 3 | 6 ± 1 |
Total identified | – | – | 8873 ± 564 | 4293 ± 512 | 6987 ± 534 | 3670 ± 418 | 5475 ± 272 | 1908 ± 95 | 704 ± 77 | 1335 ± 130 | 565 ± 79 |
a CO2 flow rate of 0.04 kgmin−1 for 4 hours.
b Co-elution of hentriacontane and octacosanol showed total of both compound.
c Further work is needed for full identification.
d Literature KI: DB-1 GC column.
e Estimated non-polar retention index (n-alkane scale) from NIST library 2008.
f Hexacontane: no clear peak so no KI calculated Tr = Trace level with limit of quantification approximately < 0.1 mgmL−1.
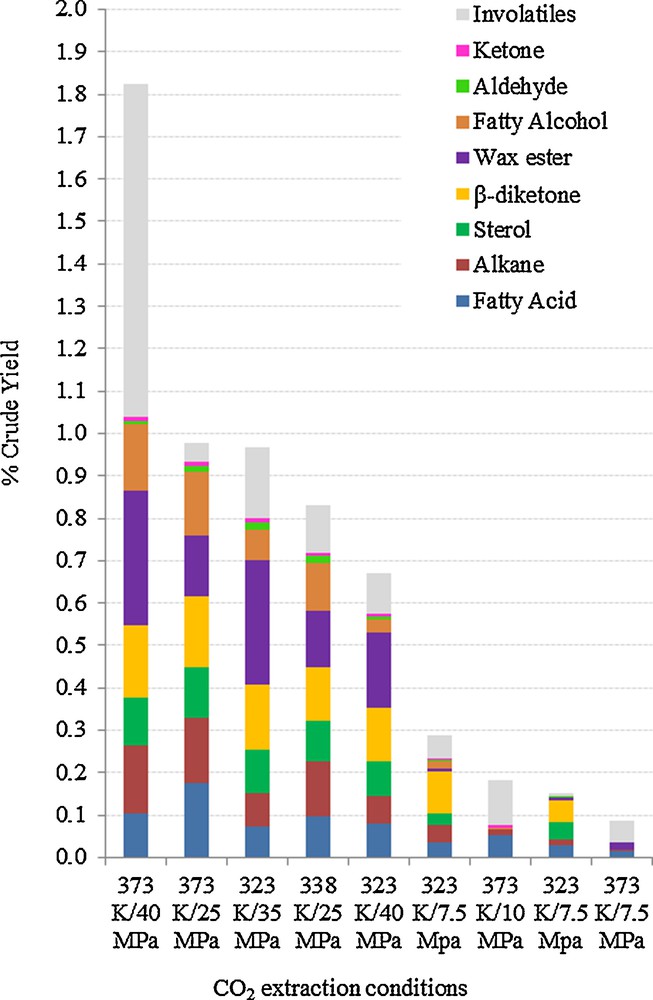
Complete breakdown of main groups in wheat straw waxes from scCO2 extractions.
As shown in Table 1, the aliphatic molecules are of long chain length ranging from C14 to C56. The smaller aliphatic molecules found were at low concentrations free fatty acids (C14–C18) with various degrees of unsaturation. These low levels of free acids arise from lipid biosynthesis and possibly from hydrolysis of triglycerides or wax esters as the plant enter senescence [27].
Odd-numbered straight chain alkanes from C27–C33 were predominantly found in wheat straw waxes and these are known to be the most abundant in many plants including wheat [28,29]. The odd-numbered chain derives from the decarboxylation of even-numbered fatty acids precursors [27]. Crude waxes contained 3–16% of these odd alkanes depending on the scCO2 extraction conditions, with hentriacontane (C31) being the most abundant single alkane. Even-numbered alkanes do not tend to be found in plants due to the biosynthesis routes and if identified, it would be at an extremely low level in comparison [30]. At medium pressure (338K/373K and 25 MPa), the elevated temperature does not appear to have any beneficial effect on the yield however, when the temperature was increased from 305 to 373 K at 40 MPa, the level of alkanes almost tripled. This highlighted the importance of increased pressure on alkane extraction.
Sterols such as stigmasterol, campesterol and β-sitosterol which comprised of 0.5–27% of the extracts were identified. These are common cereal phytosterols that have been identified in wheat and other cereal crops such as barley and oat in previous studies [31,32]. Phytosterols are important class of compounds and have nutritional benefits and used as food supplements in for reducing blood cholesterol level [33,34]. Free β-sitosterol was the major sterol in the lipid fractions under all extraction conditions, which is in agreement with other research data [32]. This is the case because β-sitosterol is the first sterol synthesised in the sterol biosynthetic pathway [35]. However, cholesterol has also been reported as a major component in these cereal crops but this was not found in any of the CO2 extracts [32]. The data from Table 1 showed increased pressure from 25 to 40 MPa does not have a significant effect on sterol yield which indicated that increasing the CO2 density to above 600 g.L−1 would not benefit sterol extraction. Previous studies indicated that sterols can also exist as bound forms such as steryl esters within wheat straw however, no steryl esters were found [36].
Up to 30% of long chain aliphatic wax esters are extracted at high temperature (373 K), data showed that a higher proportion of wax esters have been extracted at higher temperature which could be due to the high melting points. The melting point (Tm) of wax esters are much higher than other compounds found in the extracts due to the extended chain length. Saturated wax ester tetracosanyl tetracosanate (C24:0–C24:0) has a Tm of > 348 K and an increase of 1–2 °C per extra carbon on the molecules [37]. The main factor influencing the Tm was shown to be the chain length of the wax esters [37]. The smallest wax ester identified was tetracosanyl hexacosanoate (C24:0–C26:0) and this means that the fraction is not molten until the extraction temperature is at least 348 K. At high (373 K) temperature extractions, it was believed that the wax esters are melting on the surface of the straw, which can enhance the solubility of these long chain wax esters (> C40) in scCO2. Structures of wax esters in wheat are derived from esterification of saturated primary even chain alcohol and fatty acids and the chain lengths ranged from C40–C56 [27]. In this case, the most abundant is octacosanyl hexadecanoate (C28:0–C26:0) that it is synthesised from the most abundant fatty alcohol (octacosanol) and free fatty acid (palmitic acid). In addition, trace levels of odd chain aliphatic esters were also found in the wheat straw waxes. It was reported that odd chain wax esters found in barley had carbon chains in the range C31–C37 where the alcohol moieties are secondary alcohols of C11–C17 and wheat straw waxes may possess the same homologous series [38]. Wax esters are an important class of compounds as they have a large number of applications from lubricants to cosmetics [39,40].
Fatty alcohol (octacosanol) was found at high concentrations, which is in agreement with earlier studies [28]. Policosanols are a class of higher primary aliphatic long chain fatty alcohol, which are found in plant waxes, which can be used to lower cholesterol level [41]. It was shown that octacosanol is the compound that determined the shape of the wax platelets in wheat straw [42]. Aldehydes have also been previously identified and in this particular variety of wheat, only the aldehyde octacosanal was identified which is in agreement with the biosynthetic route [27]. Aldehydes are the intermediate between alcohols and fatty acids precursors and as octacosanol is the only fatty alcohol identified, this suggests that the octacosanal maybe possibly present due to partial oxidation of the alcohol [27].
Another interesting class of molecule in the extracts are the β-diketones (0.5–35%), with 14,16 hentriacontanedione (up to 31%) being the most abundant molecule in the extracts. It commonly have the 1,3-dicarbonyl group in positions, 12 and 14 or 14 and 16 or 16 and 18 of C29, C31 and C33 alkyl chains [43]. These odd-numbered β-diketones are synthesised during the elongation process in making the fatty acids precursors [44]. The β-keto group is protected by metal ions such as copper in various enzymes, which can have important applications as metal chelators [44,45]. These diketones can also be used for making super-hydrophobic coatings [46]. The β-diketones only exist as odd-numbered as a similar decarboxylation step to alkanes also occur when the molecules are formed [44]. Hydroxy-β-diketones are also commonly found in wheat straw waxes but were not found in this variety of wheat [47]. From Table 1, it is important to note that about 1000 μg.g−1 of 14,16 hentriacontanedione in straw can be extracted at critical temperature and pressure and the level remained similar at increased temperature of up to 373 K and increased pressure of 35 MPa. This indicated that the solubility of β-diketone in scCO2 is very high. The data showed that this valuable molecule can potentially be fractionated off at the critical point of CO2 (at about 35% purity), then increasing the temperature and pressure for the extraction of the other wax components.
The extracts consist of common lipid groups of mainly aliphatic long carbon chain with various different functional groups and one cyclic group of compounds. Minimal branched components were found in the extracts but have been reported in other plant species, however at much lower proportions [48]. The unidentified compounds were suspected to be involatile to analysis using GC. FAME analysis was carried out on the 373K and 40 MPa extract and found between 2–5.5% of glycerides. The hydrolysis and methylation only occurred to the glycerides as the wax esters were still remained in the extracts. Methyl hexadecanoate, methyl octadecanoate, methyl octadecenoate and methyl octadecadienoate were identified but the original structure of the glycerides was not determined. Previous research showed that glycerides usually exist as diglycerides and triglycerides in wheat straw as opposed to monoglycerides. Tripalmitin, triolein and dipalmitoyl-oleoylglycerol were identified to be the main glycerides presented on the surface of wheat straw [36,49]. Other groups of compounds that have previously been identified included phenolic acids, resin acids and steryl esters [49].
In order to selectively extract and optimise the extraction of valuable components such as wax esters, extraction models to calculate supercritical solubility behaviour must be established. In the past, many density-based supercritical models have been explored (Chrastil, Del Valle and Aguilera, Adachi and Lu, Méndez-Santiago and Teja, Bartle) [20,50–52]. The Chrastil model (Eqn. [1]) is one of the first models proposed which directly relates the solubility of solute and the density of CO2 [20]. Many of the others are derived from the Chrasil model but modified to improve their suitability for different circumstances. Del Valle and Aguilera highlighted the limitations of the model such as high solute solubility and limited temperature range in their study of extraction of vegetable oil [50].
Eqn. [1] shows the Chrastil equation:
(1) |
(2) |
Fig. 5 showed a linear relationship between experimental ln(c) and predicted ln(c) between (305 K and 7.5 MPa) to (373 K and 40 MPa) which indicated the extraction can be modelled using Eqn. [1] derived by Chrastil.
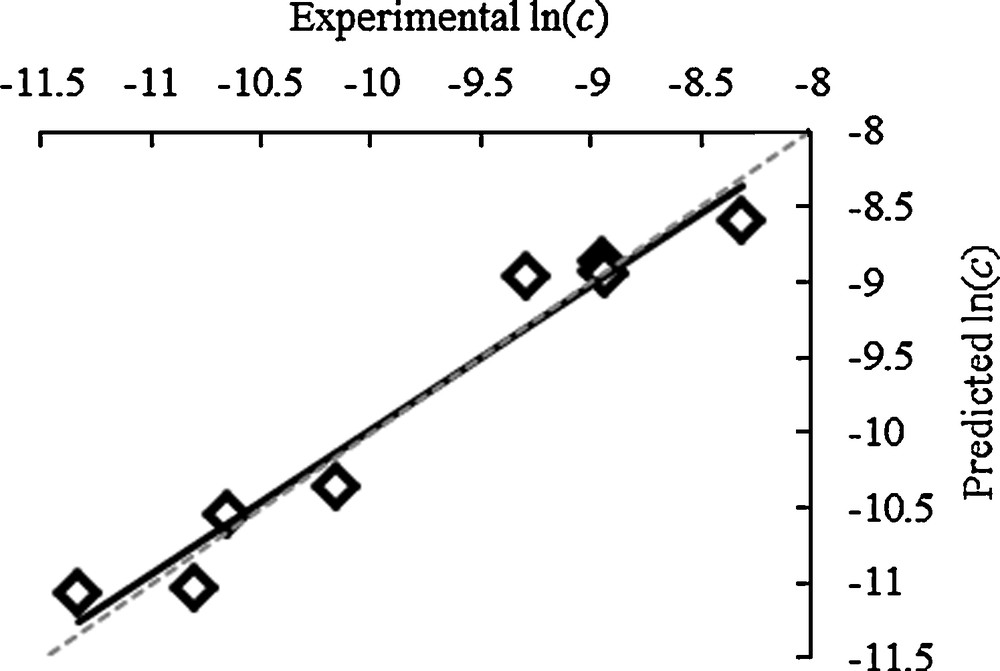
Experimental ln(c) against predicted ln(c) for % crude yield.
From Fig. 5, it can be concluded that high temperature and pressure would favour the extraction of waxes from wheat straw. However, as part of the straw biorefinery, it is crucial that the straw remained in a usable condition for exploitation such as co-firing for energy or microwave pyrolysis for bio-oil [3]. Most large commercial plants built in the 1980s and 1990s will not operate above 35 MPa due to equipment design so the maximum pressure would be limited. However, later plants have been designed to operate at up to 55 MPa so the extraction times can be significantly reduced. Operating at a high-pressure means scCO2 extraction is an energy intensive and costly process, so a full economical assessment must be carried out to calculate the extra costs against extra wax yield. The limitation of the model (temperature 305–373K and pressure 7.5–40 MPa) might result in that the optimum wax extraction conditions may not have been reached. The total wax content present on the straw surfaces is unknown at this stage so it is difficult to conclude that the straw has been completely de-waxed and extraction has gone to completion. Further extending the models extraction limits would be of interest from an academic point but, would add limited commercial value due to the added cost of large-scale extractions at higher pressures.
For the first time, the Chrastil equation had been applied to just a single groups of molecules within a complex mixture. Wax esters, the highest value components of the wax with many high value applications such as cosmetics, were selected for modelling within the extracts [11]. The same multiple linear regression was applied with the mass of wax esters extracted in ln(c) with ln(d) to calculate new k, a and b for the development of new model that is specifically to wax esters only. A new equation for wax esters have been developed as shown as Eqn. [3] with r2 = 0.90.
(3) |
Fig. 6 showed a similar straight line to Fig. 5 for the prediction of percentage yield for wax esters. This was especially useful in terms of finding the optimum conditions for the extraction of high wax esters fraction.
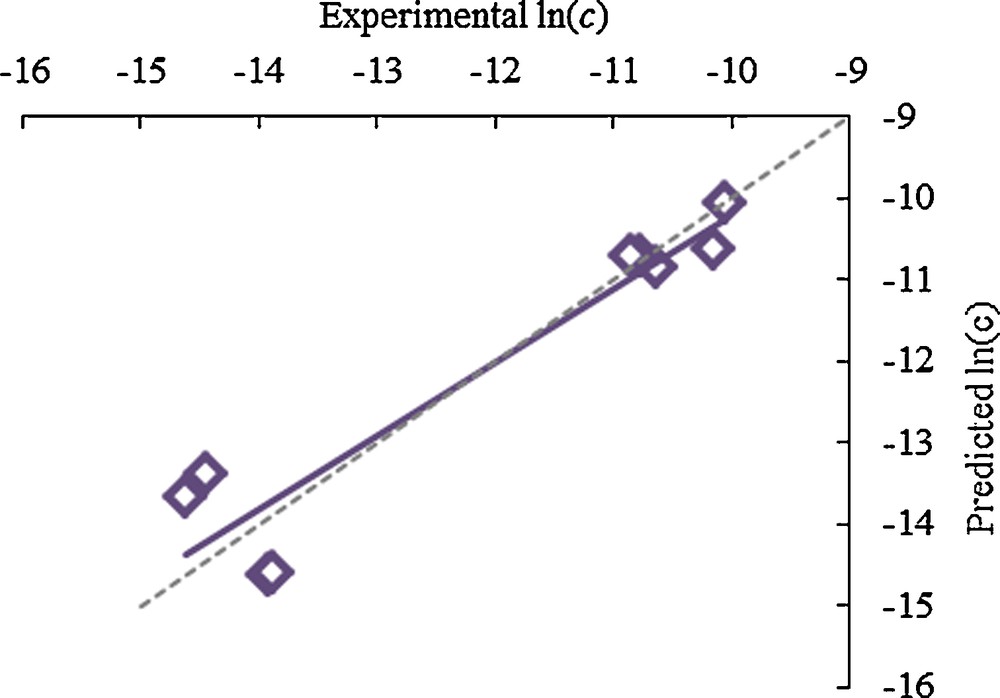
Experimental ln(c) against predicted ln(c) for % wax esters in scCO2 extract.
As shown in Fig. 6, there were two distinct clusters of points in which low ln(c) indicated high wax esters yield and high ln(c) showed low wax esters yield. Interestingly, the trend cannot be explained by density of CO2 alone and there are two opposing factors that are important in wax esters extraction. Firstly, the increase of extraction temperature leads to high Tm long chain wax esters melting on straw surfaces, thus resulting in an increase the solubility of wax esters in CO2. However, the second and opposing factor is that any increase of temperature results in the decrease in CO2 density, thus leading to reduced solvating power. Lucas et al. investigated the influence of temperature on the yields of extracted lipids [53]. This work suggested that increased volatility and solubility of lipid compounds gained by using elevated temperatures was more important than the reduction in carbon dioxide density. This effect is in agreement with those observed in the supercritical extraction of wheat straw. Importantly, at lower pressures, an increase of temperature does not result in a significant rise in wax yield, leading to a cluster of extracts with lower yields. The use of an elevated temperature must be accompanied with increased pressure for the enhancement wax ester extraction from wheat straw. Such increases in pressure and temperature lead to a second cluster of extractions with higher wax esters yields, at pressures above 25 MPa.
4 Conclusions
The key compounds within the wheat straw wax (fatty acids, sterols, fatty alcohols, wax esters, β-diketones and alkanes) have been identified and quantified. This valuable sustainable wax can be selectively extracted using scCO2 by the manipulation of pressure and temperature. This demonstrates that scCO2 is a selective alternative to traditional organic solvent for extractions of wheat straw waxes. It is the first time that the extraction of cereal straw wax has been modelled. The data generated was shown to obey the Chrastil model. Using the newly developed Chrastil model, the percentage crude yield of wheat straw waxes and percentage of wax esters within the extracts can be predicted, enabling maximum extraction and improving efficiency. By understanding the solvent selectivity of wax esters, downstream purification and fractionation steps can be reduced which would make the whole process more economical and environmentally friendly for any applications. Straw waxes have many potential applications, which are strictly regulated and restrict solvent usage and residues in cosmetics, flavour and fragrances. As scCO2 is highly selective, tuneable and leaves no solvent residues it is an ideal solvent for the extraction of waxes for such applications.
Acknowledgements
The authors would like to acknowledge Croda Enterprises Ltd. and BBSRC for the financial support for this project, Velcourt Group Plc. for the supply of wheat straw.