1 Introduction
The occurrence of human and veterinary pharmaceuticals in different water bodies worldwide has become recently a serious environmental problem [1]. These compounds cannot be treated by wastewater treatment plants, resulting in their persistence and accumulation to measurable levels in aquatic ecosystems [2,3]. Over the last decade, a large variety of physical, chemical and biological technologies were used for wastewater treatment [4,5], but did not appear to be enough effective when dealing with wastes containing antibiotics, owing to the important recalcitrance of these compounds. Therefore, the development of efficient treatment methods to mineralize antibiotics before their disposal in the environment is needed.
Advanced oxidation processes (AOPs) are potentially powerful methods, based on the generation of hydroxyl radicals (•OH), which are very reactive and non-selective oxidizing agents, leading to the degradation of organic pollutants by hydrogen atom abstraction reaction, electron transfer, or electrophilic addition to π systems [6–10]. These processes involve chemical, photochemical or electrochemical techniques such as Fenton, UV/or H2O2/ozonation, photo-Fenton, heterogeneous photocatalysis and electro-Fenton [9–13].
The Electro-Fenton process is an electrochemical advanced oxidation process based on the continuous generation of H2O2 in an acidic medium through the electrochemical reduction of O2 at the cathode (Equation 1). The generated H2O2 species reacts with the added Fe2+ ions to produce hydroxyl radicals (•OH) and Fe3+ ions via Fenton's reaction (Equation 2), which is favored by the catalytic action of the Fe3+/Fe2+ system, mainly from the regeneration of Fe2+ by the cathodic reduction of Fe3+ (Equation 3) [6,9,11,14]. Moreover, the method and the involved reactor are easy to handle and to use.
(1) |
(2) |
(3) |
However, the electro-Fenton oxidation for complete mineralization requires generally a long time and can be then expensive, because by-products generated during electrolysis may be more resistant to their degradation and compete with the target degradation compound. In this case, one alternative is to apply this electrochemical method as a pretreatment step, in order to convert the initial persistent compound into more biodegradable intermediates, which would then be treated in a biological degradation process with the aim of reducing costs [15].
Sulfamethazine (STM) is an antibiotic that belongs to the pharmaceutically important group of heterocyclic sulfonamides. It is widely used in medicine and veterinary practice as an antibacterial drug in pharmaceutical preparations [16]. Degradation of sulfamethazine has been examined through various methods. These studies mainly deal with advanced oxidation processes, such as photo-Fenton [17] and photocatalysis with TiO2 and ZnO as catalysts [16]. Among AOP, the relevance of electrochemical advanced oxidation processes (EAOP) for the pretreatment of synthetic sulmathezine solutions was also recently shown [18].
The purpose of this study was therefore to complete these previous works and hence to confirm the relevance of such a combined process for the mineralization of a synthetic solution of a veterinary antibiotic, sulfamethazine, and its implementation to an industrial pharmaceutical effluent containing this antibiotic.
2 Materials and methods
2.1 Chemicals
Sufamethazine (99%) and acetonitrile (purity 99.9%) (HPLC grade) were purchased from Sigma Aldrich (Saint-Quentin-Fallavier, France). FeSO4·7H2O (purity 99%) and Na2SO4 (purity 99%) used as a catalyst source and inert supporting electrolyte respectively, were provided from Acros Organics (Thermo Fisher Scientific, Illkirch, France). All solutions were prepared in ultra-pure water and all the other chemicals used for analysis were purchased from Acros Organics and Sigma Aldrich.
2.2 Characterization of the pharmaceutical effluent
The effluent used in this study was supplied by a pharmaceutical industry located in northern Tunisia, collected in a closed container and stored in the dark at 4 °C. Before treatment, the effluent was diluted to obtain an initial SMT concentration of 0.2 mM, in order to conduct the treatment in the same conditions as those of the synthetic solution. The main characteristics of this pharmaceutical effluent were collected in Table 1.
Main characteristics of pharmaceutical effluent.
[SMT] (g·L−1) | Conductivity (mS·cm−1) | COD (mg·L−1) | TOC (mg·L−1) | |
Pharmaceutical effluent | 1.2 | 1.2 | 11320 | 3774 |
2.3 Analytical determinations
2.3.1 High-performance liquid chromatography (HPLC)
The evolution of sulfamethazine concentrations was monitored by HPLC using a Waters 996 system equipped with Waters 996 PDA (Photodiode Array Detector) and Waters 600LCD Pump. The separation was achieved on a Waters C18, (5 μm; 4.6 × 250 mm) reversed-phase column. The effluent consisted of a mixture of acetonitrile/water (35/65 v/v with a synthetic solution and 20/80 v/v with a pharmaceutical effluent) delivered at a flow rate of 1 mL·min−1. The detection of sulfamethazine was carried out at 268 nm.
2.3.2 Chemical oxygen demand (COD) measurements
Chemical oxygen demand (COD) was measured by means of Nanocolor® tests CSB 160 and 1500 from Macherey-Nagel (Düren, Germany). The amount of oxygen required for the oxidation of the organic and mineral matter at 148 °C for 2 h was quantified after oxidation with K2Cr2O7 at acidic pH [19].
2.3.3 Total organic carbon (TOC) measurements
The solutions were filtered on Sartorius Stedim Minisart 0.40-μm GF prefilters (Göttingen, Germany). TOC was measured by means of a TOC-VCPH/CPN Total Organic Analyzer Shimadzu. Organic carbon compounds were combusted and converted into CO2, which was detected and measured by a non-dispersive infrared detector (NDIR). Reproducible TOC values were always obtained using the standard non-purgeable organic carbon (NPOC) method. For each sample, each measurement was triplicated [15].
2.3.4 Activated sludge preparation
The activated sludge used in this study was collected from a local wastewater treatment plant (Rennes Beaurade, Bretagne, France). Before use and to avoid any residual carbon or mineral nutrient, it was treated as previously detailed [20].
2.3.5 Biological oxygen demand (BOD5) measurements
BOD5 measurements were carried out in Oxitop IS6 (WTW, Alès, France). Activated sludge provided by a local wastewater treatment plant (Rennes Beaurade, Bretagne, France) was used to inoculate duplicate flasks and the initial microbial concentration was set to 0.05 g·L−1. More details regarding the experimental procedure can be found in a previous paper [18].
2.4 Experimental procedure
2.4.1 Electro-Fenton process
The degradation of the organic matter by the electro-Fenton process was carried out in a 1-L undivided cylindrical glass cell equipped with two electrodes, a carbon felt piece placed on the inner wall of the cell (Mersen RVG 4000, Paris La Défense, France) and was used as the cathode; a cylindrical platinum electrode located in the center of the electrochemical reactor (in order to have a good potential distribution) was used as the anode. Prior to electrolysis, compressed air was bubbled for 10 min through the solution to saturate the aqueous solution [20].
The pH of the solutions was adjusted to 3 by sulfuric acid (H2SO4). A catalytic quantity of FeSO4·7H2O (0.5 mM) was introduced into the cell just before electrolysis. The electrodes were connected to a DC power supply (Metrix, model AX 322, Chauvin Arnoux Group, Paris, France) operating in galvanostatic mode to control the current intensity at a value of 500 mA. The ionic strength was maintained constant by the addition of 0.05 M Na2SO4 as the supporting electrolyte. The electrolytic solution was in circulation with the help of a peristaltic pump (flow rate of 2·L min−1). The temperature was maintained at 18 °C and the initial sulfamethazine concentration was 0.2 mM, for pure SMT solution and after appropriate dilution of the industrial effluent to achieve this concentration.
2.4.2 Biological treatment
Culture media were prepared in duplicate in 500-mL serum bottles containing 200 mL of the sulfamethazine synthetic solutions or the pharmaceutical effluent, beforehand pretreated by the electro-Fenton process. The initial composition (mg·L−1) of the mineral solution was: Na2HPO4, 334; K2HPO4, 208; KH2PO4, 85; CaCl2, 27.4; MgSO4.7H2O, 22.6; NH4Cl, 2; FeCl3.6H2O, 0.26; the initial pH was adjusted to 7. Activated sludge was added in order to have an initial concentration of 1 g·L−1 of dry matter. Cultures were agitated at room temperature (25 °C) and 5-mL samples were taken regularly, filtered through 0.45-μm-syringe filters and injected for TOC measurements.
3 Results and dsiscussion
3.1 Pretreatment of SMT by the electro-Fenton process
The degradation of sulfamethazine by the electro-Fenton process using a carbon felt cathode and a platinum anode has been previously studied under various experimental conditions including current intensity, temperature and initial sulfamethazine concentration [18]. The corresponding results showed that an initial SMT concentration of 0.2 mM appeared to be a good compromise between the initial concentration level and the degradation yield, an increase of the temperature above 18 °C appeared not really relevant with respect to the increase of the operating costs, while the increase of the degradation yield remained limited. The optimal initial ferrous concentration and current intensity appeared to be 0.5 mM and 500 mA, respectively. These optimal conditions led to a rapid degradation until 100% of elimination after 30 min of pretreatment (Fig. 1a), while mineralization remained limited, 2.1 and 18.1% for 30 and 60 min electrolysis times (Fig. 1a), as well as rather low levels of oxidation (28.4 and 55.6% from an initial amount of 88 mg·L−1 O2 after 30 and 60 min of pretreatment, respectively) (Fig. 1b), which suggested the formation of organic intermediate products, as confirmed by the examination of the HPLC profiles [20], showing that the disappearance of SMT was accompanied by the formation of several intermediate products, due to the oxidation of the generated products by hydroxyl radicals [21].
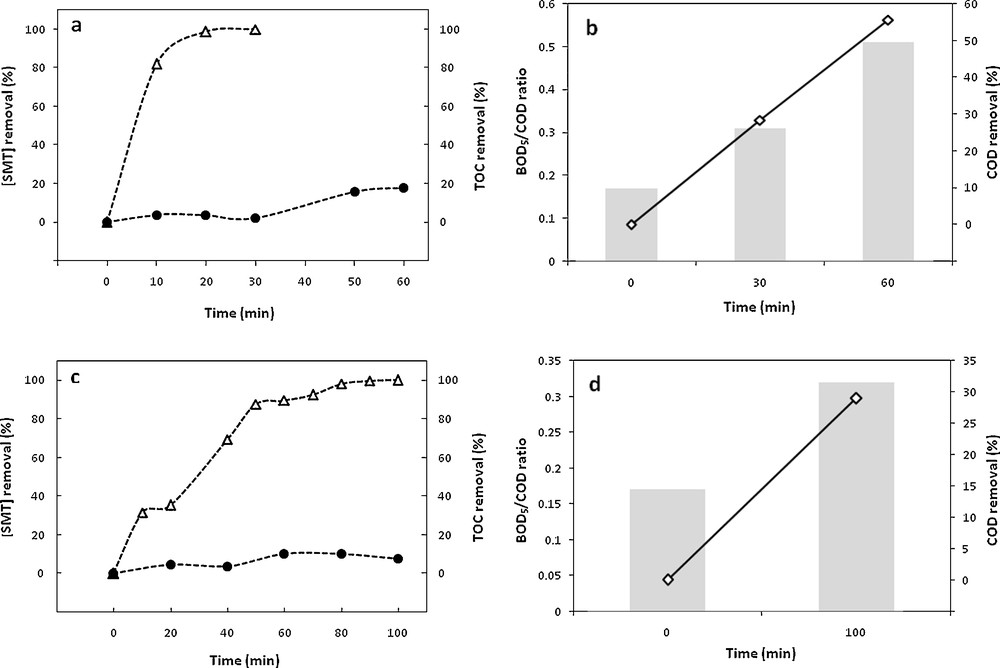
Time evolution of SMT removal () and TOC removal () (a and c), BOD5/COD ratio (column bars) and COD removal (◊) (b and d) during electro-Fenton pretreatment in the following experimental conditions: [Fe2+]0 = 0.5 mM, [Na2SO4] = 50 mM, pH = 3, T = 18 °C, I = 500 mA, V = 1 L; 0.2 mM was considered for the initial SMT concentration, for pure SMT solution and after appropriate dilution of the industrial effluent.
3.2 The biodegradability of SMT
In order to follow the evolution of the biodegradability profile during the electro-Fenton pretreatment, the samples were analyzed for BOD5 and COD. As can be seen, the measurement of the biodegradability of the target molecule led to a value of 0.17 for the BOD5/COD ratio (Fig. 1b), confirming the recalcitrance of SMT and the need for its oxidation prior to a biological treatment, while the ratio increased to 0.31 and 0.51 for 30 and 60 min of electrolysis (Fig. 1b), namely above the limit of biodegradability after only 1 h of pretreatment [22].
3.3 Biodegradation of the electrolyzed solution of SMT
Owing to biodegradability improvement, activated sludge cultures were carried out on an SMT solution pretreated during the time needed for its total degradation, namely 30 min (Fig. 2). It should be remembered that in the absence of pretreatment, the SMT recalcitrance was previously confirmed since no degradation was observed even after 20 days of activated sludge culture [20]. Regarding the pretreated SMT solution, almost constant TOC values were observed until nearly the 10th day of culture, showing obviously an absence of possible biosorption of the degradation products (Fig. 2). Then the mineralization yield increased significantly until reaching 61.4% on the 18th day of culture. It can be assumed that at the beginning of culture, microorganisms were not able to assimilate the by-products generated during the degradation of SMT by the electro-Fenton process. The mineralization observed from the 10th day of culture was most likely characteristic of the acclimation of activated sludge to the degradation products, in agreement with previous results recorded during the treatment of a pesticide, phosmet, by means of a combined process coupling an electrochemical pretreatment with a biological treatment [22]. The overall mineralization efficiency of the combined process was therefore 62.2% for the SMT solution pretreated during 30 min, of which 2.1% corresponded to the electro-Fenton pretreatment and 61.4% to the biological treatment.
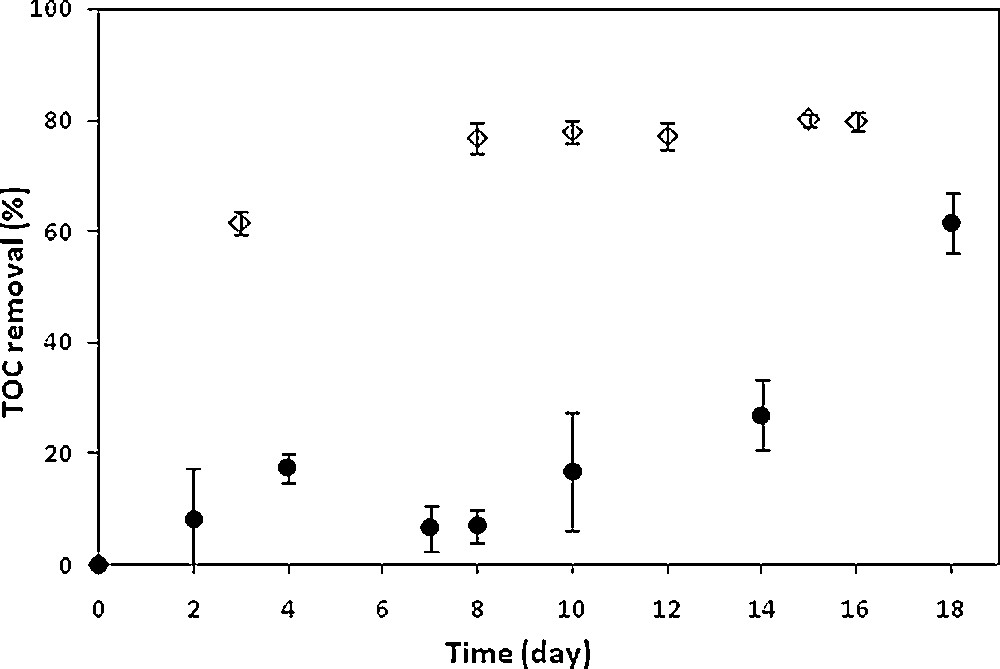
Time-courses of activated sludge cultures of solutions of synthetic SMT pretreated during 30 min (•) and industrial effluent pretreated during 100 min (◊). Electro-Fenton pretreatment conditions: [Fe2+]0 = 0.5 mM, [Na2SO4] = 50 mM, pH = 3, T = 18 °C, I = 500 mA, V = 1 L and 0.2 mM was considered for the initial SMT concentration, for pure SMT solution and after appropriate dilution of the industrial effluent.
3.4 Application to an industrial pharmaceutical effluent
The optimal conditions determined previously for the SMT degradation by an electro-Fenton process (0.2 mM SMT, 0.5 mM Fe2+, 500 mA and 18 °C) were applied to an industrial pharmaceutical effluent (Fig. 1c) and showed that nearly 100 min were needed for total SMT removal. Besides, a slower SMT degradation can be noticed in the industrial effluent if compared to the synthetic solution, while the initial SMT amount was the same in both solutions; this behavior might be attributed to the competitive consumption of hydroxyl radicals by other organic compounds contained in the real effluent. A low level of mineralization was concomitantly observed (7.5%–Fig. 1c), as well as a relatively low level of oxidation (28.9% from an initial amount of 566 mg·L−1 of O2) (Fig. 1d); this result can be explained by the generation of intermediate compounds simultaneously with the degradation of the target compound, as confirmed at the examination of the HPLC profiles (data not shown). On the other hand, the industrial effluent showed a low BOD5/COD ratio (0.17) (Fig. 1d), indicating·low biodegradability and similar to that obtained for synthetic SMT solution, confirming the need for pretreatment prior to biological treatment. Indeed, the SMT represented roughly 15% of the COD and COT in the real effluent. Even if other organic compounds were present in significant amounts in the effluent, the biodegradability value (0.17) similar to that obtained for the synthetic SMT solution seemed to indicate that effluent recalcitrance should be related to the presence of SMT.
Prior to the biological treatment step, possible biosorption on activated sludge of the organic content of the industrial effluent was assessed. Two hundred and fifty milliliter Erlenmeyer flasks containing 100 mL of medium were inoculated with 0.5 g·L−1 of activated sludge, which was washed in the same conditions as the biological treatment. The flasks were stirred at 250 rpm and the experiments were triplicated to ensure the reproducibility of the results. Samples were regularly taken over a 5-h period, filtered on 0.45 μm and then analyzed by TOC measurements. As displayed in Fig. 3, no significant TOC decrease was detected within 5 h of experiment, showing the absence of organic material biosorption. Indeed, since biosorption is a rapid phenomenon, it would have been noticed within 5 h.
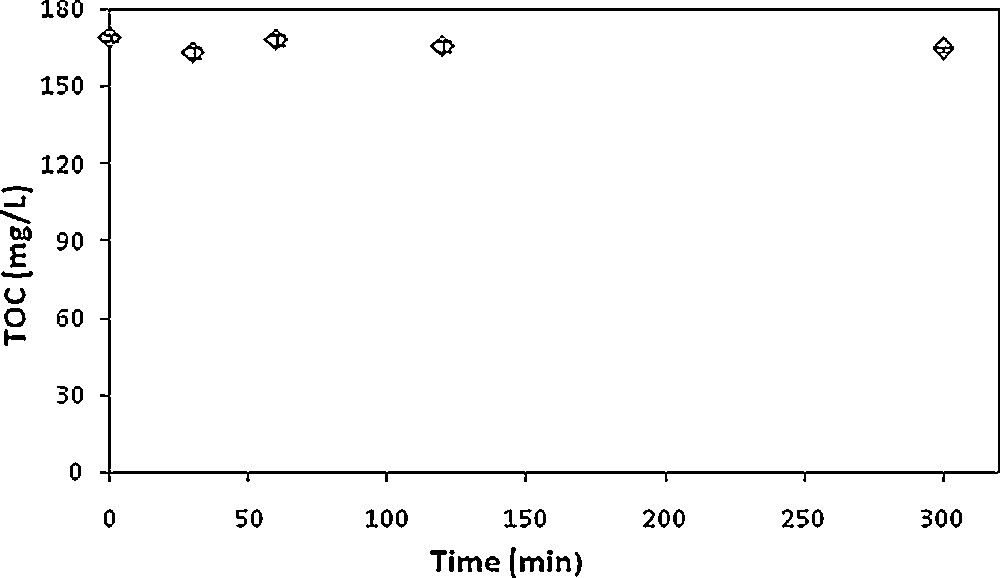
Biosorption study of the organic content of the industrial effluent on activated sludge (1 g·L−1).
After 100 min of electro-Fenton oxidation, the BOD5/COD ratio increased to 0.32, showing the efficiency of the pretreatment; therefore and even if the limit of biodegradability (0.4) was not reached, activated sludge culture was carried out for 15 days on the pretreated effluent, since SMT recalcitrance was clearly shown and hence the positive impact of electro-Fenton pretreatment [20]. In view of the comparison with the synthetic effluent, a pretreatment time of 100 min was chosen, since it corresponded to a total SMT degradation.
High levels of mineralization were observed during the first days of culture until reaching 76.8% within about eight days for the non-pretreated industrial effluent and after electrolysis respectively, showing the impact of the pretreatment and confirming that effluent biorecalcitrance was related to the presence of SMT instead of the organic compounds present in the effluent. This behavior can be attributed to a readily assimilation of some of the degradation products by microorganisms [23]. No further significant mineralization was observed between day 8 and day 15; the mineralization yield increased only slightly to reach 79.9% on the 15th day of biological treatment.
It should be noticed that the differences in the observed trends between the synthetic solution and the industrial effluent were not in disagreement with the similar BOD5 on COD ratio obtained for both solutions, 0.17, owing to the difference in the initial amounts of activated sludge inoculated, 1 g·L−1 for the biological treatment and 0.05 g·L−1 for BOD5 experiments.
A higher level of mineralization was obtained during the biological treatment of the pretreated industrial effluent if compared to that of the pretreated synthetic solution, 79.9 and 61.4% after 15 and 18 days respectively (Fig. 2), which should also be related to the organic content of the industrial effluent apart of SMT.
From this, the overall mineralization efficiency of the combined process achieved a value of 81.4% for the industrial pharmaceutical effluent pretreated during 100 min, of which 7.5% corresponded to the electro-Fenton pretreatment and 79.9% to the biological treatment (Table 2).
TOC removal by combined electro-Fenton and biological treatment1.
Untreated | Electro-Fenton pretreatment | Biological treatment | Total % removal | ||||
TOC (mg·L−1) | Time (min) | TOC (mg·L−1) | % removal | TOC (mg·L−1) | % removal | ||
SMT | 27.6 | 30 | 27.0 | 2.1 | 10.6 | 61.4 | 62.2 |
27.6 | 60 | 22.6 | 18.1 | 5.1 | 78.8 | 81.3 | |
Industrial effluent | 188.7 | 100 | 174.4 | 7.5 | 21.5 | 79.9 | 81.4 |
The results dealing with the industrial effluent suggested therefore a more complex strategy to achieve total effluent mineralization. For this purpose, a final electro-Fenton treatment of the biologically treated solution could be considered. The corresponding work is in progress in the laboratory.
4 Conclusion
The relevance of the coupling of electro-Fenton pretreatment with a biological treatment for the elimination of a veterinary antibiotic, sulfamethazine (SMT), from an aqueous medium was investigated. The pretreatment was carried out under the best operating conditions, namely an initial SMT concentration of 0.2 mM, a concentration of ferrous ions of 0.5 mM, at pH 3, 18 °C and a 500-mA current intensity. Despite a total SMT degradation obtained after only 30 min of electrolysis, the low levels of oxidation and mineralization, 28.4% for the former and 2.1% for the latter after 30 min of electrolysis, indicated the formation of organic intermediate products. However, after subsequent biological treatment, a mineralization yield of 61.4% was obtained for the pretreated solution.
In a second step, an industrial effluent, supplied by a pharmaceutical industry located in northern Tunisia, was pretreated under the same operating conditions, showing a total SMT degradation after 100 min of oxidation, while mineralization remained limited (7.5%). During the subsequent biological treatment, TOC removal increased significantly, to reach 79.9% on the 15th day, which lead to an overall mineralization efficiency of the combined process of 81.4%, confirming its relevance, even for the treatment of industrial effluents.