1 Introduction
Acetaminophen, or paracetamol (N-acetyl-p-aminophenol), is an antipyretic and minor analgesic drug that practically has no anti-inflammatory action [1]. It is non-carcinogenic and an effective substitute for aspirin to be used for the patients who are sensitive to aspirin, and it is safe up to therapeutic doses [2]. However, overdoses will cause liver and kidney damage and finally lead to death [3]. Dopamine [4-(2-aminoethyl) benzene-1,2-diol (DA)], the most significant among the class of catecholamines, plays an important role in the function of the central nervous, cardiovascular, renal and hormonal systems [4]. Insufficient DA concentration due to the loss of DA-producing cells may lead to a disease called Parkinson's disease [5]. Vitamin C or ascorbic acid (AA), a water-soluble vitamin, is widely present in many biological systems and in multivitamin preparations [6]. Ascorbic acid (AA) is very popular for its antioxidant properties, and is present in the human diet as a vital vitamin. Moreover, it is also used for the prevention and treatment of common cold, mental illness, infertility, cancer and AIDS [7]. Ascorbic acid is known for its reductive properties (antioxidant activity). Hence, it represents an important quality indicator that contributes to the antioxidant properties of food [8].
AC, DA and AA are electrochemically active compounds and can be determined by electrochemical techniques [9]. AA has an oxidation potential similar to that of DA. These facts can largely affect the determination of DA in the presence of AA [10]. On the other hand, important drugs, such as AC will interfere with catecholamine measurements in biological samples [2]. Thus, a major problem is that the oxidation peaks of AA and DA appear almost at the same potential at an unmodified electrode, resulting in overlapping voltammetric responses and making their discrimination highly difficult. Therefore, electrochemically individual and/or simultaneous determinations of AC, DA and AA on traditional electrodes are very difficult [11]. For this purpose, many attempts have been made with a variety of electrodes for the selective determination of DA in the presence of AA and/or AC [12–18]. He et al. [9] have studied the use of a Cu–zeolite A/graphene-modified glassy carbon electrode for simultaneous electrochemical determination of dopamine and ascorbic acid. Zhang et al. reported that layer-by-layer assembled CNTs modified electrodes have good selectivity to DA in the presence of AA [19]. Yang et al. [20] proposed an AuNPs@polyaniline core–shell nanocomposite-modified electrode for simultaneous electrochemical determination of dopamine and ascorbic acid. Chen and Chzo [21] have studied the simultaneous voltammetric detection of dopamine and ascorbic acid using didodecyl. Tavakkoli et al. have proposed an electrocatalytic determination of AA using a palladium-coated nanoporous gold film electrode [22]. Zare et al. have reported the application of a hematoxylin multi-wall carbon nanotube-modified carbon paste electrode (HMWCNT–CPE) as a chemical sensor for simultaneous determination of DA and AC [23]. A survey of the literature shows that there are only few electrochemical methods for the simultaneous determination of AC, DA and AA. Wang et al. [10] reported the electrocatalytic response for the oxidation of AC on carbon-coated nickel magnetic nanoparticle-modified electrodes and the simultaneous determination of AC, DA, and ascorbic acid.
Carbon paste electrode (CPE) was introduced by Adams in 1958. The ease and speed of preparation, the creation of a new producible surface and the low cost of carbon paste are some advantages of CPEs over other electrodes [24]. In further researches, a wide variety of modifiers has been used with these versatile electrodes [25]. Carbon paste electrodes are convenient conductive matrixes for preparing chemically modified electrodes (CMEs) by the simple mixing of a graphite/binder paste and a modifier [26]. The modified electrode has good electrocatalytic activity, sensitivity, and selectivity; it has also a low detection limit compared to traditional carbon paste electrodes [27]. Recently, attention has been paid to develop a new generation of modified electrodes involving Schiff bases and nanoparticles due to the unique properties of these materials [17]. Nanoparticles are the most widely employed to prepare CMEs owing to their excellent physical and catalytic properties [28–30].
In the current work, a simple and fast procedure was used for the fabrication of a carbon paste electrode modified with PbS nanoparticles and a Schiff base (Fig. 1) (PSNSB/CPE). The obtained electrode, suitable for the simultaneous determinations of AC, DA and AA by anodic differential pulse voltammetry in phosphate buffer solution as the supporting electrolyte, is described. It is also intended to evaluate this sensor for simultaneous determinations of AC, DA and AA in some commercial pharmaceutical samples.
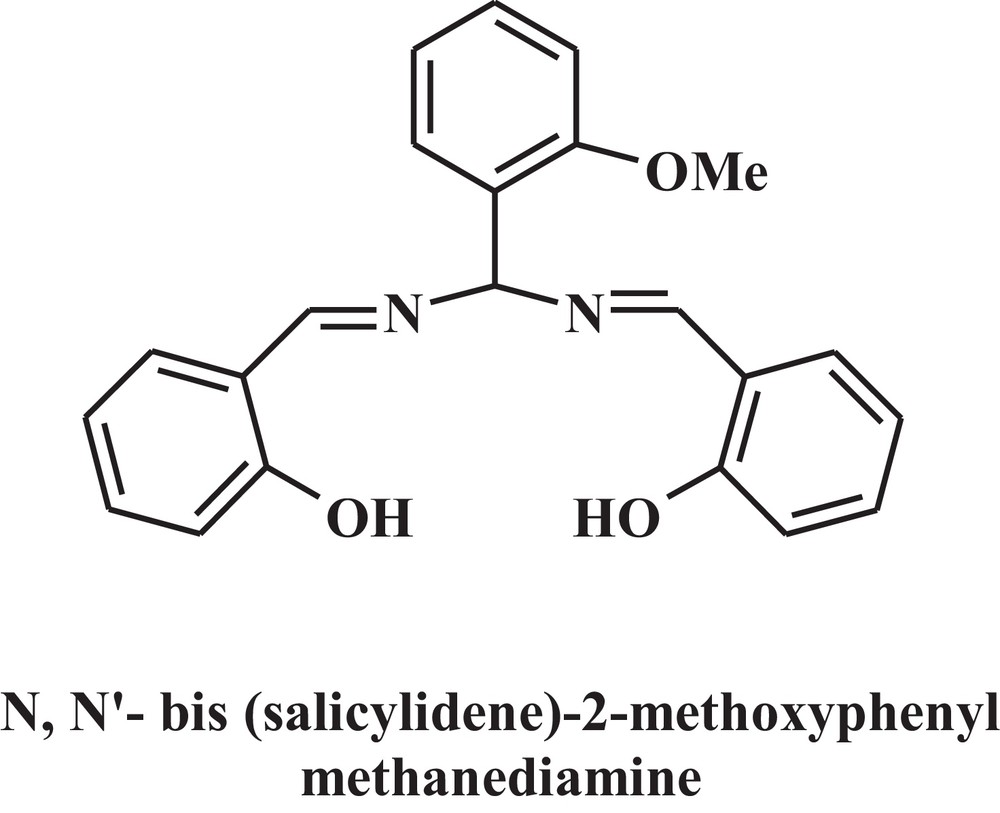
Chemical structure of the used Schiff base.
2 Experimental
2.1 Reagents and chemicals
All solutions were freshly prepared with double distilled water. AC, DA, AA and all other reagents were of analytical grade and had been purchased from Merck (Darmstadt, Germany). Graphite powder and paraffin oil (DC 350, density = 0.88 g·cm−3) as the binding agent (both from Merck) were used for preparing the pastes. The buffer solutions were prepared from orthophosphoric acid and its salts in the pH range between 2.0 and 10.0 [8].
2.2 Apparatus
The electrochemical experiments were carried out using an AUTOLAB PGSTAT-30 (potentiostat/galvanostat) equipped with a USB electrochemical interface and driven by a GPES 4.9 software package (Eco Chemie, The Netherlands) in conjunction with a three-electrode system and a personal computer for data storage and processing. A three-electrode cell system composed of an Ag/AgCl (3.0 M KCl) electrode as the reference electrode, a platinum wire as the auxiliary electrode, and the PSNSB-modified CPE (geometric surface area = 1.34 mm2) as the working electrode was employed for the electrochemical studies. A copper wire was inserted through the composite end of the working electrode to establish the electrical contact. All potentials noted in this article are reported with respect to the Ag/AgCl reference electrode. A Metrohm 827 pH/ion-meter was used for pH measurements. X-ray diffraction (XRD) patterns were recorded using a Rigaku D-max C III, X-ray diffractometer using Ni-filtered Cu Kα radiation. Scanning electron microscopy (SEM) images were obtained with LEO instrument model 1455 VP.
2.3 Preparation of PbS nanoparticles
In a typical synthesis, 1.00 g (0.003 mol) of Pb(NO3)2 powder was dissolved in 100 mL of distilled water and 100 mL of 0.09 M thioglycolic acid (TGA) were mixed slowly under stirring, while pH was maintained at 10 with NaOH 1.00 M. After stirring, the reactants were poured into a 250-mL Teflon-lined autoclave, which was maintaining at 80–16 °C for 5–12 h and then cooled down to room temperature naturally. The product was centrifuged, washed with alcohol and distilled water for three times, and dried in the air at 50 °C for 10 h [31].
2.4 Synthesis of the Schiff base
The Schiff base was synthesized according to the following procedure; NH4OAc was added to a mixture of salicylaldehyde (0.38 g, 3 mmol) and arylaldehyde (1.5 mmol) (0.25 g, 3.27 mmol) in the presence of NEt3 (0.12 mL) as a base by stirring in one portion. The progress of the reaction was monitored by TLC. After the completion of the reaction, oil pail yellow substance was obtained. Then, by dissolving the oil yellow mixture in 1.5 mL of MeOH and cooling for one night, a yellow solid was precipitated. The solid product was filtered off and washed with cold MeOH. The crude product was purified by recrystallization in ethanol and the pure Schiff base, N,N’-bis(salicylidene)-arylmethanediamine was obtained in high to excellent yields. The Schiff base products were identified by physical and spectroscopic data. The results are as follows:
Yellow solid; mp 145–147 °C; IR (KBr)/m (cm−1) 3375–3625 (br, OH), 1625 (s, CN), 1450, 1510 (Ar); 1H NMR/DMSO/d ppm: 2.3 (3 H, OCH3), 6.2 (s, 1 H, NCHN), 6.7–7.4 (m, 12 H), 8.6 (s, 2 H, HCN),12.90 (s, 2 OH); 13C NMR/DMSO/d ppm: 60, 90, 117, 119, 119.2, 129.5, 130.2, 130.3, 138, 165, 170; UV (CHCl3)/λmax (nm) 324 (w), 262 (s); MS: m/z = 361 (M++1, 2), 360 (M+, 6), 241 (50), 240 (80), 120 (70), 119 (100), 77 (15); Anal. Calcd. For C. H. N: 73.3 (C), 5.5 (H), 7.8 (N); Found: 72.69 (C), 5.48 (H), 8.07 (N).
2.5 Preparation of the electrode
The PSNSB/CPE was prepared by hand-mixing 0.003 g of Schiff base with 0.5 g of graphite powder and 0.004 g of PbS nanoparticles with a mortar and a pestle. Then, ∼ 0.7 mL of paraffin oil was added to the above mixture and mixed for 20 min until a uniformly wetted paste was obtained. A portion of the paste was put into plastic syringe tubes with an inner diameter of 2.0 mm to form the PSNSB/CPE. Electrical contact was established with the paste by inserting a copper wire into the plastic syringe tube. The surface was smoothed on a piece of weighing paper. A new surface can be obtained by pushing an excess of paste out of the tube, removing this excess, and mechanically polishing the electrode surface. For comparison, Schiff base (SB) modified CPE electrode (SB/CPE) without PbS nanoparticles (PSN), PSN carbon paste electrode (PSN/CPE) without SB, and unmodified CPE in the absence of both SB and PSN were also prepared in the same way.
3 Results and discussion
3.1 XRD and SEM study of PbS nanoparticles
The phase composition and phase structure of the as-obtained sample was examined by XRD. As shown in Fig. 2, all peaks can be readily indexed as corresponding to face-centered-cubic PbS structure with a lattice constant a = 59.41 nm, in agreement with the literature value (JCPDS Card No. 77-0244, a = 59.34 nm). The strong and broad peaks show that the material has good crystallization and small size. No impurity, such as PbS2O3 and PbSO3 were found in Fig. 2, implying that the product is pure.
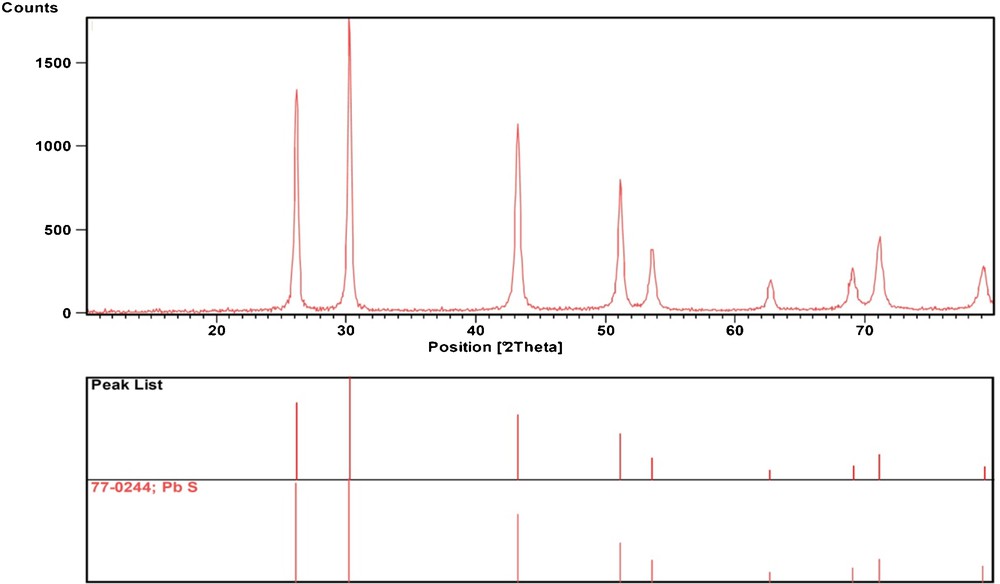
XRD pattern of the PbS nanoparticles.
Fig. 3 shows the SEM image of as-synthesized PbS nanoparticles. According to Fig. 3, the average particle size of PbS nanoparticles was 40–50 nm.
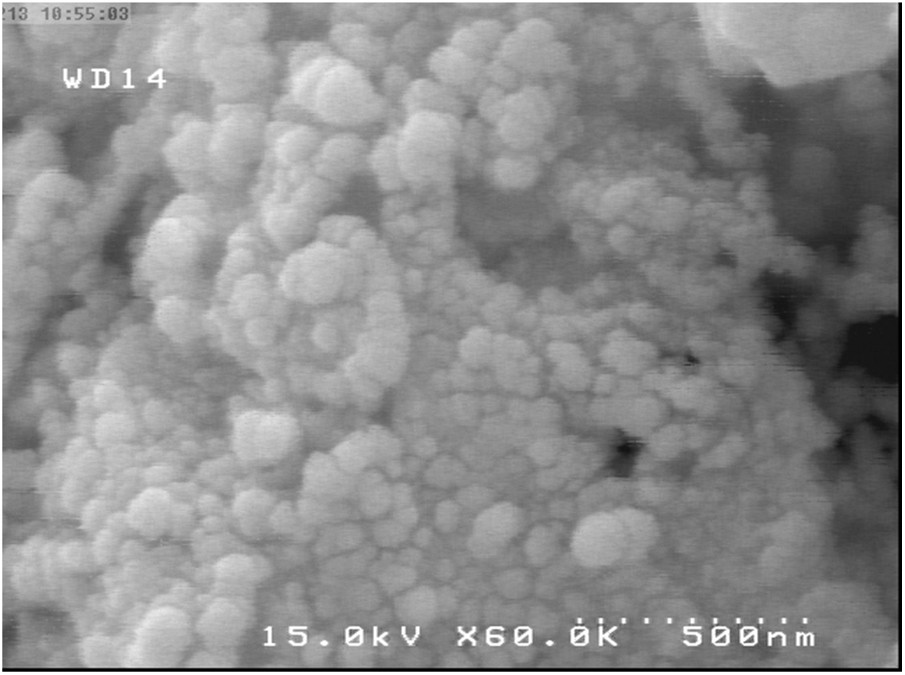
SEM image of as-synthesized PbS nanoparticles.
3.2 Electrochemistry of AC, DA and AA at bare or different modified electrodes
Our primary study showed that the electrochemical detection of AC, DA and AA in the presence of one another on an unmodified CPE electrode due to overlapped voltammetric responses and low currents is difficult (Fig. 4a). The reason of low currents is the absence of electrocatalytic behavior of bare electrodes toward AC, DA, and AA. To solve this problem, the CPE was modified with PbS nanoparticles as the operating electrocatalytic feature. Fig. 4b shows the cyclic voltammograms (CVs) of the same compounds at the surface of the PSN/CPE. As can be seen in Fig. 4b, the anodic peak current is enhanced by approximately 24-fold compared to the anodic peak current on the unmodified CPE electrode, but an overlapped voltammetric response, which is the same as that with an unmodified CPE, was observed for PSN/CPE. Trying to find a suitable method for the separation of these three compounds, a Schiff base (Fig. 1) was chosen for the modification of the CPE electrode. Cyclic voltammograms obtained in Fig. 4c show that the anodic peak potential of AC, DA and AA at the surface of SB/CPE shifts from 702 mV, 596 mV and 587 mV at the bare CPE to 674 mV, 393 mV and 171 mV at the SB/CPE respectively. On the other hand, the anodic peak currents of three compounds are enhanced by approximately 10-fold in comparison with the anodic peak current on unmodified CPE. Comparison of PSN/CPE with SB/CPE show that PSN/CPE can increase the anodic peak currents better than SB/CPE, but that the separation between the anodic peak potentials of AC, DA and AA in this electrode can not be seen. Therefore, we think that both problems of overlapped voltammetric response and low currents may be solved with adding PbS nanoparticles and Schiff base simultaneously during the preparation of the electrode. Fig. 4d shows that the anodic peak potential of AC, DA and AA at the surface of PSNSB/CPE shifts in a similar way to the case of SB/CPE, and that the anodic peak current is enhanced by approximately 24-fold compared to an unmodified CPE electrode. Therefore, our guess was right and it can be concluded that PbS nanoparticles and the Schiff base have a synergic effect on the electrocatalytic oxidation of AC, DA, and AA at the surface of PSNSB/CPE. A comparison of the peak separations of the four electrodes is also given in Table 1.
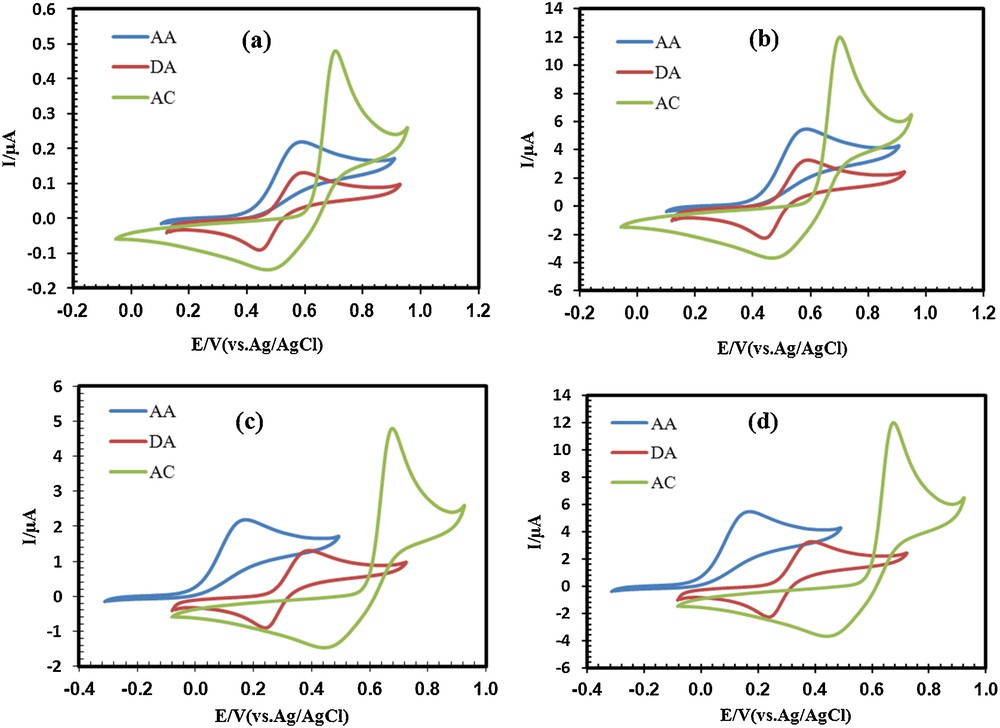
(Color online.) Cyclic voltammograms of AC (33 μM), DA (20 μM) and AA (125 μM) at (a) a bare CPE, (b) (PSN/CPE), (c) (SB/CPE) and (d) (PSNSB/CPE) in a 0.1 M phosphate buffer solution (pH 4.6), scan rate: 100 mV·s−1.
Comparison of peak separation of unmodified CPE with three modified electrodes.
Electrode | E (mV) | ||
AC | DA | AA | |
Unmodified CPE | 702 | 596 | 587 |
PSN/CPE | 698 | 593 | 584 |
SB/CPE | 674 | 393 | 171 |
PSNSB/CPE | 672 | 390 | 168 |
3.3 Optimization of the preparation conditions of the electrode
As it was mentioned in Section 3.2, both PbS nanoparticles and Schiff base can affect the electrochemical response of the electrode. Therefore, the effect of the amount of PbS nanoparticles and Schiff base in constructing the electrode must be optimized. For this investigation, differential pulse voltammetry (DPV) was carried out in the potential range between –300 and 900 mV. Fig. 5a shows the effect of the amount of PbS nanoparticles used for constructing of electrode on the anodic peak currents of Ac, DA, and AA. According to these results, the maximum current for the three species was obtained for 0.004 g of PbS nanoparticles. Therefore, the electrodes with this amount of PbS nanoparticles were used for further studies. It should be noted that the amount of Schiff base in constructing all the electrodes during this step was 0.003 g.
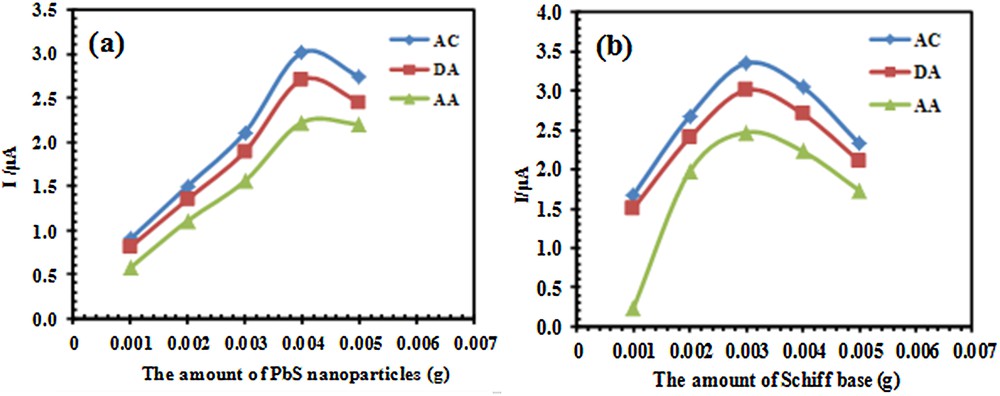
(Color online.) Effect of the amount of (a) PbS nanoparticles and (b) Schiff base on a peak current of 33 μM AC, 20 μM DA and 125 μM AA after the modification of the PSNSB/CPE, in a 0.1 M phosphate buffer solution (pH 4.6).
The amount of Schiff base for the construction of the electrodes and its effect on the current was also investigated. The results in Fig. 5b indicated that, the maximum current was obtained with addition of 0.003 g of Schiff base to graphite containing 0.004 g of PbS nanoparticles for PSNSB/CPE construction.
3.4 Effect of pH
The pH of the electrolyte solution has a significant influence on the oxidation of AC, DA, and AA at PSNSB/CPE, by varying both the peak current and the peak potential. The influence of the pH of the solution on the peak currents and peak potentials in the electrocatalytic oxidation of AC (33 μM), DA (20 μM) and AA (125 μM) at the PSNSB/CPE was investigated by DPV in the pH range from 2 to 10 (Fig. 6). The results displayed in Figs. 6 and 7a showed that the maximal current response was observed at about pH 4 for the three molecules.
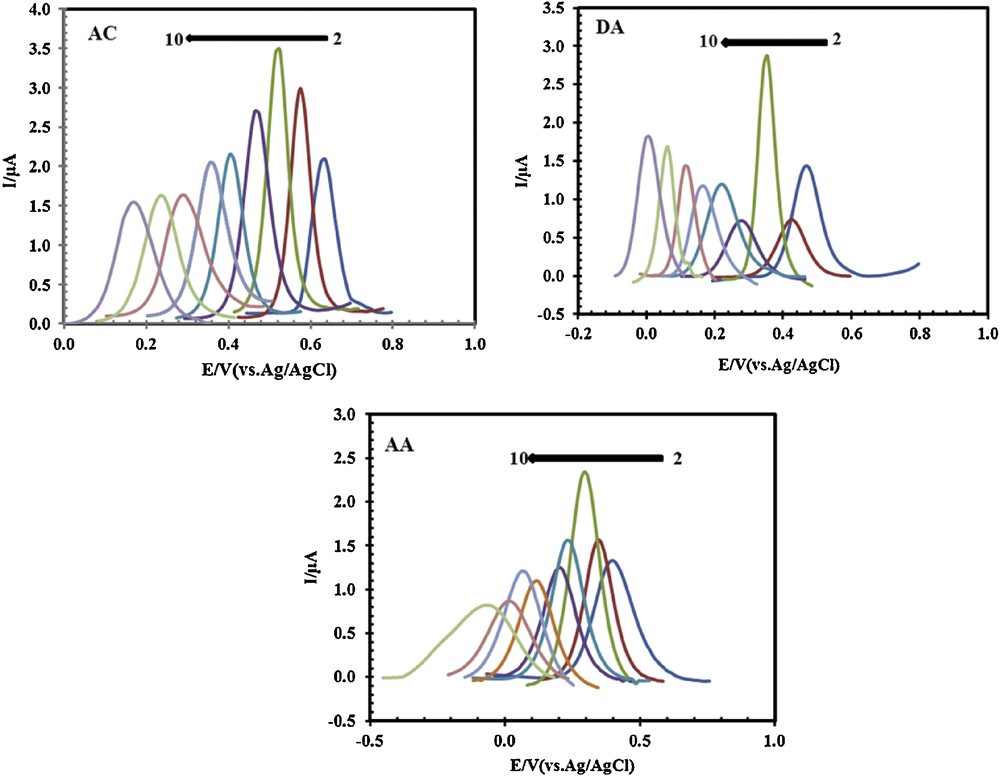
(Color online.) Differential pulse voltammograms of 33 μM AC, 20 μM DA, and 125 μM AA, recorded for different pH values from 2 to 10.
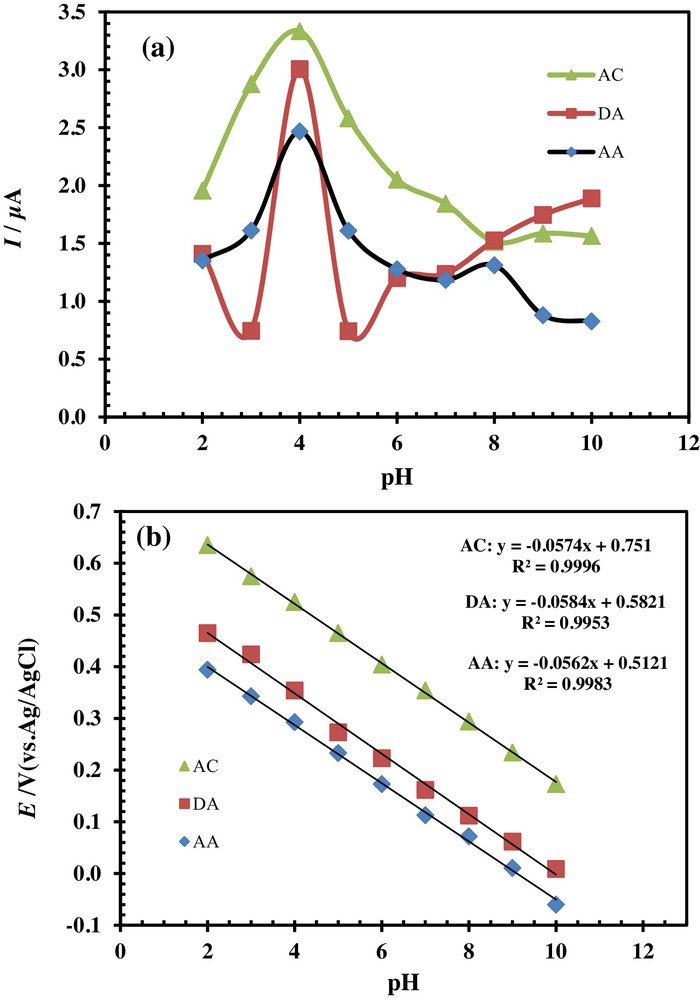
(Color online.) Effects of pH on (a) the peak current and (b) the potential of 33 μM AC, 20 μM DA and 125 μM AA in a 0.1 M phosphate buffer solution at the PSNSB/CPE.
It is obvious from Fig. 6 that with increasing the pH of the solution, the oxidation peak potentials of the three molecules shift to negative values. The potential diagram was constructed by plotting the graph of Epa vs pH of the solution (Fig. 7b). The graph shows a good linearity with slopes of 57.4, 58.4 and 56.2 mV/pH for AC, DA, and AA, respectively. The Nernst equation is nearly obeyed for a transfer reaction of equal numbers of electrons and protons [32,33].
3.5 Effect of the scan rate
A study on the effect of the scan rate on the response of PSNSB/CPE toward the oxidation of AC, DA, and AA showed that the cyclic voltammograms (CVs) of AC, DA and AA depended on the scan rate. The typical illustrative CVs of AC and DA are shown in Fig. 8 (CVs of AA not shown). As can be observed from Fig. 8, the oxidation peak potential shifted to more positive potentials with increasing the scan rate, confirming the kinetic limitation in the electrochemical reaction. Also, a plot of peak height (Ip) versus the square root of scan rate (ν1/2), in the range of 25–350 mV·s−1 was constructed (Fig. 8, inset (a)). The redox peak current increases linearly with the increase of the square root of the scan rate, demonstrating the diffusion-controlled process of AC and DA on the PSNSB/CPE [11]. A Tafel plot was drawn from the data of the rising part of the current–voltage curve recorded at a scan rate of 100 mV·s−1. This part of the voltammogram, known as Tafel region, is affected by electron transfer kinetics between substrates (AC, DA and AA) and PSNSB/CPE. Using such plots, the values of the anodic electron transfer coefficient were determined to be 0.46, 0.62, and 0.71 for AC, DA and AA, respectively.
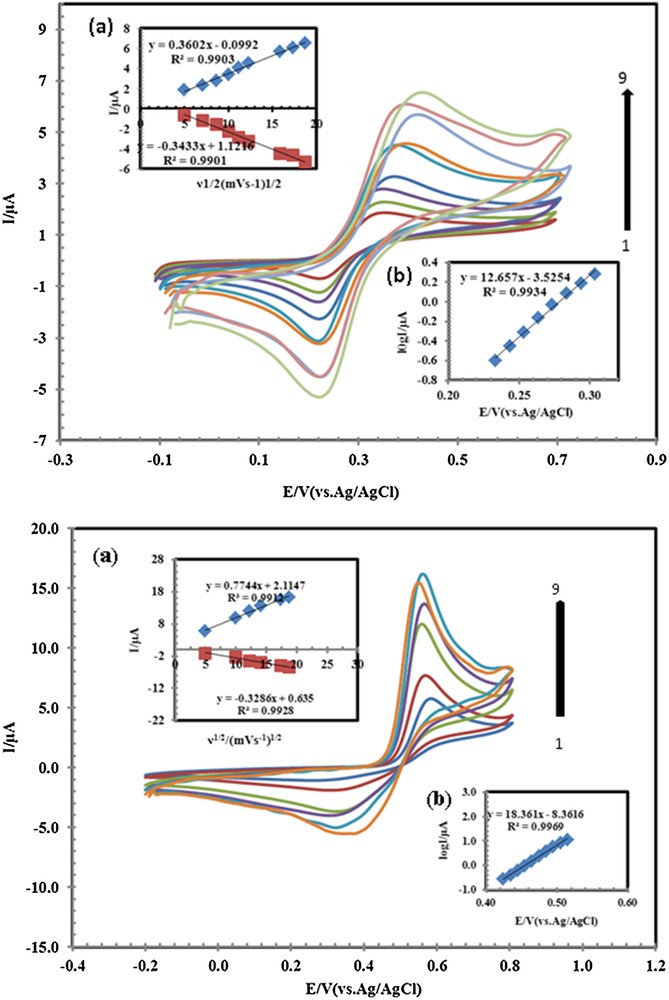
(Color online.) Cyclic voltammograms of 33 μM AC and 20 μM DA at PSNSBCPE in 0.1 M PBS (pH 4.6), at various scan rates, from inner to outer, 25, 50, 75, 100, 150, 200, 250, 300 and 350 mV·s−1. Insets: (a) variation of Ip vs scan rate; (b) plot of log (peak current) vs potential.
3.6 Chronocoulometric measurements
The diffusion coefficient of AC, DA and AA in an aqueous buffered solution (pH 4.6), during its electrocatalytic oxidation at the PSNSB/CPE surface was calculated using chronocoulometry method. The chronocoulometric responses for various concentrations of AC, DA and AA at PSNSB/CPE are shown in Fig. 9a. The plot of Q against t1/2 for different concentrations of AC, DA and AA is shown in Fig. 9b. The slopes of the resulting straight lines were plotted against the AC, DA and AA concentration (Fig. 9c). The apparent diffusion coefficient (D) of AC, DA and AA was calculated to be about 3.55 × 10−6, 1.92 × 10−6 and 8.65 × 10−6 cm2·s−1, respectively.
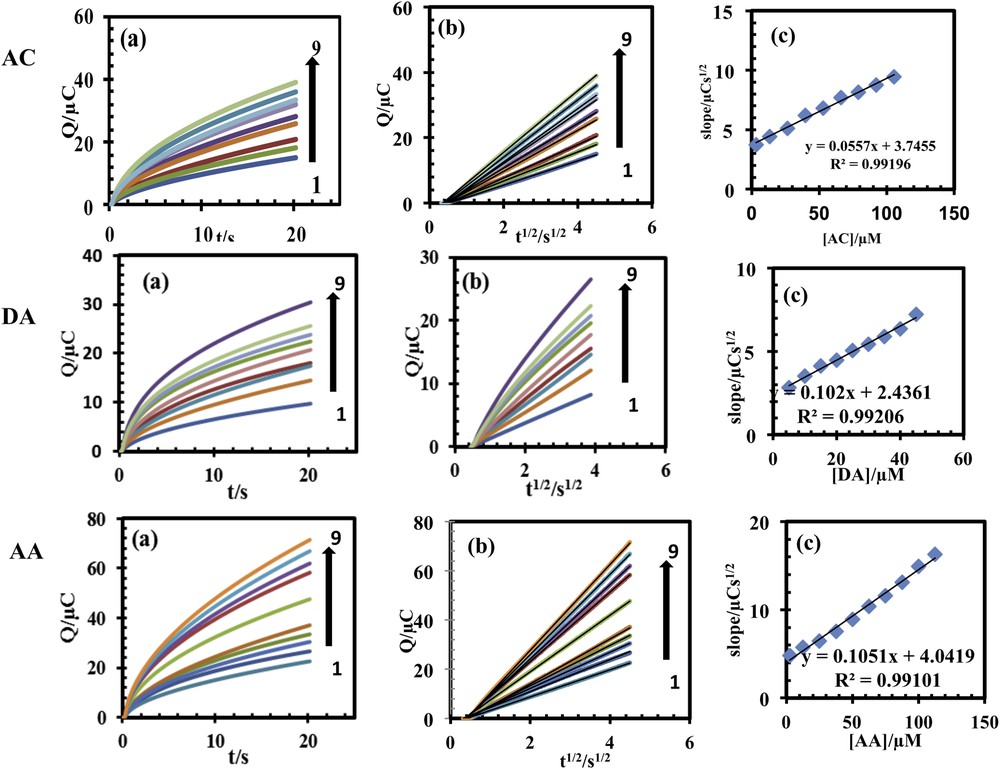
(Color online.) (a) Chronocoulomograms obtained with PSNSBCPE in in 0.1 M PBS (pH 4.6) for different concentrations of AC, DA and AA. Numbers 1–9 correspond to: 3.2, 13.2, 26.4, 40.0, 53.0, 66.0, 80.0. 92.5, 105.5 μM AA; 5.0, 10.0, 15.0, 20.0, 25.0, 30.0, 35.0, 40 and 45.0 μM DA and 2.5, 12.5, 25.0, 3735, 50.0, 62.5, 75.0, 87.5, 100.0, 112.5 μM AA; (b) plots of the charge (Q) vs square root of the time (t1/2) obtained from chronocoulomograms 1-9; (c) plot of the slope of straight lines against the AC, DA and AA concentration.
3.7 Simultaneous determination of AC, DA, and AA
One of the main objectives of the present study was the development of a modified electrode capable of the electrocatalytic oxidation of AC and separation of the electrochemical responses of AC, DA, and AA. The simultaneous determination of AC, DA and AA in the mixture was carried out at PSNSB/CPE when the concentration of one of the three compounds was changed while keeping the concentrations of the other two constant. The results are shown in Fig. 10a–c. The peak currents for AC, DA, and AA increased linearly with increases in their respective concentrations without considerable effects on the other peak currents. Examination of Fig. 10a shows that the peak current of AC increased with an increase in the AC concentration, while the concentrations of DA and AA were kept constant (40.0 μM and 25.0 μM). Similarly, as shown in Fig. 10b, keeping the concentration of AC and AA constant (20.0 μM AC and 25 μM AA), the oxidation peak current of DA was positively proportional to its concentration, while the oxidation peak current of AC and AA did not change. Fig. 10c confirms that no obvious changes took place in the DA (50.0 μM) and AC (20.0 μM) oxidation currents while varying the concentration of AA. The DPVs results show three well-distinguished anodic peaks at potentials of 672, 390, and 168 mV, corresponding to the oxidation of AC, DA and AA, respectively, indicating that the simultaneous determination of AC, DA and AA is possible at the PSNSB/CPE. These results indicate that the oxidation processes of AC, DA and AA at the PSNSB/CPE are independent and therefore that simultaneous measurements of the three analytes are possible without any interference. The voltammetric responses of the PSNSB/CPE toward the simultaneous determination of AC, DA, and AA are listed in Table 2.
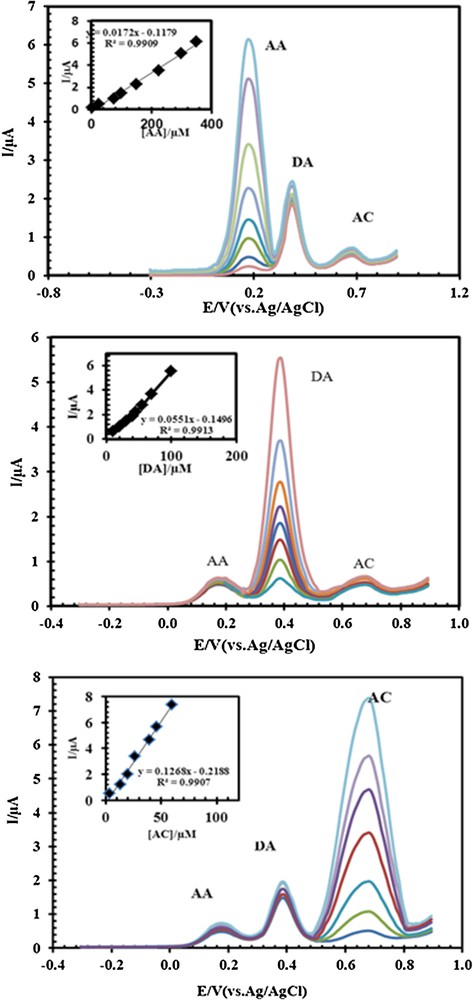
(Color online.) Differential pulse voltammograms of (A) 2.5, 25, 75, 100, 150, 225, 300, and 350 μM AA in the presence of 50 μM DA and 20.0 μM AC; (the insert is plot proportionate with these concentrations). B. 10, 20, 30, 40, 45, 55, 70, and 100 μM DA in the presence of 25 μM AA and 20.0 μM AC (the insert is plot proportionate with these concentrations). C. 3.3, 13.2, 19.8, 26.4, 39.6, 46.2, and 59.4 μM AC in the presence of 25 μM AA and 40 μM DA (the insert is plot proportionate with these concentrations) at pH 4.6 using PSNSBCPE.
Calibration curves parameters for the determination of DA, AA and AC under the optimum conditions at pH 4.6.
Analyte | Regression equation | R2 | RSDa | LODb (M) | LDRc (μM) |
AC | Y = 0.1268x–0.2188 | 0.9907 | 1.9 | 5.36 × 10−9 | 0.033–158.4 |
DA | Y = 0.0551x–0.1496 | 0.9913 | 2.3 | 2.45 × 10−9 | 0.05–120 |
AA | Y = 0.0172x–0.1179 | 0.9909 | 2.1 | 1.86 × 10−8 | 2.5–1050 |
a Relative standard deviation.
b Limit of detection.
c Linear dynamic range.
3.8 Interference study
Possible interference for the detection of AC, DA and AA at the PSNSB/CPE was investigated by the addition of various compounds and ions to a 0.1 M phosphate buffer solution in the presence of 50.0 μmol·L−1 of AC, 20.0 μmol·L−1 of DA, and 100.0 μmol·L−1 of AA. Each possible contaminant was first added to have the same concentration as that of AC, DA and AA and then another addition cause that the concentration of interfering was increased. The tolerance limit was taken as the maximum concentration of the foreign substances, which caused an approximately ± 5% relative error in the determination. The results are given in Table 3.
Interference studied of some foreign substances for 20.0 μM DA, 100.0 μM AA and 50.0 μM AC.
Species | AC | DA | AA |
Glycine | 200 | 1000 | 100 |
Aspirin | 20 | 25 | 30 |
Citric acid | 50 | 30 | 80 |
Urea | 200 | 400 | 150 |
Fructose, sucrose, glucose | 400 | 300 | 300 |
Oxalate | 80 | 30 | 50 |
Aspartic acid | 150 | 250 | 300 |
K+, Ca2+, Mg2+, Na+ | 350 | 1000 | 400 |
Nitrate, carbonate, chloride | 400 | 1000 | 300 |
3.9 Stability, reproducibility and repeatability of the modified electrode
Stability of PSNSB-modified CPE was examined by keeping the electrode in PBS pH 4.6 for 15 days; after this time period, the DPVs were recorded and compared with those obtained before immersion. The results indicated that peak current decreased only slightly for PSNSB-modified CPE, which indicated that PSNSB-modified CPE has good stability. The reproducibility about the preparation procedure of PSNSB/CPE was estimated by the simultaneous determination of 33 μM AC, 20 μM DA and 125 μM AA solution using four independently prepared modified electrodes based on the same fabrication procedure and the obtained relative standard deviation (RSD) values were 1.5%, 1.1% and 1.3%, respectively. These results confirmed good reproducibility of the prepared electrode in both the preparation procedure and voltammetric determinations. The RSD of the peak currents of 33 μM AC, 20 μM DA and 125 μM AA for 10 repeated determinations with the same modified electrode was also 0.35%, 0.14% and 0.28%, respectively. This indicates that the PSNSB/CPE has a good repeatability.
3.10 Determination of AC, DA, and AA in real samples
The utilization of the proposed method in real sample analysis was also investigated by direct analysis of AC, DA and AA in commercial pharmaceutical samples, such as acetaminophen and vitamin C tablets; DA injection solution and AC, DA and AA in human serum sample were tested. The DPVs method was applied to the determination of AC, DA and AA in pharmaceutical preparations and human serum samples. The summarized results for the analysis are given in Tables 4 and 5. According to the results listed in Tables 4 and 5, very good recoveries for the determinations of AC, DA and AA were obtained with high reproducibility, which indicates that the sensor can be applied for the analysis of these compounds with no significant influence from each other.
Determination of DA, AC and AA in pharmaceutical preparations.
Sample | Added (μM) | Found (μM) | Recovery (%) | R.S.D. (%) | ||||||||
DA | AC | AA | DA | AC | AA | DA | AC | AA | DA | AC | AA | |
Tablet of AC | – | – | – | – | 29.9 ± 0.35 | – | – | – | – | – | 1.4 | – |
– | 20 | – | – | 49.7 ± 0.50 | – | – | 99.4 | – | – | 2.1 | – | |
– | 30 | – | – | 60.3 ± 0.70 | – | – | 100.5 | – | – | 1.7 | – | |
DA injection solution | – | – | – | 4.93 ± 0.3 | – | – | – | – | – | 2.4 | – | – |
10 | – | – | 14.62 ± 0.5 | – | – | 97.4 | – | – | 1.1 | – | – | |
Tablet of AA | – | – | – | – | – | 151.1 ± 0.6 | – | – | – | – | – | 1.5 |
– | – | 150 | – | 295.5 ± 0.6 | – | – | 98.5 | – | – | 2.1 | ||
– | – | 250 | – | – | 401.3 ± 0.8 | – | – | 100.3 | – | – | 1.7 |
Determination of DA, AC and AA in human serum samples.
Human serum | Added (μM) | Found (μM) | Recovery (%) | R.S.D. (%) | ||||||||
AC | DA | AA | AC | DA | AA | AC | DA | AA | AC | DA | AA | |
Sample 1 | – | – | – | 10.2 ± 0.4 | 2.02 ± 0.35 | 50.8 ± 0.46 | – | – | – | 1.3 | 1.5 | 1.6 |
Sample 2 | 7 | 10 | 100 | 17.0 ± 0.6 | 12.00 ± 0.40 | 150.0 ± 0.58 | 98.8 | 99.8 | 99.4 | 1.8 | 2.4 | 1.9 |
Sample 3 | 13 | 20 | 150 | 23.1 ± 0.7 | 22.05 ± 0.57 | 200.1 ± 0.73 | 99.5 | 100.1 | 99.6 | 2.4 | 1.8 | 2.3 |
Sample 4 | 20 | 30 | 250 | 29.9 ± 0.8 | 31.90 ± 0.71 | 300.4 ± 0.82 | 99.0 | 99.6 | 99.8 | 1.6 | 1.3 | 2.8 |
3.11 Comparison of proposed method with literature methods
Comparisons of the proposed method with the similar electrochemical methods are presented in Table 6. As the results showed, the proposed method is comparable with the reported methods via linear dynamic range (wider range) and/or limit of detection.
Comparison of the efficiency of some modified electrodes used in the electrocatalysis of AC, DA, and AA.
Modified electrode | Detection limits (M) | Linear ranges (μM) | Reference | ||||
AC | DA | AA | AC | DA | AA | ||
SWCNT/ CCEa | 1.2 × 10−7 | – | 3.0 × 10−6 | 0.2–150 | – | 5–700 | [6] |
2,7–BFEFO/ CPEb | – | – | 4.2 × 10−6 | – | – | 9–3500 | [34] |
T/GCEc | 7.0 × 10−8 | 1.79 × 10−6 | – | 0.2–45.8 | 4–792 | [35] | |
nAu/CPEd | 2.6 × 10−8 | – | – | 0.05–100 | – | – | [24] |
S/GCEe | – | 3.0 × 10−8 | 1.7 × 10−7 | – | 0.05–470 | 0.5–1300 | [36] |
CNT/ CPEf | 2.9 × 10−10 | – | – | 0.07–600 | – | – | [37] |
a Single-walled carbon nanotube-modified carbon ceramic electrode.
b 2,7-bis(ferrocenylethynyl) fluoren-9-one modified carbon paste electrode.
c Tiron modified glassy carbon electrode.
d Gold nanoparticles carbon paste electrode.
e Sulfonazo III film modified on glassy carbon electrode.
f Nanotube-modified carbon paste electrode.
4 Conclusions
The results obtained in this work demonstrated the potentiality of the PSNSBCPE modified electrode for simultaneous determination of AC, DA, and AA. The modified electrode exhibits electrocatalytic activity for the oxidation of AC, DA and AA associated with negative shifts in anodic peak potentials. Thus, large peak separations obtained with this electrode exhibit good selectivity toward simultaneous determination of AC, DA, and AA. The scan rate effect was found to be a diffusion-controlled electrode process. High selectivity, good sensitivity, low detection limits, high stability, and the low cost of the sensor are among the properties of this modified electrode. With these excellent features, the sensor provides a new strategy for the determination of AC, DA and AA in real pharmaceutical samples.
Acknowledgment
The authors wish to express their gratitude to the University of Payame Noor for the financial support of this work.