1 Introduction
A great deal of interest has been directed in recent years towards the metal-controlled template synthesis of macrocyclic species [1–4]. To some extent, the interest in macrocyclic systems stem from the chemical properties that the macrocyclic ligands bring to the complexes. One of these properties is the enhanced thermochemical and kinetic stability of the complexes with regard to their dissociation. Thus the macrocyclic ligand forms more stable complexes as compared to their open-chain analogues [5]. Macrocyclic metal chelating agents like DOTA are useful for detecting tumour lesions [6] as well as they have DNA nuclease activity [7]. Macrocyclic metal complexes of lanthanides, e.g., Gd3+, are used as MRI contrast agents [8]. In order to gain further insight into the above properties of macrocyclic complexes, we report here the synthesis and characterization of a new series of macrocyclic complexes derived from 1,8-diaminonaphthalene and 5,5-dimethylcyclohexane-1,3-dione in the presence of trivalent metal salts.
2 Experimental
2.1 Materials and physical measurements
All chemicals and solvents used in this study were of AnalaR grade; 1,8-diaminonaphthalene and 5,5-dimethylcyclohexane-1,3-dione were purchased from Acros, New Jersey, USA. The metal salts were purchased from S.D.-fine, Mumbai, India, E-Merck, Ranbaxy, India. DPPH was purchased from Sigma Aldrich. These chemicals were used as received. The microanalyses of C, H and N were carried out at SAIF, IIT, Bombay. The metal contents in the complexes were determined by methods taken from the literature [9]. The magnetic susceptibility measurements were made at SAIF, IIT, Roorkee, on a vibrating sample magnetometer (Model PAR 155). The IR spectra were recorded on an infrared spectrometer in the range 4000–200 cm–1 using KBr pellets at SAIF, Punjab University, Chandigarh. Electronic spectra (DMSO) were recorded on a Cary Model 14 spectrophotometer. Mass spectra were recorded at SAIF, Punjab University, Chandigarh. Conductance (DMSO) of complexes was measured on a digital conductivity meter (HPG System, G-3001). The melting points were determined using capillaries in an electrical melting point apparatus.
2.2 Synthesis of the complexes
All complexes were synthesized by the template method, i.e. by condensation of 1,8-diaminonaphthalene and 5,5-dimethylcyclohexane-1,3-dione in the presence of the respective trivalent metal salts. To a hot stirred methanolic solution (∼50 cm3) of 1,8-diaminonaphthalene (10 mmol, 1.582 g) was added trivalent chromium or iron (5.0 mmol) dissolved in the minimum quantity of methanol (∼20 cm3). The resulting solution was refluxed for 0.5 h. Subsequently, 5,5-dimethylcyclohexane-1,3-dione (10 mmol, 1.37 g) dissolved in methanol was added to the refluxing mixture and refluxing was continued for 8 to 10 h. The mixture was cooled to room temperature whereby dark-coloured precipitates formed, which were filtered, washed with methanol, acetone and diethyl ether and dried in vacuo. The obtained yields were ∼45–55%. The complexes were soluble in DMSO and DMF whereas insoluble in most of the solvents. They decompose above 300 °C without melting.
2.2.1 Analyses of the metal content
In all cases, the organic part of the complexes was completely eliminated before the estimation of metal ions from the complexes. The following general procedure was adopted for this purpose for all the metal complexes. A known amount (∼0.1 g) of the metal complexes was decomposed with concentrated nitric acid at high temperature, the excess acid being expelled by evaporation with concentrated hydrochloric acid. This process was repeated till the organic part of the complex was completely removed. The residue was cooled and this residue was dissolved in distilled water in both cases.
2.2.1.1 Fe(III) determination
Standard EDTA was added to the above-obtained solution and then hexamine was added to adjust the pH to 5–6. Now xylenol orange was added as an indicator. Excess EDTA was titrated with standard lead nitrate till the end point was reached, i.e. when a red–violet colour appeared.
2.2.1.2 Cr(III) determination
NaOH was added to the solution obtained after the decomposition of the organic part for neutralization purpose until precipitates began to form. Now an acetate buffer (6 M CH3COOH + 0.6 M CH3COONa) was added. After the addition of a mixture of lead nitrate and of potassium bromate, the reaction medium was heated up to 90–95 °C till precipitation was completed. The lead chromate precipitate was cooled, filtered on a sintered glass crucible and weighed.
The condensation of 1,8-diaminonaphthalene and 5,5-dimethylcyclohexane-1,3-dione in the presence of trivalent metal salts, in the molar ratio 2:2:1, is represented in Scheme 1.
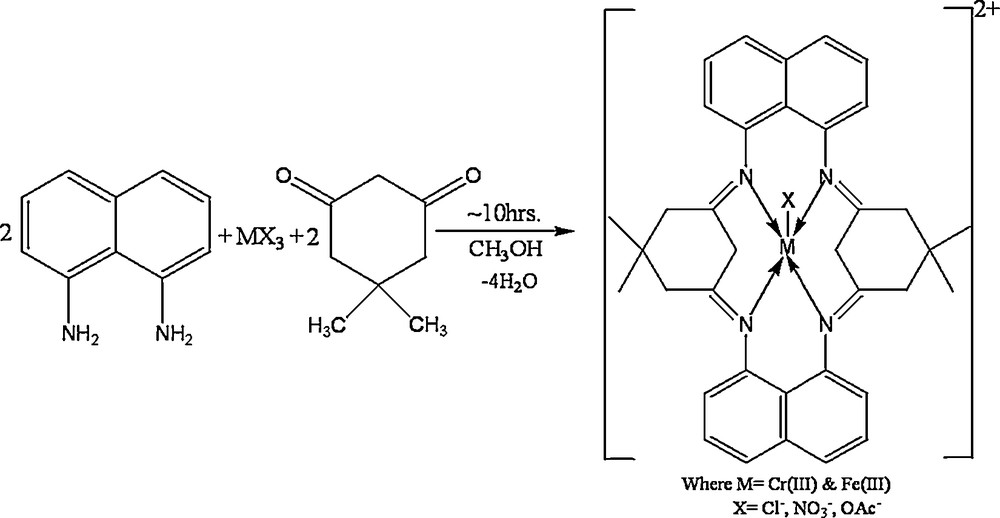
Synthesis of complexes derived from 1,8-diaminonaphthalene and 5,5-dimethylcyclohexane-1,3-dione with trivalent chromium and iron metal salts.
2.3 Molecular modelling
The ligand–M(III) complexes [M = Cr(III), Fe(III)] were modelled by Gaussian 09W program using B3LYP functional with 6-31G(d, p) basis sets. The metal-ion-containing ligands were optimized using the DFT method. Several cycles of energy minimization had to be carried out for each of the molecules.
2.4 Pharmacology
Six microbial strains (four bacterial and two fungal) were selected on the basis of their clinical importance in causing disease in humans. Staphylococcus aureus (MTCC 96), Bacillus subtilis (MTCC 121) (Gram-positive bacteria); Escherichia coli (MTCC 1652), Pseudomonas aeruginosa (MTCC 741) (Gram-negative bacteria); Candida albicans (MTCC 3017), Saccharomyces cerevisiae (MTCC 170) (yeast strains) were screened for evaluation of antibacterial and antifungal activities of synthesized complexes. All the microbial cultures were procured from Microbial Type Culture Collection (MTCC), IMTECH, Chandigarh. The bacteria were subcultured on nutrient agar as well as yeast on malt yeast agar.
2.4.1 Primary screening
The antimicrobial activities of newly synthesized complexes were evaluated by the agar well diffusion method reported in the literature [10]. DMSO was used as a negative control, whereas Ciprofloxacin was used as a positive control for bacteria and amphotericin-B for yeast. This procedure was performed in three replicate plates for each organism.
2.4.2 Determination of the minimum inhibitory concentration (MIC)
MIC is the lowest concentration of an antimicrobial compound that will inhibit the visible growth of a microorganism after overnight incubation. MIC of the synthesized complexes against bacteria and yeast strains was tested through a modified agar well diffusion method [10]. Ciprofloxacin and amphotericin-B were used as positive controls, and DMSO as ab negative control.
2.4.3 Antioxidant activity analysis
The antioxidant activity of all the complexes was tested by DPPH radical scavenging method [11]. Briefly 1 ml of each complex solution, prepared in DMSO, was added to 3 ml of a methanolic solution of DPPH (0.1 mM). After 30 min, the absorbance of the complexes was taken at 517 nm. Ascorbic acid was used as a positive control whereas the DPPH solution was used as a negative control. The percentage of scavenging activity of DPPH free radicals was measured by using the following formula:
3 Results and discussion
3.1 Chemistry
The analytical data of the metal complexes, given in Table 1, were found to be consistent with the molecular formula [M(C36H36N4)X]X2, where M = Cr(III), Fe(III) and X = Cl–1, NO3–1, and CH3COO–1. The test for anions was positive before as well as after the decomposition of the complexes. Conductivity measured in DMSO indicates that they are 1:2 electrolytes (150–180 Ω–1·cm2·mol–1) [12]. The spectroscopic and magnetic data indicated that the complexes have a square pyramidal geometry (Table 1).
Analytical data of the synthesized Cr(III) and Fe(III) complexes derived from 1,8-diaminonaphthalene and 5,5-dimethylcyclohexane-1,3-dione.
Sr. No. | Complexes | Colour | ^M | %M Found (Calcd) | %C Found (Calcd) | %H Found (Calcd) | %N Found (Calcd) | μeff. B.M. |
1 | [Cr(C36H36N4)Cl]Cl2 | Black | 174 | 7.60 (7.62) | 62.99 (63.25) | 5.24 (5.27) | 8.14 (8.20) | 4.30 |
2 | [Cr(C36H36N4)(NO3)](NO3)2 | Black | 159 | 6.79 (6.82) | 55.26 (56.69) | 4.28 (4.76) | 12.78 (12.86) | 4.21 |
3 | [Cr(C36H36N4)(OAc)](OAc)2 | Black | 165 | 6.89 (6.89) | 66.90 (66.92) | 5.89 (6.02) | 7.45 (7.43) | 4.50 |
4 | [Fe(C36H36N4)Cl]Cl2 | Black | 155 | 8.04 (8.13) | 62.92 (62.93) | 4.95 (5.28) | 8.10 (8.15) | 5.70 |
5 | [Fe(C36H36N4)(NO3)](NO3)2 | Black | 168 | 7.16 (7.29) | 56.32 (56.41) | 4.51 (4.73) | 11.93 (12.79) | 5.65 |
6 | [Fe(C36H36N4)(OAc)](OAc)2 | Dark brown | 160 | 7.32 (7.37) | 66.50 (66.58) | 5.96 (5.99) | 7.40 (7.39) | 5.90 |
3.2 IR spectra
The IR spectrum of 1,8-diaminonaphthalene shows a pair of medium-intensity bands at ∼3302 and 3387 cm–1, corresponding to the v(NH2) group, which were found to be absent in the IR spectra of all the metal complexes. Furthermore, no strong absorption band was observed near 1710 cm–1, indicating the absence of >C = O groups of 5,5-dimethylcyclohexane-1,3-dione moiety and thus confirms the condensation of the amino group of diaminonaphthalene and the carbonyl group of 5,5-dimethylcyclohexane-1,3-dione. A strong absorption band at 1595–1640 cm–1 confirms the formation of the macrocyclic frame [13] (Fig. S1). The lower value of v(C = N) may be explained by a drift of the lone-pair electron density of the azomethine nitrogen towards the metal atom [14]. The medium intensity bands in the region 2830–2990 cm–1 may be assigned to v(C–H) stretching vibrations of the methyl groups in the 5,5-dimethylcyclohexane-1,3-dione moiety. The v(C–N) stretching vibrations occur at 1010–1250 cm–1. The various absorption bands in the region between 1400 and 1588 cm–1 may be assigned to aromatic skeletal vibrations of the naphthalene ring and the bands around 845–875 cm–1 may be attributed to the C–H out-of-plane bending vibration of the naphthalene moiety [15,16] (Supplementary material, Fig. S1(a and d)).
Nitrate complexes: the presence of absorption bands around 1412–1427, 1281–1296 and 1080–1095 cm–1 in the spectrum of nitrato complexes suggests the unidentate coordination of nitrogen with the central metal ion [17] (Supplementary material, Fig. S1(b and e)).
Acetate complexes: the IR spectra of acetate complexes includes absorption bands at 1674–1682 and at 1296–1319 cm–1, which are assigned to the v(COO–)as asymmetric and v(COO–)s symmetric stretching vibrations of the acetate ion, respectively. The difference (vas–vs), which is around 363–376 cm–1, is greater than 144 cm–1, indicating an unidentate coordination behaviour of acetate ion [18] (Supplementary material, Fig. S1(c and f)).
The far-infrared spectra show bands in the region 420–450 cm–1, corresponding to v(M–N) vibrations [19–21]. The presence of bands in all complexes in the region 420–450 cm–1, originating from (M–N) azomethine vibrational modes, identifies the coordination of the azomethine nitrogen [22]. The bands present in the range 300–320 cm–1 may be assigned to v(M–Cl) vibrations [19–21]. The bands present in the region 220–250 in all nitrato complexes are related to v(M–O) stretching vibrations [19,20].
3.3 Mass spectra
The EI mass spectra of Cr(III) and Fe(III) macrocyclic complexes exhibit parent peaks due to molecular ions [M]+ and [M + 2]+. The proposed molecular formulas of these complexes were confirmed by comparing their molecular formula weights with m/z values. The molecular ion [M]+ peaks obtained for these complexes are as follows: [Cr(C36H36N4)Cl]Cl2 (1), m/z = 681.5 (due to 35Cl) and 683.5 (due to 37Cl) [Mol. Wt. 683]; [Cr (C36H36N4) NO3] (NO3) (2), m/z = 761 [Mol. Wt. 762]; [Cr(C36H36N4)OAc] (OAc)2 (3), m/z = 752 [Mol. Wt. 753]; [Fe(C36H36N4)Cl]Cl2 (4), m/z = 683.3 (due to 35Cl) and 685.2 (due to 37Cl) [Mol. Wt. 686]; [Fe (C36H36N4) NO3] (NO3)2 (5), m/z = 764.8 [Mol Wt. 766]; [Fe(C36H36N4)OAc] (OAc)2 (6), m/z = 756.5 [Mol. Wt. 757]. The data were in good agreement with the proposed formula. This indicates the formation of macrocyclic frames. In addition to the molecular ion peaks, the spectra also contain the peaks of various fragments resulting from the thermal cleavage of the complexes (Fig. 1 and Supplementary material, Fig. S2).
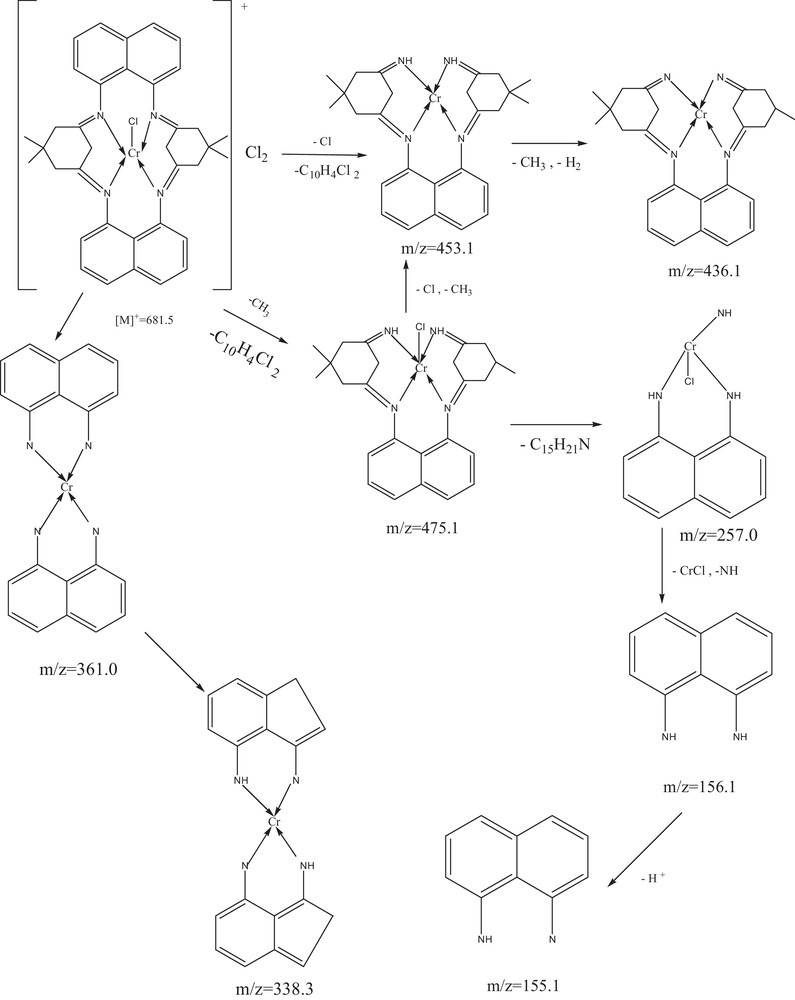
Mass spectral fragmentation of [Cr(C36H36N4)Cl](Cl)2.
3.4 Electronic spectral analyses
3.4.1 Chromium complexes
The magnetic moment of chromium(III) complexes at room temperature was found to be in the range 4.21–4.50 B.M., which is close to the predicted values for three unpaired electrons in the metal ion [23]. The electronic spectra of the chromium complexes show bands at 1048–1082, 744–785, 545–593, 360–370 and a shoulder at 285 nm. These spectral bands are consistent with a five-coordinated square pyramidal geometry of chromium complexes, the structure of which was confirmed by X-ray measurements [24]. Thus, assuming a C4v symmetry for these complexes [25,26], the various bands may be assigned as: 4B1→4Ea, 4B1→4B2, 4B1→4A2, and 4B1→4Eb, respectively (Fig. 2 and Supplementary material, Fig. S3(a and b)).
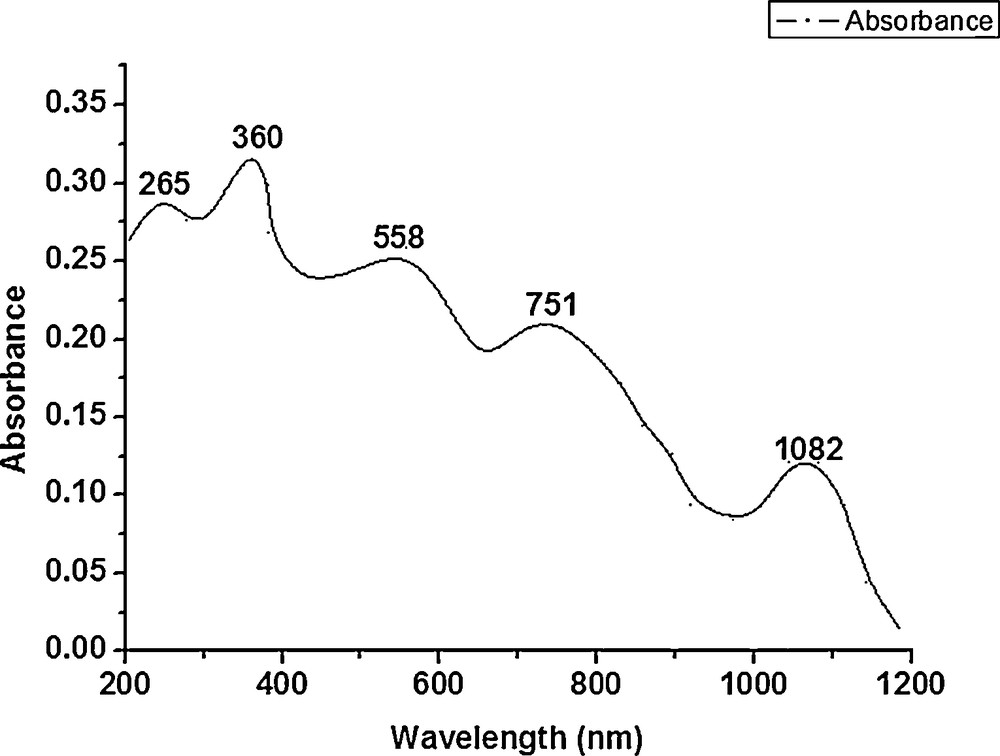
Electronic spectra of [Cr(C36H36N4)Cl]Cl2.
3.4.2 Iron complexes
The magnetic moment of iron complexes lies in the range 5.65–5.90 B.M., corresponding to five unpaired electrons, which is close to the predicted high spin values for these metal ions [23]. The electronic spectra of the iron complexes show bands at 1002–1013, 643–651 and 356–362 nm, which are consistent with the reported square pyramidal geometry for iron(III) complexes [25,26]. Assuming a C4v symmetry for these complexes, the various bands can be assigned as: dxy→dxz, dyz and dxy →dz2 (Supplementary material, Fig. S3(c–e)).
3.5 Molecular modelling
The calculated optimized energy for complex [Cr(C36H36N4)Cl]Cl2, shown in Fig. 3a, was 3115.25 a.u., whereas the calculated energy of HOMO and LUMO is 0.217 a.u. and 0.172 a.u., respectively. The selected bond lengths and bond angles are as listed in Table 2. The HOMO and LUMO orbitals of the complexes are shown in the Supplementary material, Fig. S4.
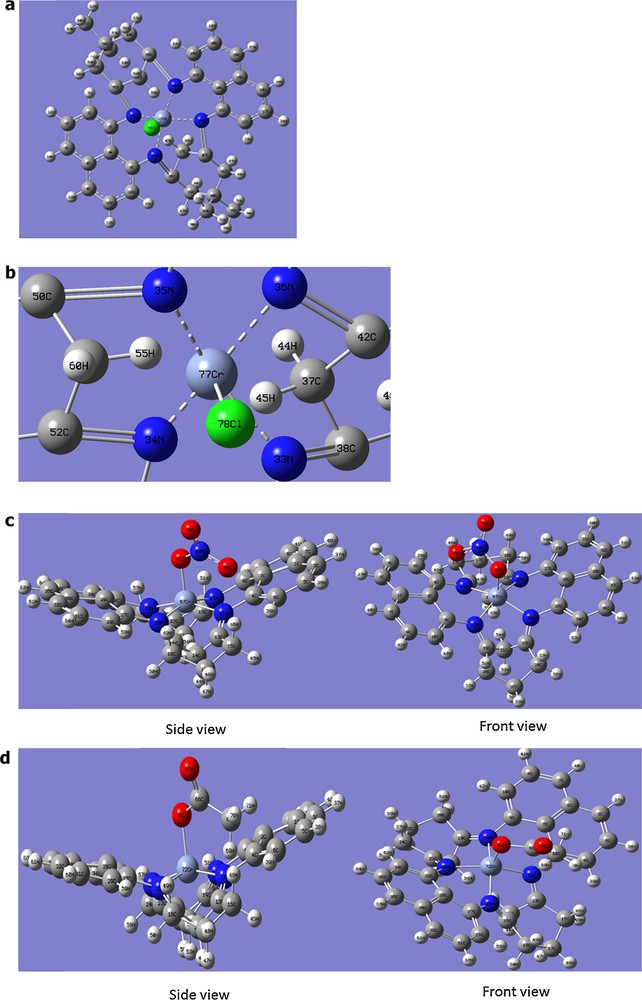
(Colour online.) a: geometry-optimized structure of complex [Cr(C36H36N4)Cl]2+; b: enlarged view of Fig. 3a; c: geometry-optimized structure of complex [Cr(C36H36N4)(NO3)]2+; d: geometry-optimized structure of complex [Cr(C36H36N4)(OAc)]2+.
Selected bond lengths and bond angles of complex [Cr(C36H36N4)Cl]2+.
Parameters | Bond length (Å) | Parameters | Bond angles (degree) |
33N–77Cr | 2.05 | Cl 78–Cr 77–N33 | 72.041 |
34N–77Cr | 1.61 | Cl 78–Cr 77–N34 | 66.871 |
35N–77Cr | 2.06 | Cl 78–Cr 77–N35 | 84.857 |
36N–77Cr | 2.07 | Cl 78–Cr 77–N36 | 103.251 |
77Cr–78Cl | 2.12 | N33–Cr77–N34 | 83.258 |
50C–35N | 2.23 | N34–Cr77–N35 | 99.975 |
52C–34N | 1.85 | N35–Cr77–N36 | 72.643 |
42C–36N | 1.92 | N36–Cr77–N33 | 99.460 |
38C–33N | 2.24 |
3.6 Powder XRD
The X-ray diffractogram of metal complex [Cr (C36H36N4)(NO3)](NO3)2 was scanned in the range 4–85 degree at wavelength 1.54060 Å; the generator settings were 30 mA, 40 kV. The interplanar spacing (d value), hkl and lattice parameters were calculated using computer programme FullProf suite [27]. The indexing was confirmed by comparing the observed and calculated 2θ values, as shown in Table 3. The lattice parameters for the complex were a = 4.6549, b = 8.2856, c = 5.0549, β = 90.626, α = γ = 90 and volume = 194.95. The condition a ≠ b ≠ c and α = γ ≠ β for Cr(III) complexes are consistent with a monoclinic crystal system. (Supplementary material, Fig. S5).
X-ray diffraction data of complex [Cr(C36H36N4)(NO3)](NO3)2.
2 θ Obs | 2 θ Cal | d spacing | h | k | l | Diff. 2θ |
17.524 | 17.515 | 5.05684 | 1 | 0 | 0 | 0.009 |
28.805 | 28.810 | 3.09686 | 0 | 2 | 1 | –0.005 |
40.376 | 40.375 | 2.23208 | 2 | 0 | –1 | 0.002 |
43.992 | 43.981 | 2.05663 | 1 | 1 | –2 | 0.012 |
53.142 | 53.137 | 1.72209 | 0 | 2 | 0 | 0.005 |
55.561 | 55.574 | 1.65270 | 2 | 0 | –1 | –0.013 |
60.622 | 60.630 | 1.52627 | 1 | 2 | 0 | –0.008 |
60.976 | 60.947 | 1.51825 | 1 | 0 | –2 | 0.029 |
62.088 | 62.104 | 1.49372 | 2 | 1 | –1 | –0.016 |
62.972 | 62.948 | 1.47484 | 2 | 1 | 0 | 0.024 |
63.489 | 63.512 | 1.46407 | 0 | 0 | 2 | –0.022 |
3.7 Pharmacological results
3.7.1 Antimicrobial activity
All complexes were screened for their antibacterial and antifungal activity. All the six tested complexes possessed variable antibacterial activity against the Gram-positive (S. aureus and B. subtilis) bacteria, while only three complexes, 1, 5 and 6, displayed activity against Gram-negative bacteria (E. coli), whereas all complexes do not exhibit any activity against P. aeruginosa. Among all complexes, only complex 1 exhibits an activity against C. albicans and S. cerevisiae, with zones of inhibition of 16.3 mm and 18.6 mm, respectively (Fig. 4).
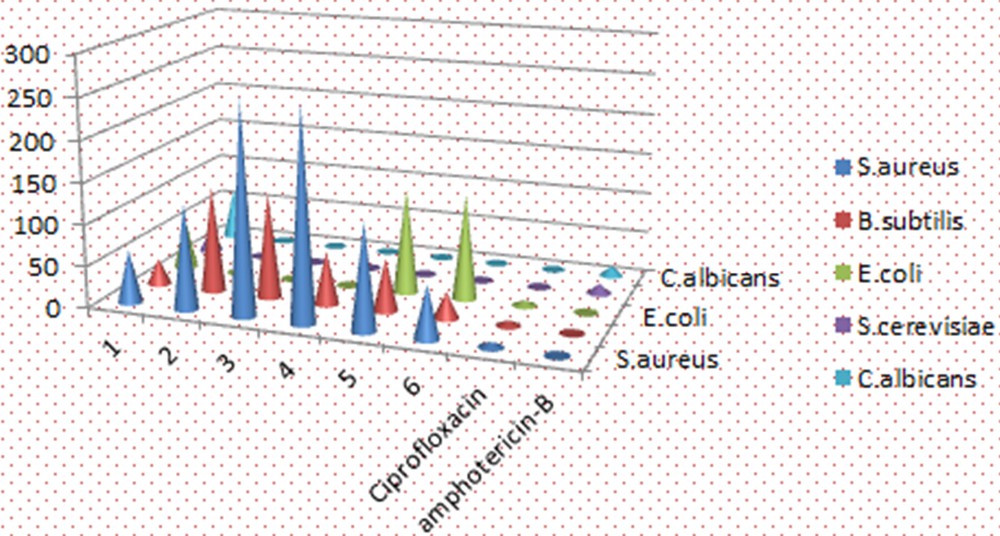
(Colour online.) Bar graph representation of the minimum inhibitory concentration (MIC) of the complexes.
On the basis of the maximum inhibitory activity shown against the tested bacteria, complex 1 was found to be the most effective against B. subtilis, S. aureus, and E. coli, with zones of inhibition of 23.6 mm, 20.6 mm, and 18.6 mm, respectively (Table 4).
In vitro antibacterial activities of synthesized trivalent chromium and iron macrocyclic complexes derived from 5,5-dimethylcyclohexan-1,3-dione and 1,8-diaminonaphthalene using the agar well diffusion method.
Compound No. | Diameter of growth of inhibition zone (mm)a | |||||
S. aureus | B. subtilis | E. coli | P. aeruginosa | S. cerevisiae | C. albicans | |
1 | 20.6 | 23.6 | 18.6 | – | 18.6 | 16.3 |
2 | 15.3 | 17.3 | – | – | – | – |
3 | 13.6 | 15.6 | – | – | – | – |
4 | 14.6 | 18.3 | – | – | – | – |
5 | 15.3 | 20.6 | 16.3 | – | – | – |
6 | 18.3 | 22.6 | 15.6 | – | – | – |
Ciprofloxacin | 26.6 | 24.0 | 25.0 | 22.0 | Nt | Nt |
Amphotericin-B | Nt | Nt | Nt | Nt | 19.3 | 16.6 |
a Values, including the diameter of the well (8 mm), are means of three replicates.
In the whole series, the MIC of the various tested chemical compounds ranged between 32 μg/ml and 256 μg/ml against Gram-positive bacteria. Complexes 1 and 6 were found to be best as they exhibit the lowest MIC of 32 μg/ml against B. subtilis and 64 μg/ml against S. aureus. However, in case of yeast, complex 1 was found to be the best as they exhibit the lowest MIC of 64 μg/ml against C. albicans and 32 μg/ml against S. cerevisiae (Table 5).
Minimum inhibitory concentration (MIC) (in μg/ml) of chemical compounds by using the modified agar well diffusion method.
Compound No. | Staphylococcus aureus | Bacillus subtilis | Escherichia coli | Saccharomyces cerevisiae | Candida albicans |
1 | 64 | 32 | 64 | 32 | 64 |
2 | 128 | 128 | nt | nt | nt |
3 | 256 | 128 | nt | nt | nt |
4 | 256 | 64 | nt | nt | nt |
5 | 128 | 64 | 128 | nt | nt |
6 | 64 | 32 | 128 | nt | nt |
Ciprofloxacin | 6.25 | 6.25 | 6.25 | nt | nt |
Amphotericin-B | nt | nt | nt | 12.5 | 12.5 |
Among all the tested chemical complexes, complex 1, i.e. [Cr (C36H36N4)Cl]Cl2, was found to be the best one in inhibiting the growth of bacteria and fungi.
3.7.2 Antioxidant activity
The newly synthesized complexes were tested for their antioxidant activity by free-radical scavenging using DPPH. The IC50 value, i.e. the concentration of the complexes required to reduce the initial absorption by 50%, was determined as shown in Fig. 5. It was found that complex 4, i.e. [Fe(C36H36N4)Cl]Cl2 has the highest IC50 value, i.e. the lowest antioxidant activity, whereas complex 1, i.e. [Cr(C36H36N4) Cl]Cl2 has the lowest IC50 value and the highest antioxidant activity, as shown in Table 6.
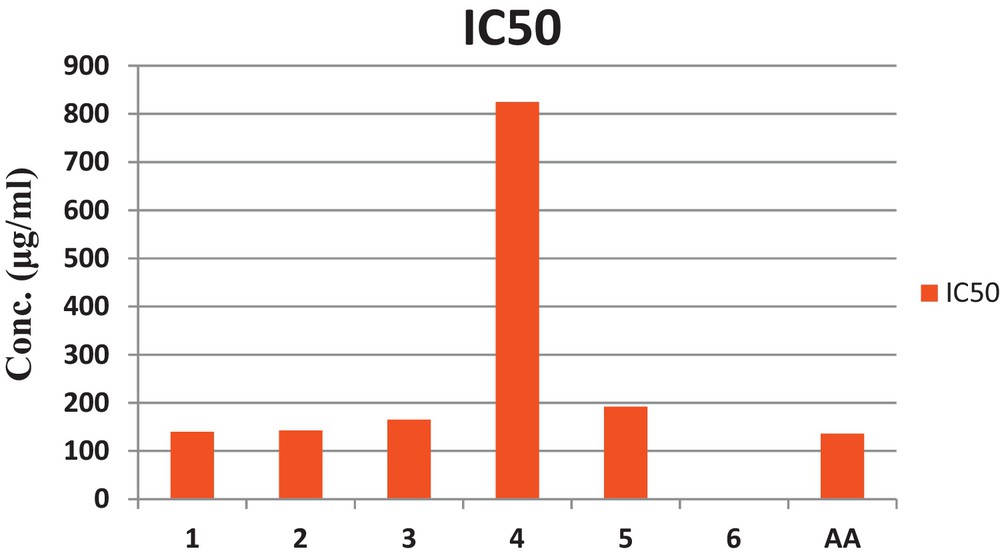
Bar graph representation of the antioxidant activity (IC50) of the complexes.
IC50 values of the test compounds (μg/ml).
Complex | AA | 1 | 2 | 3 | 4 | 5 | 6 |
IC50 (μg/ml) | 136.0 | 140 | 143 | 165 | 825 | 192 | 1000 |
4 Conclusions
Based on various studies like elemental analyses, conductance measurements, magnetic susceptibilities, infrared, electronic, mass and X-ray diffraction studies, a square pyramidal geometry may be proposed for all of these complexes. Powder XRD suggests that the complexes belong to the monoclinic crystal system. Complex 1 shows the best antimicrobial as well as the best antioxidant activity. Therefore this compound can be further explored in the pharmaceutical industry, after testing its toxicity to human beings. Chelation/coordination reduces the polarity of the metal ion mainly because of partial sharing of its positive charge with the donor group within the whole chelate ring system [28,29], which enhances the lipophilic nature of the central metal atom, which in turn favours its permeation through the lipoid layer of the membrane, thus causing the metal complex to cross the bacterial membrane more effectively, thus increasing the activity of the complexes.
Acknowledgements
Authors are thankful to the Department of Microbiology, Kurukshetra University, Kurukshetra for carrying out the in vitro antimicrobial analysis. One of the authors, Ms. Parveen, thanks CSIR, New Delhi, India for financial support in the form of a Senior Research Fellowship (File No. 09/1050 (0001)/2011-EMR-1).