1 Introduction
Multicomponent reactions (MCRs) can be defined as convergent chemical processes where three or more reagents are combined in such a way that the final product retains significant portions of all starting materials. From an environmental perspective, it is obvious that the traditional methods of performing chemical synthesis are unsustainable, and multicomponent reactions provide a great solution since they are more efficient, cost-effective and less wasteful [1].
The 1,4-dihydropyridine (DHP) scaffold is an important pharmacophore found in a large number of biologically active and potential therapeutic compounds [2]. They present significant biological activity, for example, as calcium channel blockers, multidrug-resistance (MDR) reversing agents, neuroprotective agents, and chemosensitizers. Furthermore, the dihydropyridine skeleton is common in many bronchodilator, antitumor, antidiabetic, and hepatoprotective agents [3].
The Hantzsch synthesis is one of the most broadly used methods for the preparation of DHPs. The classical methods for the production of 1,4-dihydropyridines involve the three-component condensation of an aldehyde with a β-dicarbonyl compound, and a source of ammonia or an amine in acetic acid or in refluxing alcohol [4]. Several procedures using molecular iodine, cyanuric chloride, ionic liquids, silica gel/NaHSO4, TMSCl–Nal, metal triflates, and heteropolyacids have been reported for this synthesis, with excellent yields [5].
A recent paper describes that the asymmetric 2-arylpyridines could be obtained as a major product in the Hantzsch reaction, by the one-pot multicomponent reaction of benzaldehyde, ethyl acetoacetate and ammonium under solvent-free, heat-free, catalysis-free and oxidant-free conditions, with a long reaction time (72 h). In these conditions, only trace amounts of classic Hantzsch products were obtained [6]. Two recent reports show that 2-arylpyridines can also be obtained via the Hantzsch reaction: under visible irradiation and oxygen atmosphere using [Ru(bpy)3]+2 as a catalyst [7] or by heating using Si–Zr–Mo nanocomposites [8]. The most commonly used method for the synthesis of 2-arylpyridines is the metal-catalyzed cross-coupling reaction of aryl or pyridyl halides; however, one has to introduce functional groups after the cross-coupling because of the low compatibility of the organometallic compounds with many substituents [7,9].
On the other hand, in the last decade, organocatalysts have emerged as a powerful method for chemical transformation. They have attracted increasing interest due to their operational simplicity, low toxicity and cost, and tolerance to traces of water and molecular oxygen. In addition, products from reactions catalyzed by non-metallic species are desirable to pharmaceutical, food, and cosmetic industries [10].
Calix[n]arenes have been used as catalysts in organic synthesis, for example, the use of sulfonic calix[n]arenes in the allylic alkylation reaction [11], Mannich-type reactions [12], Biginelli reaction [10a], and esterification of fatty acid, such as palmitic acid [13]. In this letter, we report a new methodology for the synthesis of unsymmetrical 2-arylpyridines via aromatization of 1,2-dihydropyridines produced in the Hantzsch reaction using p-sulfonic acid calix[6]arene as an efficient and reusable organocatalyst (Scheme 1).
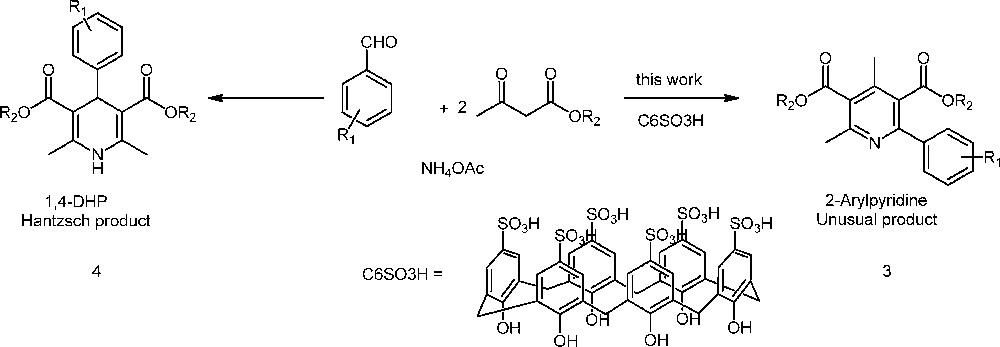
1,4-DHP and 2-arylpyridine formation.
2 Experimental
2.1 Materials and methods
All chemicals were purchased commercially and used without further purification. Melting points were measured in an open capillary using a Büchi melting point apparatus and were uncorrected. 1H NMR and 13C NMR spectra were recorded on a Bruker AM-500 spectrometer (500 and 125 MHz, respectively) using TMs as an internal standard. Gas chromatography (GC) was recorded on a Shimadzu 2010 instrument. p-Sulfonic acid calix[6]arene was synthesized in our laboratory following the literature procedures. First p-tert-butyl-calix[6]arene was prepared by the Gutsche and Iqbal method [14], secondly p-tert-butyl-calix[6]arene was dealkylated by treatment with aluminum chloride in the presence of toluene and phenol according to the method described by Ungaro et al. [15]; finally, the preparation of p-sulfonic acid calix[6]arene was carried out with the treatment of calix[6]arene with concentrated sulfuric acid (98 wt %) added (Shinkai et al.) (purity > 99%, elemental analysis) [16].
2.2 General procedure for the synthesis of 2-substituted pyridines
A 10-mL reaction tube was charged with aldehyde (1 mmol), methyl or ethyl acetoacetate (2 mmol), ammonium acetate (1.2 mmol) and the catalyst (20 mg, ca. 1.8 mmol %). The mixture was stirred at 25 °C until mixing was completed (monitored by TCL). It was extracted with ethyl acetate (2 × 3 mL) and dried over anhydrous Na2SO4, filtered and concentrated under reduced pressure to leave the crude product, which was purified by chromatography over silica gel. All products were purified by recrystallization using methanol or ethanol as a solvent.
2.3 1H and 13C NMR data for selected compounds
2.3.1 Dimethyl 2-phenyl-4,6-dimethylpyridine-3,5-dicarboxylate (3a)
Yield 81%; white solid; mp: 84–85 °C; 1H NMR = (500 MHz, CDCl3): 7.57–7.54 (2H, m); 7.40–7.38 (3H, m), 3.93 (3H, s), 3.59 (3H, s), 2.58 (3H, s), 2.32 (3H, s); 13C NMR = (125 MHz, CDCl3): 168.8, 168.7, 156.3, 155.4, 143.2, 139.5, 130.2, 129.2, 128.5, 128.1, 127.1, 52.5, 52.4, 23.2, 17.2.
2.3.2 Dimethyl 2-(4-chlorophenyl)-4,6-dimethylpyridine-3,5-dicarboxylate (3e)
Yield 92%; white solid; mp: 88–90 °C; 1H NMR = (500 MHz, CDCl3): 7.50 (2H, d, J = 8 Hz), 7.38 (2H, d, J = 8 Hz), 3.92 (3H, s), 3.62 (3H, s), 2.58 (3H, s), 2.31 (3H, s); 13C NMR = (125 MHz, CDCl3): 168.5, 168.4, 155.5, 154.8, 143.1, 137.8, 135.2, 129.4, 128.4, 128.2, 126.7, 52.4, 52.3, 23.2, 23.0.
2.3.3 Diethyl 2-(4-methylphenyl)-4,6-dimethylpyridine-3,5-dicarboxylate (3k)
Yield 71%; white solid; mp: 49–50 °C; 13H NMR = (500 MHz, CDCl3): 7.48–7.50 (2H, m), 7.22 (1H, s), 7.20 (1H, s), 4.45 (2H, q; J = 7.1 Hz), 4.13 (2H, q, J = 7.1 Hz), 2.1 (3H, s), 2.40 (3H, s), 1.44 (3H, t, J = 7.1 Hz), 1.05 (3H, t, J = 7.1 Hz); 13C NMR = (125 MHz, CDCl3): 168.5, 168.4, 156.3, 155.1, 142.5, 138.9, 137.0, 129.1, 128.2, 128.0, 127.2, 61.8, 61.5, 23.2, 21.4.
3 Result and discussion
First, we prepared the calix[6]arene catalyst, and then, we studied its catalytic activity in the mentioned reaction. In general, and as reported by Ananthakrishnan et al., the Hantzsch reaction produces 1,4-dihydropyridine as the principal product in the presence of the catalyst, solvent and heating. This is because the reaction proceeds via 1,4-addition (Michael-type), which is the thermodynamically favourable compound [7]. Meanwhile, 2-arylpyridine can be obtained as the principal product at 20 °C and in an open atmosphere, under solvent- and catalysis-free conditions, in a long reaction time (72 h). In this case, the reaction proceeds through a 1,2-adition (kinetic control) [11].
To obtain the optimized reaction condition for 2-arylpyridine synthesis, we performed several experiments based on the variation of temperature. The reaction conditions were examined employing benzaldehyde (1 mmol), methyl acetoacetate (2 mmol) and ammonium acetate (1.2 mmol) as substrates. Table 1 shows the results obtained in the respective reactions under solvent-free conditions at different temperatures with or without catalyst. Low-temperature reaction conditions were favourable for forming 1,2 DHP (see Table 1, entry 1, reaction performed at 110 °C, ratio of 3a/4a: 30:70 vs. entry 9 reaction performed at 25 °C, ratio of 3a/4a: 83:17).
Effect of the reaction temperature on the conversion and selectivity of products 3 and 4 under solvent-free conditions with and without catalyst.
Entry | Catalyst | Temperature (° C) | Time (h) | Conversion (%) | Selectivity 3:4 (%) |
1 | Yes | 110 | 0.5 | 100 | 30:70 |
2 | None | 8 | 80 | 38:62 | |
3 | Yes | 80 | 2 | 100 | 40:60 |
4 | None | 12 | 72 | 30:70 | |
5 | Yes | 60 | 3 | 100 | 51:49 |
6 | None | 16 | 75 | 68:32 | |
7 | Yes | 40 | 9 | 100 | 73:27 |
8 | None | 30 | 70 | 77:23 | |
9 | Yes | 25 | 12 | 100 | 83:17 |
10 | None | 60 | 85 | 87:13 |
When a mixture of three substrates and the catalyst was stirred at 25 °C under solvent-free conditions, the corresponding 2-arylpyridine was formed with 83% yield (100% conversion), in a reaction time of 12 h. Under the same reaction conditions and in the absence of the catalyst, the corresponding 2-arylpyridine was obtained with 87% yield (85% conversion) in 60 h, indicating that the catalyst is effective enough to activate the process (see Table 1: entries 9 and 10, respectively).
The effect on the amount of catalyst on the yield of 2-arylpyridine was checked. The experimental reaction conditions were: benzaldehyde (1 mmol), methyl acetoacetate (2 mmol), ammonium acetate (1.2 mmol) and a variable amount of the catalyst (0.1, 0.9, 1.8, and 5 mmol%). The mixture was stirred at 25 °C for 12 h. The yields increased from 55% to 81% when the amount of catalyst increased from 0.1 to 1.8 mmol%. No relevant changes were observed with further increase in the amount of catalyst (5 mmol%), 80%. Thus 1.8 mmol% of catalyst is a suitable amount of catalyst in this reaction.
Two additional experiments were performed using p-toluene sulfonic acid and hydroxybenzenesulfonic acid. The reaction conditions were: benzaldehyde (1 mmol), methyl acetoacetate (2 mmol), ammonium acetate (1.2 mmol) and the catalyst (1.8 mmol %). The mixture was stirred at 25 °C, for 12 h. In both cases, the principal reaction product was 1,4-dihydropyridine 4.
Encouraged by the remarkable results obtained with the above reaction and in order to show the generality and scope of this catalytic method, the new one-pot three-component protocol was then explored. A series of diverse aldehydes, methyl (ethyl) acetoacetate and ammonium acetate were subjected to solvent-free conditions in an air atmosphere at 25 °C to obtain the corresponding 2-arylpyridine derivatives (Table 2). In most of the studied examples, the reaction proceeded smoothly, and benzaldehyde having either weak electron-withdrawing or electron-releasing substituents reacted efficiently to give very good to excellent yields of the desired 2-arylpyridines.
Synthesis de 2-arylpyridines 3a–3n using C6SO3H as catalysta.
Compound | Product | Yields (%)b | Compound | Product | Yields (%)b |
3a | 81 | 3h | 91 | ||
3b | 77 | 3i | 83 | ||
3c | 82 | 3j | 71 | ||
3d | 62 | 3k | 79 | ||
3e | 92 | 3l | 89 | ||
3f | 89 | 3m | 84 | ||
3g | 88 |
a Reaction conditions: aldehyde (1 mmol); ammonium acetate (1.2 mmol); methyl or ethyl acetoacetate (2 mmol); catalyst (1.8 mmol %); reaction time 12 h; temperature 25 °C; solvent-free reaction; in air atmosphere; under stirring. The experiment was performed in duplicate.
b Yields were based on GC analysis.
All products listed in Table 2 were characterized by 1H NMR and 13C NMR, the corresponding melting points were determined, and the degree of purity > 99% was determined by gas chromatography.
We also investigated the reuse of the catalyst. For this purpose, after completion of the reaction, ethyl acetate (2 × 2 mL) was added to the semi-solid reaction mixture. The catalyst was washed with H2O to remove the ammonium acetate traces (2 × 1 mL), dried under vacuum (25 °C) and reused in these conditions for a new reaction cycle. The yields of 2-arylpyridine 3a for the first, second, and third reuses were 80, 78, and 78%, respectively (Table 3).
Synthesis of 2-arylpyridines 3a under solvent-free reaction conditions under air atmosphere at 25 °C, using C6–SO3H as catalyst. Catalyst reuse.
Entry | Catalyst reuse | Yields 3a (%) |
1 | Use | 81 |
2 | First reuse | 80 |
3 | Second reuse | 78 |
4 | Third reuse | 78 |
A rational mechanism for the formation of compound 3 activated by C6–SO3H can be established for the reaction of benzaldehyde, methyl acetoacetate and ammonium acetate. The reaction sequences are well known and involve a Knoevenagel condensation of the methyl acetoacetate with the activated benzaldehyde to give α,β-unsaturated carbonyl compounds and a condensation of another equivalent of methyl acetoacetate with ammonia to afford an enamine. The key step of the reaction is a Michael-type addition (Scheme 2) between the enamine and the Knoevenagel product. If this addition is of type 1,4, 1,4-DHPs are obtained, whereas if such an addition is of type 1,2, after an intramolecular cyclization and subsequent dehydration and oxidation, 2-arylpyridines are obtained [6]. The catalyst can act over the three initial steps of the reaction mechanism:
- • activation of the carbonyl group of the aldehyde to give with the methyl acetoacetate, the α,β-unsaturated carbonyl compounds;
- • activation of the carbonyl group in methyl acetoacetate to give with ammonia the enamine;
- • activation of the carbonyl group in the α,β-unsaturated carbonyl compounds, to condense with the enamine, to give the 2-arylpyridines, product of 1,2-Michael-type addition (Scheme 2).

Key step in the synthesis of 2-arylpyridines.
4 Conclusion
In conclusion, an efficient and environmentally friendly one-pot multicomponent procedure for the synthesis of 2-arylpyridines under solvent-free conditions at room temperature (25 °C) in the air was developed based on the use of p-sulfonic acid calix[6]arene 1 as a recycled organocatalyst. Generally, the Hantzsch reaction is expected to produce 1,4-dihydropyridines as the major product in the presence of a catalyst, solvent and heating; however, p-sulfonic acid calix[6]arene favors 1,2-arylpyridine production via 1,2-dihydropyridine formation and subsequent aromatization in an air atmosphere. Eleven examples of 2-arylpyridines were obtained with very good to excellent yields (92–62%). Furthermore, it was also demonstrated that the catalytic activity of p-sulfonic acid calix[6]arene remained unaltered even after several recovery/reutilization cycles. To the best of our knowledge, this is the first report on the application of calixarenes as catalyst in the multicomponent synthesis of 2-arylpyridines.
Acknowledgment
This work was funded by Universidad Nacional de La Plata, CONICET, ANPCyT, FAPEMIG, CAPES and CNPq. AGS and GPR are members of CONICET.