1 Introduction
The synthetic and structural chemistry of metal–organic frameworks are fast developing areas of research beaming at the rational design of novel materials for potential applications in fields ranging from catalysis, gas storage, separation, and chromatography [1]. Their physical properties, such as magnetism [2], proton conduction [3], dielectricity [4], and optics [5] are also of major interests. One of the basic rules in the synthesis is the use of multitopic organic ligands to connect metal centers through coordination bonds. The number of coordinating atoms and their position on the organic ligands as well as the coordination numbers of the metal ions set the stage in creating well-known structures in the mineral world [6]. Thus, networks adopting a variety of mineral structures such as halite, perovskite, CsCl, CdI2, SiO2, graphite, diamond and more have been possible. In contrast, there are few works dealing with monotopic ligands. We have previously explored briefly the crystal chemistry of metal mono-carboxylate in contrast to dicarboxylate [7,8]. Both finite clusters and infinite lattices have been demonstrated to form. In the present work, we explore the synthesis of metal complexes using a simple mono-carboxylate (trans-cinnamate) in the presence of a terminating ligand (1,10-phenanthroline) in view of forming finite clusters by limiting the formation of infinite polymers [9]. The two ligands chosen have nearly the same dimension in all three directions of space and the same number of coordinating atoms, but they differ in the position of the coordinating atoms (Scheme 1). In cinnamate, they are located on the short axis while they are on the long axis for phenanthroline. Both are fairly rigid ligands. In contrast to phenanthroline that can act only as a bidentate chelate ligand, cinnamate can display a range of coordination modes, viz: mondentate, terminal, bidentate, chelate, bridging, and combination of syn- and anti-modes [10].
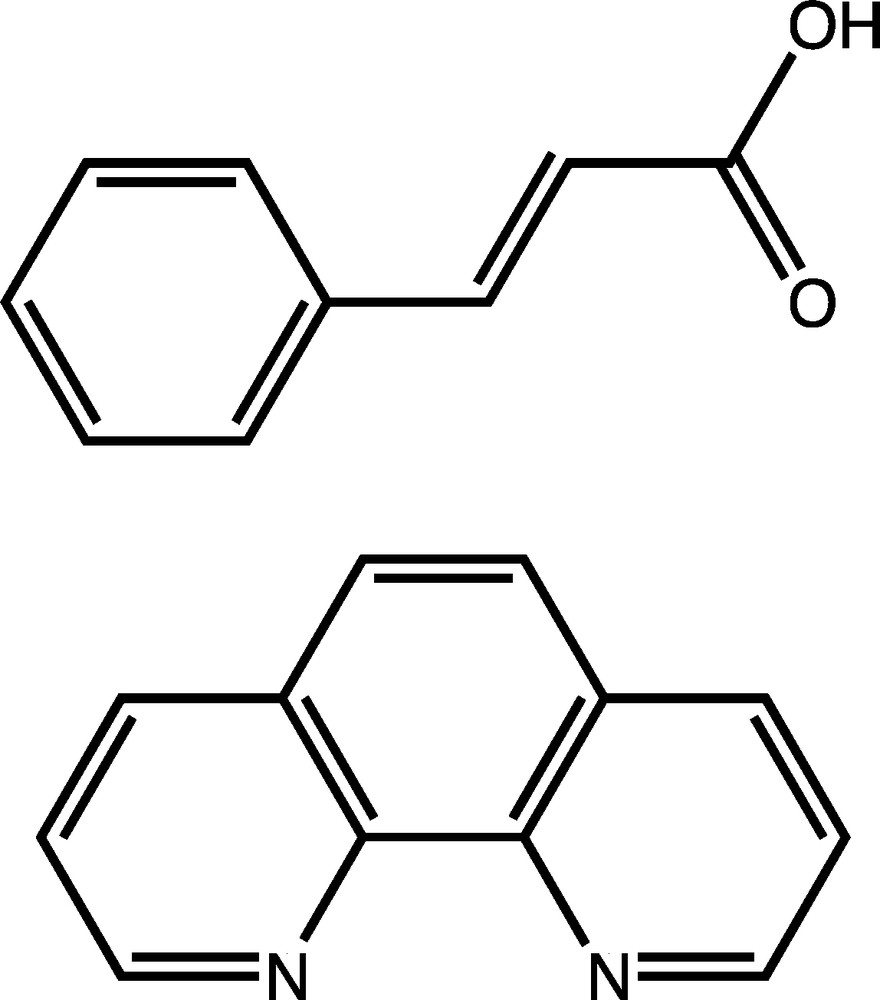
Chosen organic building blocks: trans-cinnamic acid (top) and 1,10-phenanthroline (bottom).
Here, we present the synthesis, crystal structure determinations and magnetic properties of two clusters: a trimer of cobalt Co3(trans-cinna)6(phen)2 (1) and a dimer of copper, [Cu2(trans-cinna)2(phen)2(NO3)(H2O)]NO3·CH3OH (2). The first consists of two isomers within the unit cell, which differ in the coordination mode of the carboxylate and the coordination number of the cobalt atoms. The findings suggest the segregation of the ligands by their bonding functions, where the carboxylate adopts several coordination modes enveloping the spine of the trimers, while the phenanthroline chelates at the ends. The second is a dimer bridged by the carboxylate ions and face-to-face phenanthroline, which can be described as a pincer. Interestingly, the copper atoms of the two arms have different charges and thus the molecule can be considered as an inorganic zwitterion [11].
2 Experimental
2.1 Materials
Trans-cinnamic acid, 1,10-phenanthroline, CuII(NO3)2·3H2O, and CoII(NO3)2·6H2O, were purchased from Alfa Aesar. All the reagents and solvents were used as received without further purification.
2.2 Physical measurements
Elemental analyses (C, H, N) were performed at the ‘Service de microanalyses’ of the Institut de chimie de Strasbourg (Université de Strasbourg, France). FT-IR spectra were recorded with an ATI Mattson Genesis by transmission through 0.1-mm-thick KBr pellets containing the powder samples. The magnetic measurements were made on a Quantum Design MPMS7 SQUID magnetometer. Susceptibility was measured on cooling in an applied field of 100 Oe for 1 and 5 kOe for 2 and isothermal magnetization was performed at 2 K in field of ± 70 kOe.
2.3 Synthesis of the complexes
2.3.1 Synthesis of Co3(trans-cinna)6(phen)2 (1)
Trans-cinnamic acid (0.148 g, 1 mM) and NaOH (0.04 g, 1 mM) were dissolved in a mixture of methanol–water (3:2). After several minutes of stirring Co(NO3)2·6H2O (0.210 g, 1 mM) and 1,10-phenanthroline (0.180 g, 1 mM) were combined. The mixture was placed in the Teflon liner of an autoclave, sealed and heated to 120 °C for 24 h. It was then allowed to cool to room temperature in a water bath. Brown crystals were obtained, which were washed with water and ethanol and dried in an oven at 40 °C. Anal. calcd. for Co3(C9O2H7)6(C12N2H8)2, C78H58O12Co3N4, C: 65.97; H: 4.12; N: 3.95%. Found: C: 65.49; H: 4.25; N: 3.86%.
2.3.2 Synthesis of [Cu2(trans-cinna)2(phen)2(NO3)(H2O)]NO3·CH3OH (2)
A methanol solution of Cu(NO3)2·3H2O (0.1208 g, 0.5 mM) was added under stirring to a mixture of methanol and water (2:1) containing trans-cinnamic acid (0.0074 g, 0.5 mM) and NaOH (0.02 g, 0.5 mM). After 30 min of stirring, a methanol solution containing 1,10-phenanthroline (0.09 g, 0.5 mM) was added. After few minutes, a blue precipitate appeared and was filtrated; the clear blue filtrate was left to stand undisturbed at room temperature. After several days, X-ray-quality blue crystals were obtained, which were filtrated and dried in the air. Anal. calcd. for Cu2(C9O2H7)2(C12N2H8)2(NO3)2(H2O)(CH3OH), C43Cu2H36O12N6, C: 54.03; H: 3.80; N: 8.79%. Found: C: 53.45; H: 3.84; N: 8.40%.
2.4 X-ray diffraction studies
Single crystals of 1 and 2 were mounted on glass fibers for data collections on a Nonius Kappa CCD area detector diffractometer equipped with Mo Kα radiation (λ = 0.71073 Å), using ω−2θ scans at room temperature. The parameters of the data collections (Denzo software) [12], crystallographic crystal data and final refinement parameters for 1 and 2 are given in Table 1. The structures were solved by direct methods (SHELXS97) and refined against F2 using SHELXL97 and CRYSTALBUILDER [13,14]. All non-hydrogen atoms were refined anisotropically. Hydrogen atoms were generated according to stereochemistry and refined using the riding model within SHELXL97. The absorption was not corrected (small and almost isotropic crystal shapes). Selected bond lengths and angles are given in Table 2. Table 3 lists the hydrogen bond interactions for 1 and 2. Geometrical calculations were carried out with PLATON [15] and are reported in Table 3.
Crystal data, data collection and refinement details for 1 and 2.
Compound | 1 | 2 |
Empirical formula | C78H58N4O12Co3 | C43H36N6O12Cu2 |
Formula weight | 1420.1 | 955.9 |
Temperature (K) | 173(2) | 173(2) |
Crystal size (mm) | 0.3 × 0.18 × 0.12 | 0.25 × 0.13 × 0.10 |
Crystal system, Space group | Triclinic, | Orthorhombic, Pbn21 |
a (Å), (α)° | 12.9410(4), 106.950(2) | 10.588(1), 90 |
b (Å), (β)° | 14.8210(5), 104.190(2) | 15.3883(4), 90 |
c (Å), (γ)° | 18.7280(5), 93.690(1) | 25.0987(6), 90 |
V (Å3) | 3295.20(17) | 4089.36(15) |
Z, Dc (g·cm−3) | 2, 1.43 | 4, 1.55 |
μ (mm) | 0.815 | 1.114 |
F(000) | 1416.7 | 1959.6 |
θ range (°) | 1.6–27.5 | 2.1–30 |
Index range (h,k,l) | −16/15, −18/19, −23/24 | −10/21, −10/14, −35/33 |
Reflections collected | 31948 | 27448 |
Independent reflections | 15021 | 10192 |
R(int) | 0.080 | 0.051 |
Data/restraints/parameters | 11516/0/877 | 7791/4/581 |
Goodness-of-fit on F2 | 1.029 | 1.01 |
Final R indices [I > 2σ(I)] | R1 = 0.054, wR2 = 0.119 | R1 = 0.040, wR2 = 0.084 |
R indices (all data) | R = 0.077, wR = 0.130 | R = 0.064, wR = 0.099 |
Largest diff. peak and hole/e·Å−3 | 1.151 and −0.708 | 0.46 and −0.59 |
Selected bond lengths (Å) and bond angles (°) of compounds 1–2.
Compound 1 | |||||||
Co1 | O1 | 2.009(2) | O1 | Co1 | O3 | 100.41(8) | |
Co1 | O3 | 2.007(2) | O1 | Co1 | O6 | 108.07(8) | |
Co1 | O6 | 2.019(2) | O1 | Co1 | N1 | 132.51(9) | |
Co1 | N1 | 2.076(2) | O1 | Co1 | N2 | 89.55(9) | |
Co1 | N2 | 2.180(3) | O3 | Co1 | O6 | 92.21(9) | |
Co2 | O1 | 2.105(2) | O3 | Co1 | N1 | 95.01(8) | |
Co2 | O4 | 2.068(2) | O3 | Co1 | N2 | 170.01(9) | |
Co2 | O5 | 2.117(2) | O1 | Co2 | O4 | 91.22(8) | |
Co3 | O8 | 2.016(2) | O1 | Co2 | O5 | 89.70(8) | |
Co3 | O10 | 2.011(2) | O1 | Co2 | O4a | 88.78(8) | |
Co3 | O11 | 2.140(2) | O1 | Co2 | O5a | 90.30(8) | |
Co3 | O12 | 2.221(3) | O4 | Co2 | O5 | 91.20(8) | |
Co3 | N3 | 2.106(2) | O4 | Co2 | O5a | 88.80(8) | |
Co3 | N4 | 2.160(2) | O11 | Co3 | N3 | 159.45(9) | |
Co4 | O7 | 2.101(2) | O11 | Co3 | N43 | 88.97(8) | |
Co4 | O9 | 2.054(2) | O8 | Co3 | O10 | 92.91(9) | |
Co4 | O11 | 2.133(2) | O8 | Co3 | O11 | 101.12(9) | |
O8 | Co3 | O12 | 160.87(9) | ||||
O8 | Co3 | N3 | 94.95(9) | ||||
O8 | Co3 | N4 | 91.21(9) | ||||
O10 | Co3 | O11 | 99.10(8) | ||||
O10 | Co3 | O12 | 95.19(9) | ||||
O10 | Co3 | N3 | 92.74(9) | ||||
O10 | Co3 | N4 | 170.05(9) | ||||
O11 | Co3 | O12 | 60.49(9) | ||||
O12 | Co3 | N3 | 101.94(10) | ||||
O12 | Co3 | N4 | 83.69(9) | ||||
N3 | Co3 | N4 | 77.88(9) | ||||
O7b | Co4 | O11 | 88.33(9) | ||||
O7 | Co4 | O9 | 91.71(9) | ||||
O9 | Co4 | O11 | 92.39(8) | ||||
Symmetry Code: a: 1–x, 1–y,–z; b: 2–x,–y, 1–z | |||||||
Compound 2 | |||||||
Cu1 | O3 | 2.196(2) | O3 | Cu1 | N2 | 94.30(9) | |
Cu1 | O4 | 1.948(2) | O4 | Cu1 | O5 | 94.06(9) | |
Cu1 | O5 | 1.936(2) | O4 | Cu1 | N1 | 167.05(10) | |
Cu1 | N1 | 2.015(3) | O4 | Cu1 | N2 | 91.91(10) | |
Cu1 | N2 | 2.020(3) | O5 | Cu1 | N1 | 91.18(10) | |
Cu2 | O7 | 2.154(2) | O5 | Cu1 | N2 | 172.90(9) | |
Cu2 | O8 | 1.942(3) | N1 | Cu1 | N2 | 82.19(10) | |
Cu2 | O11 | 1.936(2) | O7 | Cu2 | O8 | 90.77(9) | |
Cu2 | N3 | 2.028(3) | O7 | Cu2 | O11 | 93.69(8) | |
Cu2 | N17 | 2.012(3) | O7 | Cu2 | N3 | 96.68(9) | |
O7 | Cu2 | N17 | 100.36(10) | ||||
O8 | Cu2 | O11 | 93.25(9) | ||||
O8 | Cu2 | N3 | 169.64(10) | ||||
O8 | Cu2 | N17 | 89.93(10) | ||||
O11 | Cu2 | N3 | 93.44(10) | ||||
O11 | Cu2 | N17 | 165.55(10) | ||||
N3 | Cu2 | N17 | 81.68(10) | ||||
Cul | 03 | N15 | 114.43(17) | ||||
O3 | Cu1 | O4 | 87.68(8) | ||||
O3 | Cu1 | O5 | 89.77(9) | ||||
O3 | Cu1 | N1 | 104.20(9) |
Hydrogen bond lengths (Å) and angles (°) for compounds 1–2.
Compound 1 | ||||||
D | H | A | DH | H···A | D···A | DH···A |
C2 | H2 | O4 #1 | 0.95 | 2.78 | 3.710(3) | 166 |
C68 | H68 | O5 #2 | 0.95 | 2.81 | 3.632(4) | 145 |
C74 | H74 | O8 #3 | 0.98 | 2.79 | 3.683(4) | 151 |
Symmetry code: #1: 1–x, 1–y,–z; #2: 1–x, −y, −z; #3: 1–x, −y, 1–z | ||||||
Compound 2 | ||||||
D | H | A | DH | H···A | D···A | DH···A |
O1W | H1W | O5 #1 | 0.87(3) | 1.97(3) | 2.828(3) | 168(3) |
O1W | H2W | O10 | 0.81(3) | 2.08(3) | 2.883(4) | 173(3) |
O8 | H8A | O9 | 0.94(3) | 2.02(3) | 2.861(4) | 149(3) |
O8 | H8A | O11 | 0.94(3) | 2.59(3) | 3.405(5) | 146(2) |
C2 | H2 | O6 #1 | 0.93 | 2.48 | 3.375(4) | 162 |
C3 | H3 | O7 | 0.93 | 2.57 | 3.456(4) | 161 |
C8 | H8 | O9 #2 | 0.93 | 2.59 | 3.370(4) | 142 |
C15 | H15 | O6 #3 | 0.93 | 2.51 | 3.174(5) | 128 |
C19 | H19 | O7 #1 | 0.93 | 2.49 | 3.178(4) | 131 |
C23 | H23 | O5 #4 | 0.93 | 2.56 | 3.328(4) | 141 |
C36 | H36 | O11 #5 | 0.93 | 2.44 | 3.308(5) | 155 |
C40 | H40 | O10 #6 | 0.93 | 2.51 | 3.328(4) | 147 |
Symmetry code: #1: 3/2–x, −1/2 + y, z; #2: 2–x, 1–y, 1/2 + z; #3: 1/2 + x, 3/2–y, −1/2 + z; #4: 1/2–x, −1/2 + y, z; #5: 1/2–x, 1/2 + y, z; #6: 3/2–x, 1/2 + y,z |
3 Results and discussion
3.1 Crystal and molecular structure of [bis(μ2-cinnamato-O,O′)-tetrakis-(μ2-cinnamato-O,O)-bis(1,10-phenanthroline-N,N′)-tris-cobalt(II)]·[bis(μ2-cinnamato-O,O′)-tetrakis-(μ2-cinnamato-O,O,O′)-bis(1,10-phenanthroline-N,N′)-tris-cobalt(II)] (1)
The key feature of this structure is the presence of two independent trimeric neutral molecules of the same formulation, [Co3(cinna)6(phen)2]. As both molecules contain a center of symmetry, the asymmetric unit of 1 contains four independent cobalt sites (two per trimer) of different occupancies (center one of 50% and the end ones of 100%), six cinnamates and two phenanthrolines (Fig. 1). The two trimers, labeled A and B, are almost linear and differ in the coordination numbers of the terminal cobalt atoms and the mode of coordination of two out of the six cinnamate carboxylate. The terminal cobalt atoms arrange themselves in distorted trigonal bipyramidal and octahedral geometries in A and B, respectively. In both cases, phenanthroline acts as a chelate ligand and is bonded to the end metal atoms of the trimers, which principally prevents the structure from propagating into linear chains. The carboxylate of the cinnamate bridges the cobalt atoms and their phenyl groups radiate out perpendicular to the spine of the trimer. Thus, the magnetic cobalt atoms of each trimer are magnetically shielded from their neighbors without chemical bonds and the Co3···Co3 separation is ≥ 5.98 Å.
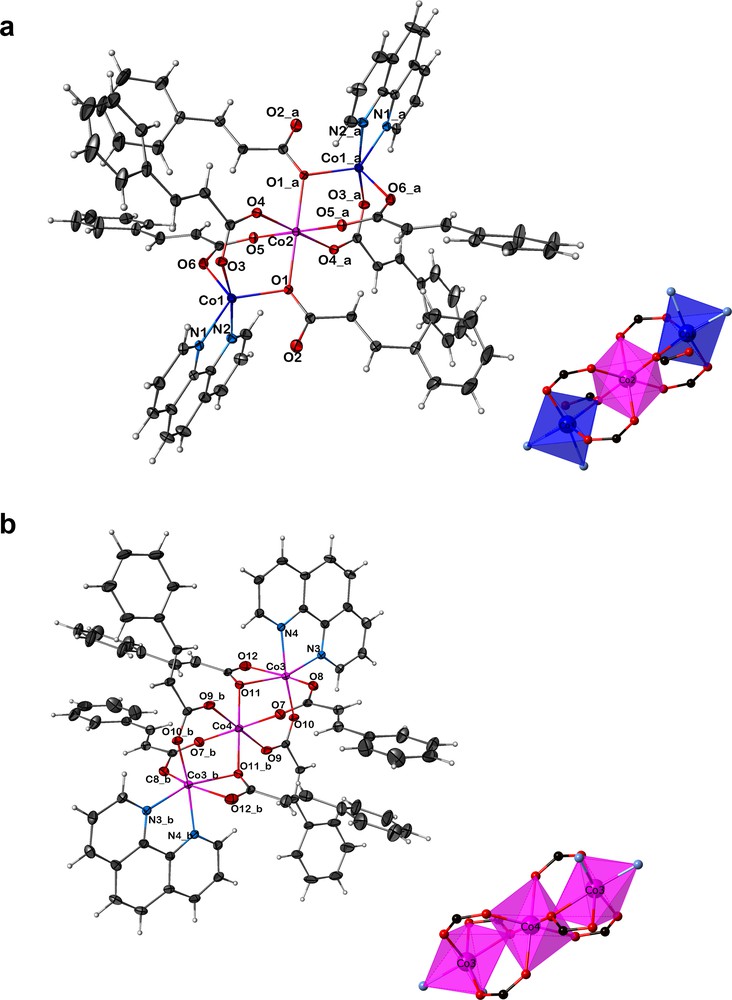
(Color online.) a: ORTEP and polyhedral views of 1 [Co3(cinna)6(phen)2]; molecule A: [bis(μ2-cinnamato-O,O′)-tetrakis-(μ2-cinnamato-O,O′)-bis(1,10-phenanthroline-N,N′)-tris-cobalt(II)]. The ellipsoids enclose 50% of the electron density, symmetry code: a = 1–x,1–y, −z; b: ORTEP and polyhedral views of 1 [Co3(cinna)6(phen)2]; molecule B: [bis(μ2-cinnamato-O,O,O′)-tetrakis-(μ2-cinnamato-O,O′)-bis(1,10-phenanthroline-N,N′)-tris-cobalt(II)]. The ellipsoids enclose 50% of the electron density, symmetry code: b = 2–x, −y,1–z.
The two crystallographic independent Co(II) ions of trimer A are in different coordination geometries (Fig. 1a), but are the same for trimer B. The central Co2 and Co4 atoms in both trimers adopt a octahedral coordination and are located on the crystallographic inversion center; the Co–Co distances in molecules A and B are 3.327(2) Å and 3.487(2) Å, respectively. The molecule A, [bis(μ2-cinnamato-O,O)-tetrakis-(μ2-cinnamato-O,O′)-bis(1,10-phenanthroline-N,N′)-tris-cobalt(II)], involves two different coordination geometries in which the three linear cobalt atoms are bridged by six carboxylate groups, four of which act as bidentate syn-syn η1:η1:μ2 bridging mode, linking the Co2 with the peripheral Co1 atoms, and two as syn-anti, η2:μ2 monodentate bridging mode (Scheme 2). The central atom Co2 is octahedral with six cinnamate oxygen atoms (O5, O5a, O1, O1a, O4, O4a), with trans angles of 180° and Co2–O distances ranging from 2.068(2)–2.117(2) Å. The terminal metal Co1 adopts a distorted trigonal bipyramid geometry with the carboxylate O3 atoms and pyridyl N2 atoms occupying the apical positions. They are surrounded by two pyridyl nitrogen atoms (N1 and N2) at a distance of 2.076(2) and 2.180(2) Å, respectively, and three carboxylate oxygen atoms (O1, O3, O6) at a distance in the range of 2.007(2)–2.019(2) Å. The molecule B, [bis(μ2-cinnamato-O,O,O′)-tetrakis-(μ2-cinnamato-O,O′)-bis(1,10-phenanthroline-N,N′)-tris-cobalt(II)], involves all cobalt atoms in octahedral geometry in which the three linear cobalt atoms are bridged by six carboxylate groups, four of which act as bidentate syn–syn η1:η1:μ2 fashion linking Co4 with the peripheral Co3 atoms (Fig. 1b), and two as both bidentate bridging and bidentate chelating syn, syn, anti η1:η2:μ2 (Scheme 2). The central atom Co4 is octahedral with six cinnamate oxygen atoms (O7, O7a, O9, O9a, O11, O11a), with trans angles of 180° and Co4–O distances ranging from 2.054(2) to 2.133(2) Å. The terminal metal Co3 atoms are in a distorted octahedral environment, surrounded by two pyridyl nitrogen atoms (N3 and N4) at a distance of 2.106(2) and 2.160(2) Å, respectively, and four carboxylate oxygen atoms (O8, O10, O11, O12) at a distance in the range of 2.011(2)–2.221(2) Å. The apical positions are occupied by N4 and O10 with a trans angle of 170.05(9)°.
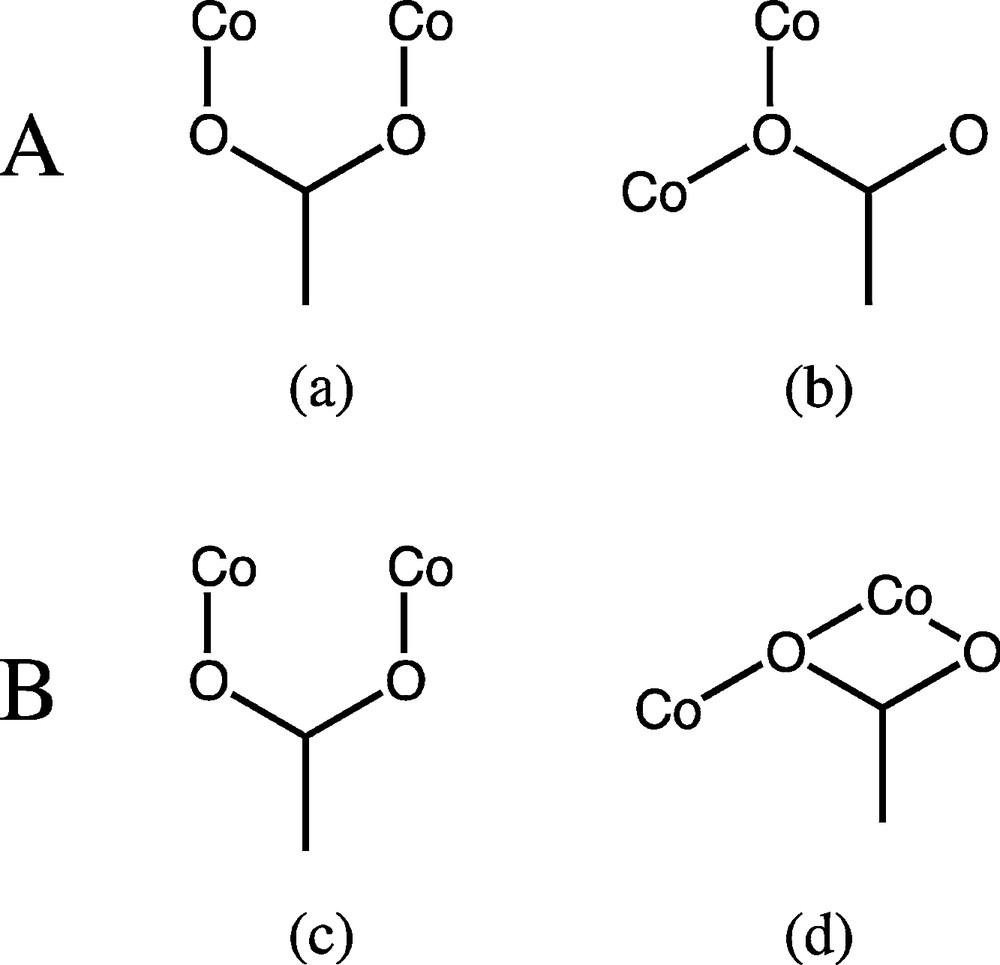
Coordination modes adopted by the carboxylates in the structure of 1. Molecule A: syn–syn (a) and monodentate bridging (b), and molecule B: syn–syn (c) and anti-chelate (d).
The trinuclear linear array and the bridging modes of carboxylate groups in molecules A are observed in two complexes of zinc with the formula [Zn3(cinna)6L2]·H2O (L = 2,2′-bipyridine or 1,10-phenanthroline), while those of molecule B are found for two isostructural complexes of cobalt and manganese with the formula [M3(cinna)6(bpy)2] (M = Co, Mn), respectively [9]. It is interesting to note that while the above complexes contain either molecule A or B, the present complex 1 contains both types (Fig. 2). Our findings and those mentioned above point to the segregation of the two ligands by their bonding functions, where the carboxylate adopts several modes enveloping the spine of the trimers while the phenanthroline caps the ends. The two independent trimers are segregated within alternate layers parallel to the ab-plane where that of molecule A is at c/2 and that of molecule B is at the origin.
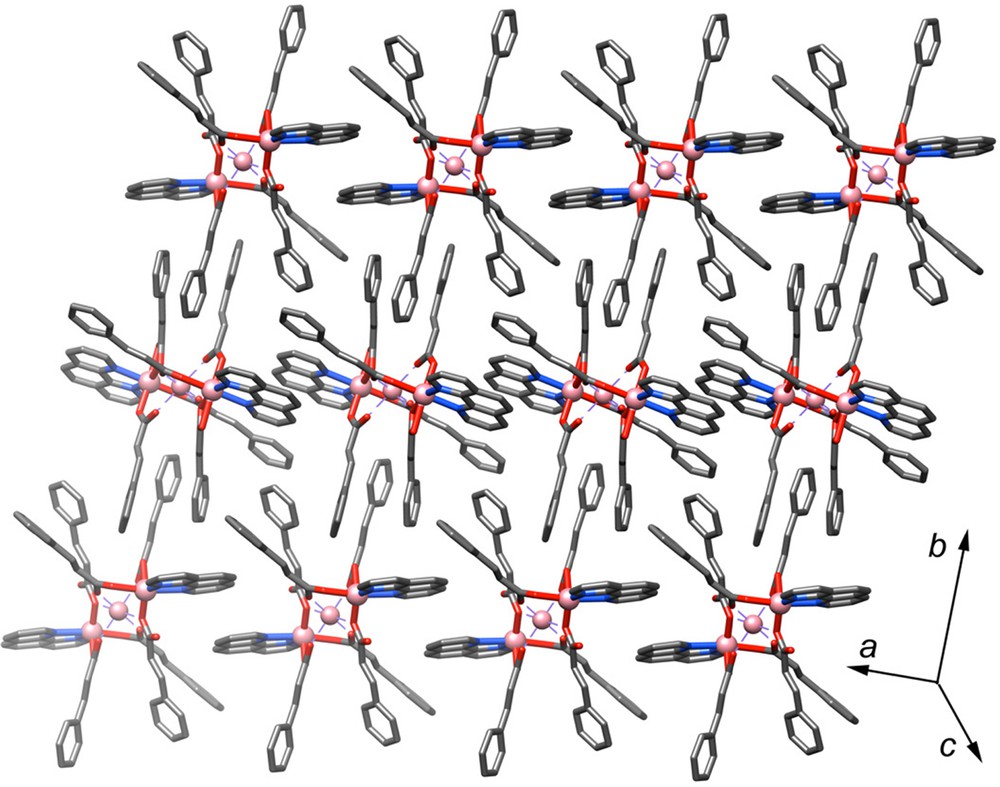
(Color online.) Packing of the trimers within the structure of 1 (hydrogen atoms have been omitted for clarity).
In the crystal structure of 1, there are two CH···O weak hydrogen-bonding interactions between phenanthroline CH atoms and carboxylate oxygen atoms. The molecule A involves 1,10-phenanthroline groups donating H68 atoms to the carboxylate O5 atoms [d(C68···O5#1) = 3.632(4) Å, ∠ C68-H68···O5#1 = 145°, symmetry code #1: 1–x, −y, −z] generating one-dimensional chains parallel to [011] direction, and molecule B involves likewise through 1,10-phenanthroline groups donating H74 atoms to the carboxylate O8 atoms [d(C74···O8#2) = 3.683(4)Å, ∠ C74–H74···O8#2 = 151°, symmetry code #2: 1–x, −y, 1–z] into one-dimensional chains along the axis at (0,0,1/2). The two sets result in a 2D network.
3.2 Crystal and molecular structure of [Cu2(cinna)2(phen)2(NO3)(H2O)]NO3·MeOH (2)
Complex 2 can be described as a pincer (or ‘forceps’) molecule, where the two carboxylate act as the elbow and the phenanthroline as the pincer arms. 2 consists of a positively charged complex [Cu2(cinna)2(phen)2(NO3)(H2O)]+, one uncoordinated nitrate group and one solvent methanol molecule. The two carboxylate groups bridge the metal centers in a bidentate syn–syn η1:η1:μ2 mode and the Cu–Cu distance is 3.0555(5) Å (Fig. 3). The Cu1 atom is penta-coordinated in a square pyramidal environment by two cinnamate oxygen atoms (O2, O4) at a distance of 1.936(2) and 1.948(2) Å, respectively, and surrounded by two pyridyl nitrogen atoms (N3; N4) from the 1,10-phenanthroline, with a distance of 2.014(2) and 2.019(2) Å, respectively. The coordination sphere is completed by a monodentate nitrate ion via the oxygen atom O5 in the apical position at a distance of 2.197(2) Å. The Cu2 atom is also penta-coordinated in a square pyramidal environment by two cinnamate oxygen atoms (O1, O3) at a distance of 1.936(2) and 1.942(2) Å, respectively, and surrounded by two pyridyl nitrogen atoms (N1; N2) from the 1,10-phenanthroline, with a distance of 2.012(2) and 2.027(2) Å, respectively. The coordination sphere is now completed by an oxygen atom O1W from a water molecule in the apical position at a distance of 2.154(2) Å.
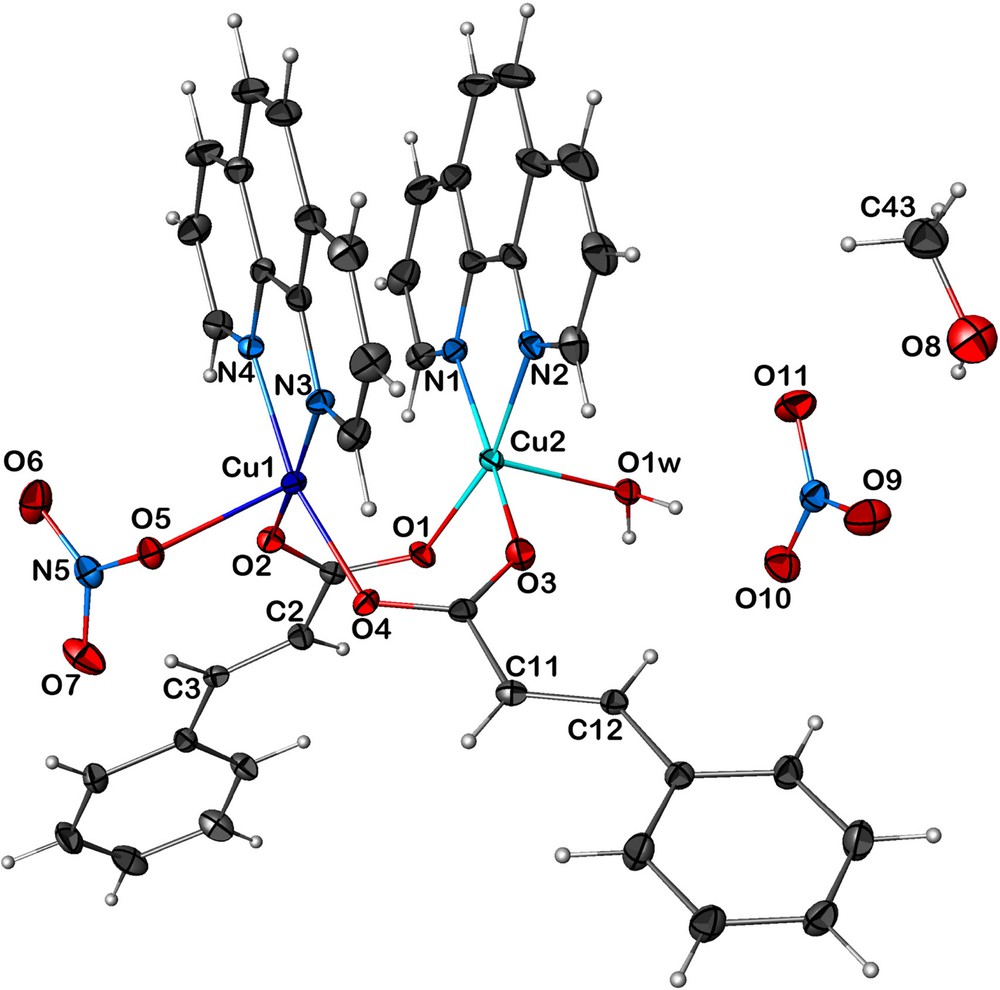
(Color online.) ORTEP view of 2 [Cu2(cinna)2(phen)2(NO3)(H2O)]NO3·MeOH with the labeling scheme. The ellipsoids enclose 50% of the electronic density.
The structure of compound 2 is stabilized by an extensive network of OH···O and CH···O hydrogen-bonding interactions. The crystal structure can be described as a three-dimensional set of chains, interlinked by medium-to-weak hydrogen bonds of the type OH···O and CH···O, respectively along the a-axis, between O1WH1W···O5 and C40H40···O10. These chains are interconnected along the b-axis via weak hydrogen bonds of the type CH···O between C2H2···O6 and C19H19···O7. These chains are also interconnected along the c-axis via medium-to-weak hydrogen bonds of the type OH···O and CH···O, respectively, between O1WH2W···O10 and C8H8···O9 and C15H15···O6 (Fig. 4). In addition to these hydrogen bonds, we also note the presence of π–π stacking interactions within the same molecule, between centroid (cg1) of the N1–C19 pyridyl ring and the centroid (cg2) of the N3–C31 pyridyl ring, and between centroid (cg3) of the N2–C28 pyridyl ring and the centroid (cg4) of the N4–C40 pyridyl ring, with a distance cg1···cg2 and cg3···cg4 of 3.800 and 3.841 Å, respectively.
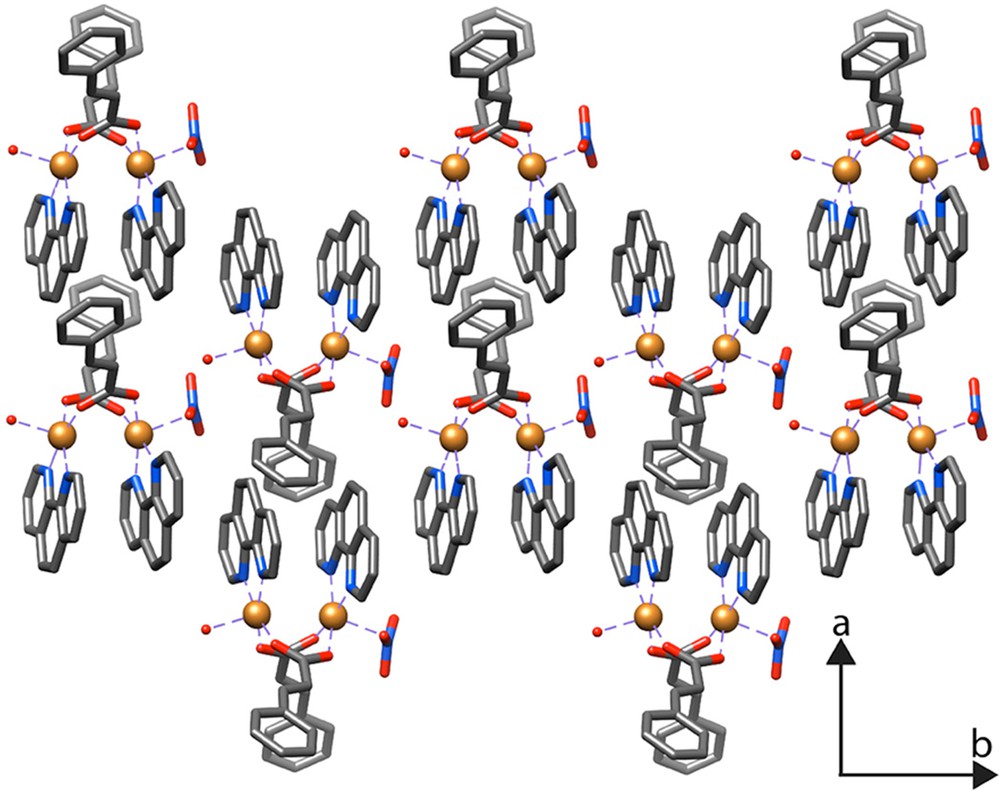
(Color online.) Packing of the molecules in the structure of 2 (hydrogen atoms have been omitted for clarity).
3.3 Spectroscopic properties
The infrared spectra in the range 400–4000 cm−1 cover mainly the vibrations of the organic ligands, and thus the observed bands have been assigned based on group frequencies with consideration of their energies and widths. 1 displays the ν(CH) symmetric stretching bands at 3060 and 3025 cm−1 and its asymmetric stretch at 2924 cm−1. The corresponding bands for 2 are at 3060 and 2922 cm−1. The vibrations of the cinnamate carboxylate groups are differentiated from those of the benzene rings by their intensities and widths. Thus, for 1 the bands at 1568 and 1512 cm−1 are assigned to νas(CO2) and those at 1394 and 1348 cm−1 to νs(CO2). For 2 these bands are at 1558 and 1404 cm−1, respectively. The doubling of the νs(CO2) bands for 1 is due to the two types of carboxylate groups, bidentate bridging and monodentate bridging, present in the compounds [16]. The sharp bands below 1300 cm−1 are principally those from the benzene rings of the ligands, though among them some bending carboxylate bands, δ(CO2), will also be present. The bands from the coordinated and non-coordinated nitrate ions, expected around 1590, 1380 and 840 cm−1, are heavily overlapped with other bands of carboxylate and the benzene rings. The metal ligand vibrations are expected below 500 cm−1.
3.4 Magnetic properties
The magnetic properties of the compounds were studied as randomly oriented polycrystalline samples. Fig. 5a shows the susceptibility and its inverse as a function of temperature in a fixed field of 100 Oe of compound 1. The data above 100 K was fit to the Curie–Weiss law with a Curie constant of 8.098(12) emu K/mol (i.e. 2.696 emu K/Co) and a Weiss temperature of −13.5(3) K. While the Curie constant is within the expected experimental values for orbital active Co(II) ion, the magnitude of the negative Weiss constant corresponds to the expected effect of the spin–orbit coupling [2]. It is therefore difficult to assign the type of interaction. There is a sharp anomaly at ca. 50 K, which is due to a very small trace of a ferromagnetic impurity whose nature has so far eluded identification. Our present guess based on previous knowledge and judging from the high temperature of the transition is that it may be a cobalt hydroxide layered compound [17], which is poorly crystalline. From the chemical analyses, data and the small moment observed at the transition, we conclude that it is only present in trace quantity.
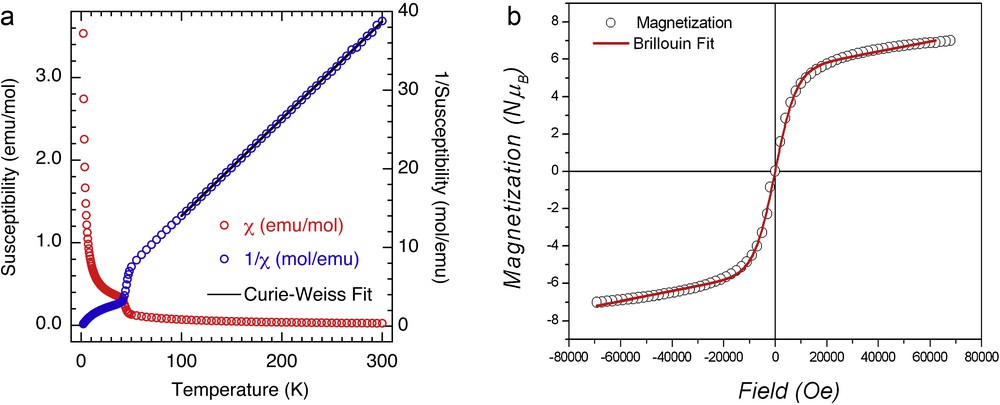
(Color online.) a: Magnetic susceptibility (red circles) and its inverse (blue circles) as a function of temperature measured in a field of 100 Oe. The solid black line is the Curie–Weiss fit for the high temperature data; b: isothermal magnetization of 1 at 2 K superposed by the fit to an Brillouin function (solid red line), gS = 0.33938 × 7.87759 = 2.67, g = 5.3.
The isothermal magnetization at 2 K (Fig. 5b) has the form of a Brillouin function with a saturation magnetization of 7 μB in a field of 70 kOe. The saturation value corresponds to that expected for three Co(II). The absence of hysteresis indirectly indicates that the amount of the ferromagnetic impurity is very small.
The temperature dependence of the magnetic susceptibility of 2 in 5 kOe is shown in Fig. 6a and its isothermal magnetization at 2 K in Fig. 6b. The susceptibility displays a smooth increase from 350 K to a peak at ca 100 K and then to a minimum at 15 K. The peak is characteristic of low-dimensional behavior with near-neighbor antiferromagnetic coupling. However, the minimum at low temperature is due to a contribution from paramagnetic species. Consequently, the data have been fitted to the equation below, where the first term is the model for a dimer [18], the second term is due to a paramagnetic species of S = 1/2 present in a proportion ρ. The best fit gives J = –153(1) K, g = 2.164(5), ρ = 0.97.
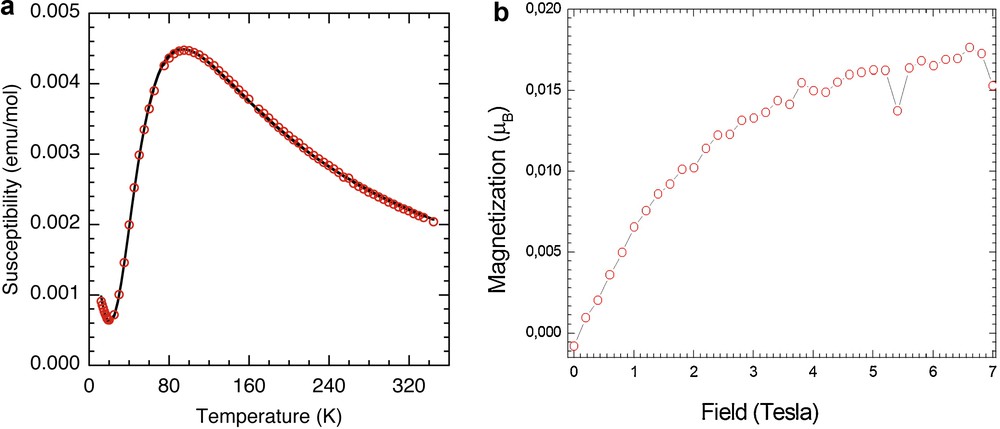
(Color online.) a: magnetic susceptibility (red circles) of 2 as a function of temperature measured in a field of 5 kOe superposed by the fit to an S = 1/2 dimer model (solid black line); b: isothermal magnetization of 2 at 2 K.
The isothermal magnetization (Fig. 6b) is quite low, confirming the large singlet–triplet gap of the dimer.
4 Conclusion
Using two ligands of the same shape but with different bonding functions, trans-cinnamate with potentially multiple coordination modes and phenanthroline with a unique chelate bonding mode, two new complexes, [Co3(cinna)6(phen)2] and [Cu2(cinna)2(phen)2(NO3)(H2O)]NO3·MeOH, have been isolated. The structure of the former contains two closely related isomers (A and B) unlike the zinc and manganese analogues that contain solely A and B, respectively. The molecular structures also point to the segregation of the ligands by their bonding functions, where carboxylate adopts several modes enveloping the spine of the linear trimers, while phenanthroline caps the ends. For the latter, carboxylate bridges the metals and phenanthroline chelates to each metal forming a pincer molecule with a different charge on each primary coordination sphere. The magnetic properties of the cobalt compound correspond to that of a paramagnet and those of the copper compound display strong antiferromagnetic coupling.
Acknowledgements
The authors thank the Université de Strasbourg and the CNRS (France) and MESRS (Algeria) for financial supports. A. L. thanks the Algerian MESRS for the financial support (PNR project).
Appendix A Supplementary material
Crystallographic data for 1 and 2 as CIF files (excluding structure factors) have been deposited at the Cambridge Crystallographic Data Centre as Supplementary publication No. CCDC 875421 & 875422. Copies of the data can be obtained free of charge on application to CCDC, 12, Union Road, Cambridge CB2 1EZ, UK (fax: (+44) 1223 336 033; e-mail: deposit@ccdc.cam.ac.uk).