1 Introduction
Environmental protection plays a very important role in sustainable development. Among the most harmful pollutants, volatile organic compounds and nitrogen oxides may be mentioned.
The most common pollutants are volatile organic compounds (VOC), which are present in most manufacturing processes as raw materials, solvents, intermediates, decomposition products, etc. The influence of VOCs on health and human life is significant, so they should be removed. Several types of methods are considered for abating these emissions, such as absorption [1], adsorption [2], scrubbing, biodegradation [3], thermal and catalytic incineration [4].
Nitric oxide NO is capable of catalyzing the decomposition of ozone, which protects the Earth against harmful UV rays. Nitrogen oxides influence also our health. The main negative feature is their carcinogenicity. Even their low concentrations in air can be very dangerous. Nitrogen oxides are formed in the processes of energy production, both by stationary and mobile sources. NO may be removed from outgases from stationary sources by selective catalytic reduction with ammonia [5–14]. Similar reaction is considered for outgases from diesel engines. However, ammonia in itself is harmful to environment and new reducing agents are studied, among them alcohols.
The reaction of SCR–NO–alcohol was studied for different catalysts: Pt/Al2O3 [15], Ag/SBA [16], Co3O4, CoFe2O4 [17] as well as for different alcohols: CH3OH [18–20], C2H5OH [21–27], propanol or butanediol [15,28]. Bion et al. [29] studied 2% Ag/Al2O3 using ethanol as a reducing agent. In the temperature range between 350 and 500 °C, the promotion of Al2O3 with 2% Ag increased NO conversion from 10% to 30%. For comparison, Ag/SiO2 catalysts did not show any activity in this process. Boutros et al. [16] tested Al-SBA-15 catalysts in the same reaction, but under other conditions. Conversion of NOx to N2 and ethanol to COx depended on the method of impregnation with AgNO3 (ESI-excess precursor solution method or IW-incipient wetness). For catalyst Ag/Al-SBA-15 (ESI) the maximum NOx conversion was below 50% but it was higher than for Ag/Al-SBA-15 (IWI). Additionally, Ag/Al-SBA-15 (ESI) was more efficient in ethanol oxidation than Ag/Al-SBA-15 (IWI) [16].
Reduction of NO with ethanol was also studied with Fe- and Co-zeolites by Dźwigaj et al. [× 28]. The catalytic activity was relatively high for Fe0.3SiBEA and Fe0.9SiBEA or Co0.3SiBEA and Co0.7SiBEA, containing tetrahedral Fe(III) or Co(II) ions.
Selectivity to N2 exceeded 90% for NO conversion from 25 to 60%. Oxidation of VOC was carried out by Białas et al. [30] who used copper-aluminum oxide catalysts with different amounts of copper. The Cu0.64 sample exhibited the best catalytic activity among all tested materials. The temperature of 50% conversion was equal to 303 °C over this catalyst. Ross and Clancy [18] studied NO reduction with methanol using catalysts prepared by co-precipitation from solutions containing the appropriate metal nitrates: Cu, Zr, Ce, Y, and La. In the temperature range from 300 to 400 °C, the highest activity and selectivity to nitrogen were observed for CuCeZr and reached 30% and 100%, respectively.
Joubert et al. [15] studied the comparison between alcohols and diols as reducing agents in NO-SCR-alcohol. NO conversion formed a sequence: butane-1,3-diol > butane-1,4-diol > propane-1,3-diol > propan-1-ol > ethanol > methanol > propan-2-ol > propane-1,2-diol. The maximum NOx conversion was between 20 and 44%.
The reactivity between ethanol and NOx can be enhanced by NO2 formation. Radlik et al. [31] have shown that the ceria–zirconia supported copper catalysts are able to oxidize NO to NO2 even at low temperature. It can be explained by the strong oxidizing properties of the surface oxygen of ceria–zirconia. Łamacz et at [32] showed that the NO adsorbs on CuO/CeZrO2 to form nitrites which are oxidized to nitrates. During HC-SCR reaction, these surface nitrates react with co-adsorbed toluene, giving RNO2. Similar conclusions are given by Adamowska et al. [33] who tested a ceria-zirconia catalyst and found that the only function involved in the deNOx process was the oxidation of NO to NO2 on support or on Rh2O3 particles.
The aim of this paper was to study the influence of the presence of NO on the catalytic properties of aluminosilicates in ethanol oxidation. This is a first step to accommodate these materials as bifunctional catalysts in simultaneous VOC and NOx removal. This work is a continuation of the studies presented elsewhere [34].
2 Experimental
2.1 Preparation of catalysts
All samples based on vermiculite (Sigma-Aldrich GmBH) (designation VER) and montmorillonite belonging to K10 group (Fluka) were directly impregnated with an AgNO3 or a Cu(NO3)2 solution. Active materials (CuOx or AgOx) were introduced either by adsorption or incipient wetness methods as follows. Adsorption method: in the first stage solutions of 3% AgNO3 and Cu(NO3)2 were prepared. A determined amount of mineral (montmorillonite K10 or vermiculite) was introduced into the solution and mixed for 2 hours and then filtered. The resulting precipitate was dried for a week at room temperature.
Montmorillonite and bentonite were pillared with Al-polycations (designation “Al”) with chlorhydrol. (Optionally an acidic pretreatment of clays was carried out with a 20% HCl solution at a boiling temperature for 1 h [designation “H”]).
Impregnation method (incipient wetness): the calculated amount of the solution of AgNO3 or Cu(NO3)2 was added to a weighed amount of mineral and dried. Next samples were calcined in situ in an inert gas at 500 °C for 30 min [34]. The list of the studied samples including the applied preparation steps is given in Table 1.
Applied preparation steps and specific surface area for studied catalysts.
Samples | VER-Cu1 | VER-Cu3 | VER-Ag1 | VER-Ag3 | BAlCu | BHCu | BHAlCu |
Acidic activation | – | – | – | – | – | + | + |
Intercalation | – | – | – | – | + | – | + |
Adsorption (Cu/Ag nitrate, 3%) [–] | – | + | – | + | – | – | – |
Impregnation 5% [wt%Cu] | – | – | – | – | + | + | + |
SBET [m2/g] | 1 | 2 | nm | nm | 50 | 142 | 145 |
Samples | K10-Ag3 | K10-Ag1 | K10-Cu3 | K10-Cu1 | K10AlCu | MtAlCu | MtHAlCu |
Acidic activation | – | – | – | – | – | – | + |
Intercalation | – | – | – | – | + | + | + |
Adsorption (Cu/Ag nitrate, 3%) [–] | + | – | + | – | – | – | – |
Impregnation 5% [wt%Cu] | – | – | – | – | + | + | + |
SBET [m2/g] | 219 | nm | 233 | 239 | 216 | 64 | nm |
2.2 Characterization
The studied catalysts were characterized by the following methods: low-temperature N2 sorption (specific surface area SBET) at 77 K with ASAP 2010; transmission electron microscopy TEM using the microscope TEM-1011; temperature-programmed reduction TPR with Autochem 2910, operating under atmospheric pressure, using a TCD as a detector.
The catalytic tests were carried out under the following conditions: weight of catalyst 200 mg, flow rate 250 mL/min, composition of a gas mixture: NO – 500 or 0 ppm, ethanol – 1000 ppm, O2 – 7%, and He used as carrier gas. The catalyst sample was placed in the U-shape reactor. The flow rates were controlled by means of a Brooks flowmeter (series 5850). The reaction temperatures were studied in the steady state from 250 to 400 °C by steps of 50 °C. Before the catalytic runs, in each experiment a sample was subjected to the pretreatment in He flow (250 mL/min) at 500 °C for 60 min.
The reaction products were analyzed by a Siemens analyzer containing the CO/CO2 detector ULTRAMAT 6E and equipped additionally with NOx detector NOximat CLD 700AL [34].
Ethanol conversion XETOH was calculated on the basis of the stoichiometry of ethanol oxidation, as: . Selectivity to CO2 was defined as:
3 Results and discussion
3.1 Specific surface area
SBET values for the studied catalysts are given in Table 1. K10AlCu presents a much higher specific surface area than MtAlCu (216 and 64 m2/g, respectively). It may have been caused by a different distribution of active material and by a higher acidity of K10 than that of Mt, which may have led to smaller clusters of CuOx for K10. The differences in distribution are presented in TPR experiment, as discussed below. Montmorillonite K10 promoted with Cu by adsorption method (K10-Cu3) had slightly higher SBET (233 m2/g) than K10–Ag3 (SBET = 219 m2/g), which results probably from different distributions of CuOx and AgOx species (Table 1). Bentonite pillared with Al showed moderate SBET (123 m2/g), which decreased after the promotion with Cu (to 50 m2/g). Samples treated with acid, either pillared or not pillared, subsequently promoted with Cu (BHCu, BHAlCu) showed higher SBET than BAlCu. This could have been caused by a different distribution of active material. Vermiculite is characterized by low SBET, which additionally decreased after the Cu promotion [34].
3.2 Reducing properties
TPR results for the studied catalysts are summarized in Fig. 1a. The samples of commercial montmorillonite K10 promoted with copper (K10-Cu1 and K10-Cu3) show profiles different from those registered for Mt-based samples. For K10-Cu3 the reduction peak was observed at ca 370 °C with a shoulder at ca 580 °C and for K10-Cu1 at 390 °C with a shoulder at the same temperature as for K10-Cu3. The reduction peaks for 3% Ag-promoted K10 (K10-Ag3) were found at temperatures lower than for K10-Cu3: 170 °C and from 280 °C to 370 °C. In the case of K10-Ag1 the reduction peak was stretched between 400 °C and 500 °C (Fig. 1a). With the increase in the copper content, the reduction temperature increases. For Ag samples, the reduction temperature was slightly decreasing. K10AlCu has a very weak TPR profile with an indistinct maximum at ca 330 °C and a second one at around 600 °C.
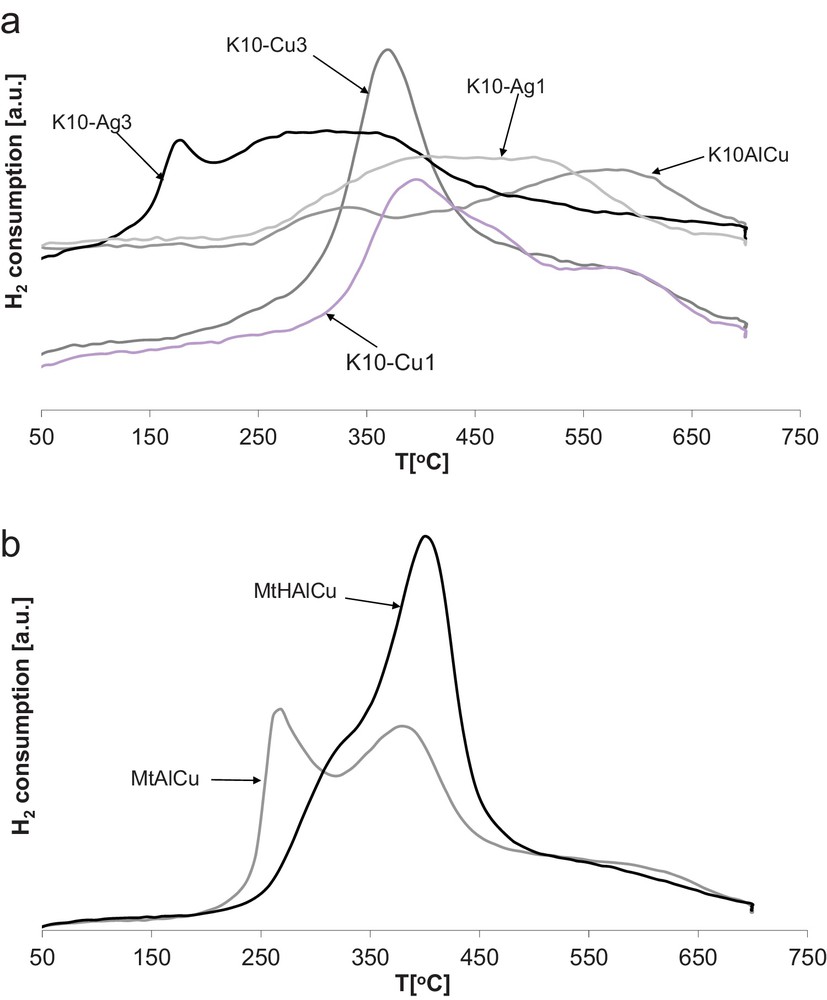
(Color online.) Temperature-programmed reduction of the studied montmorillonite samples: a: K10 samples; b: Mt samples.
Fig. 1b compares two montmorillonites from Milowice: (i) pillared with Al-hydroxycations (MtAlCu) and promoted with Cu pretreated with hydrochloric acid solution (MtHAlCu). It may be seen that the reducibility of copper species was strongly dependent on the history of montmorillonite. For these catalysts there are two types of copper species on the surface reduced at ca 260 °C and ca 390 °C for the former sample, and a shoulder at ca 320 °C, and a large peak at ca 400 °C for the latter, with shoulder at ca 320 °C. The location of the TPR peak of MtHAlCu at higher temperature in relation to MtAlCu allows us to suppose that acid activated Mt samples are less susceptible to deactivation (i.e. coke formation). The exchange of carrier to a more acidic (K10) led to a very weak TPR profile with an indistinct maximum at ca 330 °C and a second one around 600 °C.
Knapczyk [35] suggested that depending on the temperature of the maximum of TPR peaks either bulky CuO or Cu2+ clusters may be present. It is also possible that the irregular shape of the profiles and several peaks may be the result of the presence of Cu clusters. Additionally, it was proven [36] that the amount and reducibility of redox sites depend strongly on the pillaring medium, acidity and differences in the structure of the supports. TPR results both for vermiculites and bentonites were presented elsewhere [34].
3.3 Transition microscopy
TEM images in the studied catalysts are presented in Fig. 2. The materials containing silver show higher distribution (Fig. 2a) than respective supports promoted with Cu (Fig. 2b,c). Crystallites of silver are smaller than crystallites of copper, are well distributed over the surface of the tested samples. Copper generally is not uniformly distributed. In the case of Cu, numerous bigger aggregates may be observed. In some samples, e.g., K10-Cu1, active material may be observed on the outer surface of the carrier particles (Fig. 2d).

TEM for acidic (K10) and montmorilonite from Milowice (Mt) samples.
3.4 Catalytic performance
Catalytic activity and selectivity to CO2 for the studied catalysts in ethanol oxidation in the absence and the presence of NO are presented in Tables 2–4.
Catalytic activity and selectivity as a function of temperature for vermiculites in the presence or absence of NO in the reaction mixture.
Sample | Catalytic activity XETOH (%) | Selectivity to CO2 (%) | NOx removal (%NOx) | Temperature (°C) | |||
a | b | a | b | a | b | ||
VER-Ag1 | 1 | 0 | * | * | – | 0 | 250 |
4 | 1 | * | * | – | 0 | 300 | |
53 | 28 | 97 | 93 | – | 1 | 350 | |
76 | 62 | 94 | 92 | – | 0 | 400 | |
VER-Ag3 | 3 | 0 | * | * | – | 0 | 250 |
62 | 21 | 99 | 95 | – | 3 | 300 | |
91 | 81 | 99 | 99 | – | 2 | 350 | |
95 | 81 | 99 | 99 | – | 2 | 400 | |
VER-Cu1 | 2 | 0 | * | * | – | 0 | 250 |
18 | 0 | 95 | * | – | 0 | 300 | |
68 | 57 | 97 | 98 | – | 0 | 350 | |
85 | 76 | 96 | 96 | – | 0 | 400 | |
VER-Cu3 | 8 | 1 | * | * | – | 2 | 250 |
69 | 26 | 98 | 96 | – | 3 | 300 | |
94 | 62 | 99 | 98 | – | 3 | 350 | |
96 | 81 | 99 | 96 | – | 2 | 400 |
Catalytic activity and selectivity as a function of temperature for bentonites in the presence or absence of NO in the reaction mixture.
Sample | Catalytic activity XETOH (%) | Selectivity to CO2 (%) | NOx removal (%NOx) | Temperature (°C) | |||
a | b | a | b | a | b | ||
BHCu | 2 | 0 | * | * | – | 0 | 250 |
6 | 0 | * | * | – | 0 | 300 | |
39 | 29 | 79 | 79 | – | 2 | 350 | |
67 | 43 | 79 | 72 | – | 2 | 400 | |
BAlCu | 5 | 0 | * | * | – | 1 | 250 |
27 | 15 | 89 | 87 | – | 1 | 300 | |
64 | 47 | 92 | 93 | – | 2 | 350 | |
91 | 62 | 89 | 90 | – | 1 | 400 | |
BHAlCu | 6 | 5 | * | * | – | 1 | 250 |
28 | 12 | 52 | 67 | – | 3 | 300 | |
55 | 18 | 57 | 61 | – | 1 | 350 | |
72 | 20 | 63 | 65 | – | 0 | 400 |
Catalytic activity and selectivity as a function of temperature for montmorillonites (Mt and K10) in the presence or absence of NO in the reaction mixture.
Sample | Catalytic activity XETOH (%) | Selectivity to CO2 (%) | NOx removal (%NOx) | Temperature (°C) | |||
a | b | a | b | a | b | ||
K10-Cu3 | 5 | 2 | * | * | – | 2 | 250 |
21 | 12 | 60 | 50 | – | 4 | 300 | |
49 | 32 | 58 | 50 | – | 5 | 350 | |
62 | 46 | 57 | 50 | – | 4 | 400 | |
K10-Ag3 | 10 | 0 | 80 | * | – | 0 | 250 |
48 | ** | 98 | ** | – | ** | 300 | |
78 | 83 | 97 | 99 | – | 0 | 350 | |
83 | 80 | 93 | 91 | – | 0 | 400 | |
K10AlCu | 3 | 2 | * | * | – | 1 | 250 |
7 | 6 | * | * | – | 3 | 300 | |
14 | 15 | 39 | 40 | – | 4 | 350 | |
17 | 18 | 38 | 50 | – | 2 | 400 | |
MtAlCu | 7 | 0 | 67 | 0 | – | 0 | 250 |
25 | 1 | 71 | * | – | 0 | 300 | |
65 | 30 | 73 | 80 | – | 2 | 350 | |
77 | 48 | 74 | 79 | – | 0 | 400 | |
MtHAlCu | 6 | 7 | * | * | – | 1 | 250 |
35 | 30 | 77 | 80 | – | 5 | 300 | |
79 | 77 | 84 | 84 | – | 6 | 350 | |
94 | 92 | 86 | 85 | – | 3 | 400 |
3.4.1 Vermiculites
For all studied vermiculites, the presence of NO led to a slight decrease in activity of C2H5OH oxidation, while selectivity to CO2 was almost unchanged in comparison to the mixture which did not contain NO (Table 2). The stability of the catalysts was not influenced by the presence of NO (Fig. 3). Indeed, for vermiculites NO conversion was negligible. The extent of decrease in VOC oxidation activity was influenced by the temperature of reaction, the type of active material and its amount. The influence of NO on the catalytic activity in VOC oxidation decreased with temperature.
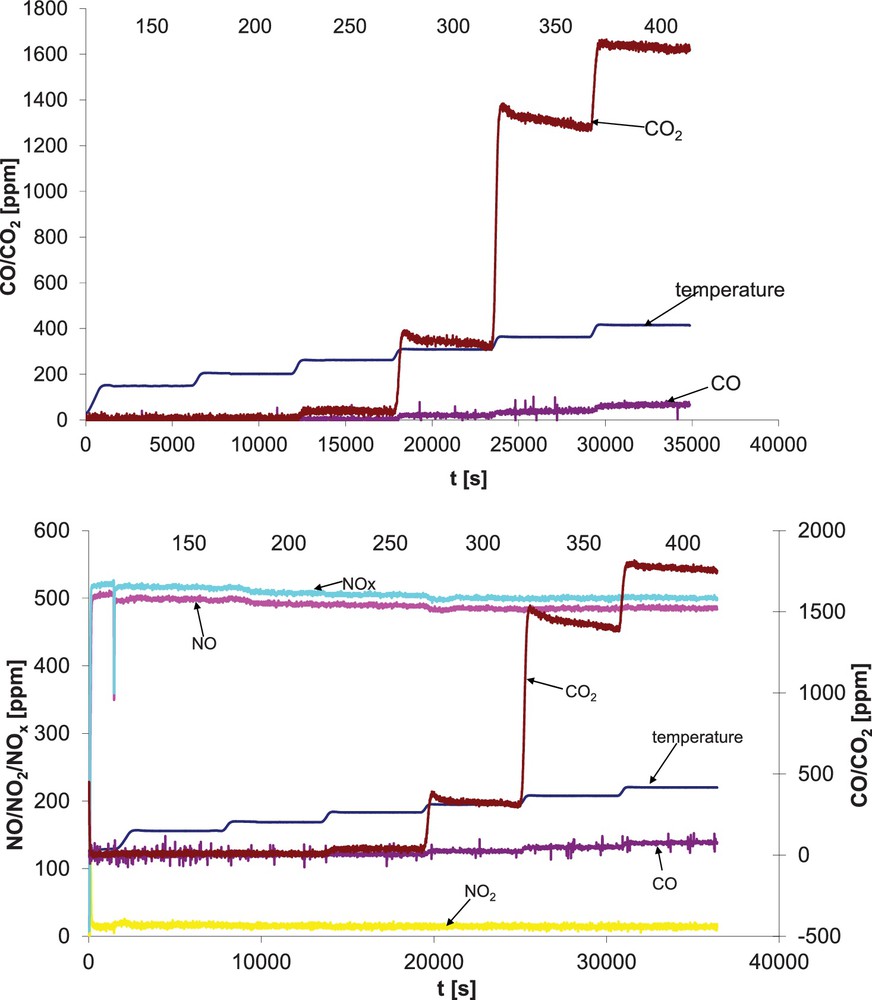
(Color online.) Activity versus time on stream for VER-Cu1. Top, in the absence of NO. Bottom, in the presence of NO.
Either the choice of the active phase or the way of its introduction on the carrier surface is important. At 300 °C for Cu-vermiculites prepared by adsorption (VER-Cu3) or by incipient wetness method (VER-Cu1), the decrease in ethanol oxidation activity was 32% and 11%, respectively. Ag-vermiculites were less influenced than Cu-containing ones; at 350 °C the introduction of NO to the reaction mixture decreased %COx from 92% to 62% for VER-Cu3 and from 91% to 81% for VER-Ag3. At 400 °C, Cu and Ag-catalysts were similarly influenced by the presence of NO.
For vermiculites, a decrease is observed in catalytic activity for ethanol conversion as a result of the addition of NO to the reaction mixture and should (at least partially) correspond to their physicochemical properties. Neither SBET, nor TEM images of the studied samples gave a clear answer to their different catalytic behavior. Only distinction between TPR-profiles of VER-Cu3 and VER-Ag3 [34] allows formulating some conclusions. A higher temperature reduction peak for VER-Ag3 corresponds to a higher onset temperature of reaction in the presence of NO in relation to VER-Cu3.
3.4.2 Bentonites
The addition of NO to the reaction system resulted in a decrease in activity in ethanol oxidation especially for BHAlCu. This effect was more apparent, when upon higher temperature the reaction was carried out (Table 3). Generally, a slight increase of selectivity to CO2 without change of selectivity for the studied samples was observed (Fig. 4). Similar to vermiculites, for bentonites conversion of NO was negligible. The influence of NO on catalytic activity in ethanol consumption depends on the way of modification of B-based catalysts. The application of Al-pillaring method (BAlCu) instead of acid activation (BHCu) as well as more complex preparation (BHAlCu) resulted in rising differences between activities registered for both types of discussed reactions.
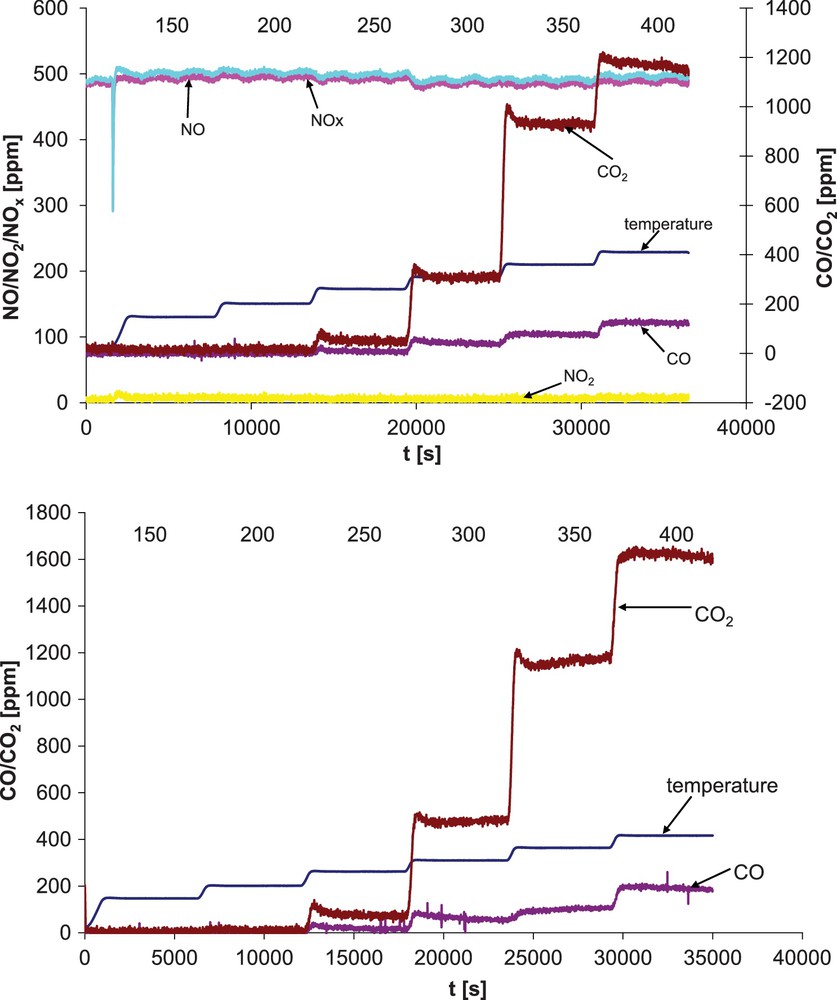
(Color online.) Activity versus time on stream for BAlCu. Top, in the absence of NO. Bottom, in the presence of NO.
At 350 °C and 400 °C, the ranking of the activity is as follows: BAlCu > BHAlCu > BHCu (in the absence of NO) and BAlCu > BHCu > BHAlCu (in the presence of NO). Selectivity for the studied samples is as follows: BAlCu > BHCu > BHAlCu independently of the presence or absence of NO in the reaction mixture.
Acid treated bentonites show much worse catalytic properties than BAlCu in both types of reaction (i.e. with or without NO). Catalytic activity of bentonites was in good correspondence with TPR results, i.e. B-samples having temperature reduction peak at higher values was generally more active both in the absence and the presence of NO in ethanol oxidation reaction.
For studied bentonites promoted with Cu depending on the preparation way, the onset temperature for VOC removal in the presence of NO was: 250 °C (for BHAlCu), 300 °C (for BAlCu), and 350 °C (for BHCu). Starting from 300 °C (for BAlCu), we can see that acidic modification alone led to an increase in the onset reaction temperature, while additional application of Al-pillaring resulted in a reverse effect.
3.4.3 Montmorillonites
3.4.3.1 K10 montmorillonite
Table 4 compares the activity and the selectivity to CO2 in the total oxidation of ethanol for the reaction mixture, with and without NO, by differently modified commercial montmorillonites K10: K10 promoted with 3% Ag (K10-Ag3) or Cu (K10-Cu3), and K10 pillared with aluminum hydroxycations and promoted with Cu (K10AlCu). The onset temperature of the oxidation reaction for a mixture containing NO was 300 °C. For montmorillonites belonging to K10 group, the presence of NO among agents influenced variously the catalytic activity and selectivity to CO2 in this reaction depending on the way of modification or/and the applied reaction temperature.
For acidic samples promoted with Ag by adsorption method (K10-Ag3), activity increased slightly in the presence of NO (by ca 5%) at 350 °C and minimally decreased (by ca 3%) at 400 °C. Unfortunately, due to unstable performance of the catalytic equipment at 300 °C, no information is available. Selectivity to CO2 was very good (between 91–99% for both mixtures). The catalytic results obtained for this sample allow us to conclude that the addition of NO to the reaction mixture had a neutral effect on ethanol oxidation.
In the case of K10-Cu3 sample, the addition of NO resulted in a decrease in catalytic activity in ethanol oxidation. This effect was more notable with rising temperature up to 350 °C, and remained stable at 350–400 °C. The presence of NO in the reaction system deteriorated also selectivity to CO2, which was equal to 50% independently of temperature. For K10-Cu3 low activity was caused by coke formation, and low selectivity to CO2 was due to the non-uniform distribution of active sites created by Cu species.
For K10AlCu, the presence of NO raised slightly the activity of C2H5OH oxidation and improved selectivity to CO2 by ca 10% at 400 °C.
Stability of the catalysts versus time on stream was similar for mixtures with and without NO (Fig. 5). Conversion of NO was negligible and was maximal (ca 5%) for K10-Cu3 at 350 °C. At temperatures 300 °C, 350 °C and 400 °C activity of C2H5OH oxidation formed a sequence: K10-Ag3 > K10-Cu3 > K10AlCu. Selectivity to CO2 was generally low, except for K10-Ag3 and formed the same sequence as in the case of activity.
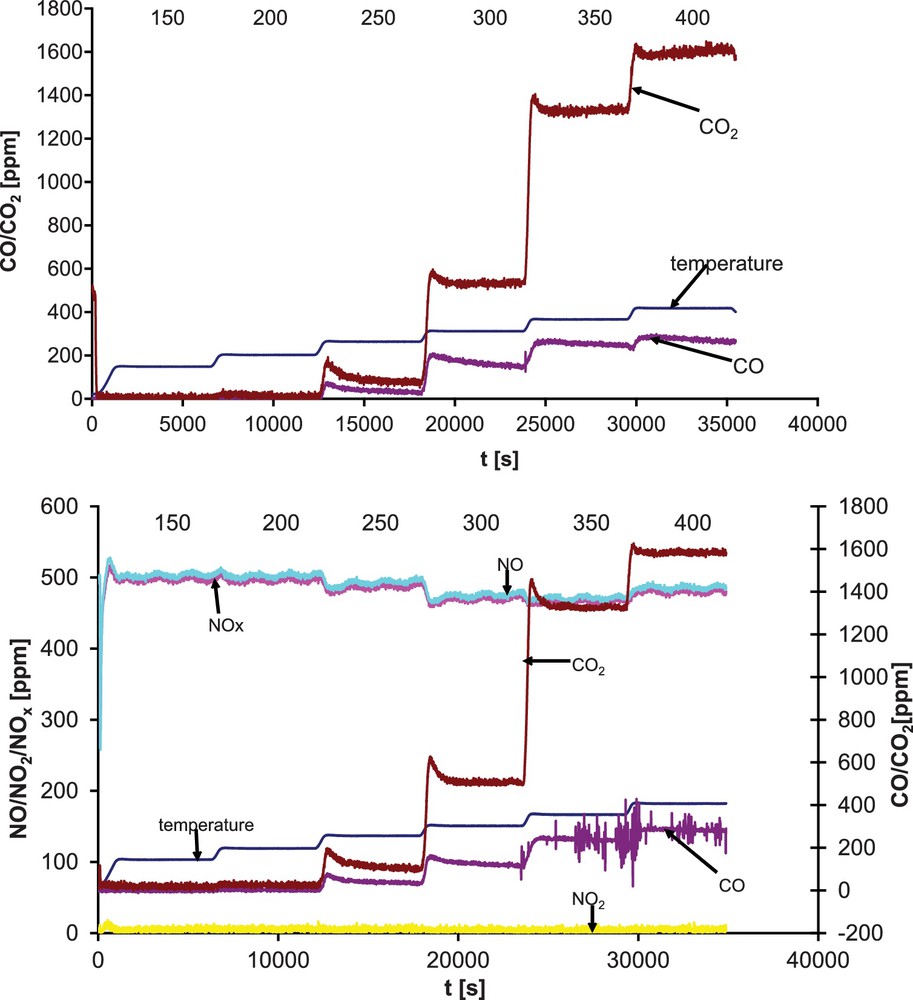
(Color online.) Activity versus time on stream for MtHAlCu. Top, in the absence of NO. Bottom, in the presence of NO.
3.4.3.2 Montmorillonite from Milowice (Mt)
Catalytic activity and selectivity in both types of discussed reaction were also performed for differently modified montmorillonite from Milowice: pillared with Al hydroxycations (MtAlCu) and promoted with Cu or modified by acid and then pillared, and subsequently promoted by Cu (MtHAlCu).
The presence of NO in the reaction mixture for MtAlCu led to the considerable decrease in activity of ca 25-30% and a small increase in selectivity to CO2 (Table 4).
For MtHAlCu the influence of the addition of NO on the activity of C2H5OH oxidation and selectivity to CO2 was minimal (2–5%). NO conversion was negligible for MtAlCu and small (ca 5%) for MtHAlCu. For the latter, NO consumption at 350 °C was the highest from all studied samples.
Montmorillonites modified by the same way, but coming from different sources (K10AlCu and MtAlCu) were compared. It turned out that the choice of the provenance of the montmorillonite sample was very important. Differences in the catalytic behavior of both types of samples result from the higher acidity of K10 in relation to Mt sample. The acid pretreatment of MtAlCu improved its acidity and catalytic properties as we can see in TPD measurements, while the replacement of Mt for K10 sample (MtAlCu vs K10AlCu or MtHAlCu vs K10AlCu) resulted in a drastic decrease in catalytic activity due to coke formation.
At temperatures 300 °C, 350 °C and 400 °C selectivity to CO2 was low and formed a sequence MtHAlCu > MtAlCu > K10AlCu. Activity formed the same sequence as in the case of selectivity, except at 300 °C in the presence of NO. Stability for the catalysts versus time on stream was similar for mixtures with and without NO (Fig. 5).
4 Conclusions
In this work, layered clays were tested as catalysts for the total oxidation of ethanol. The experiments were performed either for the gas mixture of C2H5OH + O2 + He or C2H5OH + O2 + NO + He. In both types of reaction activity in C2H5OH oxidation and selectivity to CO2 depended strongly on the type of clay, the type of active material, the way of introduction of active material (adsorption from solution or incipient wetness), and the preparation/modification procedures of clays.
Thus from this study, we can conclude that the best carrier was vermiculite. This mineral modified only by promotion with 3% of Cu(NO3)2 or AgNO3 solution (VER-Cu3 and VER-Ag3, respectively) showed conversions over 90% at temperatures 350–400 °C in the absence of NO and over 80% at 400 °C in the presence of NO in feed. Selectivity to CO2 exceeded 95% in the whole studied temperature range. Generally, acidic or acid pretreatment of clays (then promoted with Cu: K10-Cu, BHCu) led to lower activity and selectivity to CO2 than for vermiculites.
The type of active material also plays a role. On the whole, Ag-containing catalysts seemed to be more selective to CO2 and less sensitive to the presence of NO in reaction mixture- cp. VER-Ag3 vs. VER-Cu3 and K10-Cu3 vs. K10-Ag3. The comparison of structural data (SBET, reducibility, active material distribution) of the studied catalysts with catalytic performance led to the following conclusions: there was no correlation of activity or selectivity to CO2 with SBET; the distribution of an active material on the catalyst surface was important for catalytic activity. In the case of Cu-promoted catalysts much worse catalytic properties in both types of reaction were observed (in relation to Ag-analogues) due to formation of Cu clusters, when higher quantities of this metal were used for clay modification. The exceptions were Cu-vermiculites. TPR results showed generally good correlation with the catalytic performance. The presence of moderately reducible Cu sites is more important for the reaction than easily reducible sites which, possibly, undergo quick deactivation by carbonaceous deposit formation.
Predominantly, the addition of NO to the reaction mixture decreased ethanol conversion, but not as drastically as we expected. The presence of NO had no inhibiting properties on the tested reaction, but rather neutral. When K10AlCu was used as a catalyst, NO had a positive effect for VOC oxidation. Only in single cases, NO conversion was not observed. Generally, the introduction of NO into a system of agents slightly influenced selectivity to CO2 without change in the stability of the used catalysts.
Catalytic results obtained for the tested samples prompt to further study of layered clays as bi-functional catalysts for simultaneous VOC oxidation and DeNOx process.
Acknowledgements
The work was supported by AGH 11.11.210.203. The work was carried out within GDRI “Catalysis for environment” framework sponsored by PAK and CNRS.