Polyoxometalates (POMs) are nanosized anionic clusters of highly oxidized early transition metals and oxygen, whose composition can be finely tuned. They have therefore been used as active agents in scores of catalytic reactions as oxidants, [1] Brønsted [2] and Lewis [3] acids, bases, [4] etc. The recent introduction of methods for the easy preparation of organo-POMs [5] has led to more complex catalytic systems [6]. The introduction of a flexible covalent linker between the POM cluster and a catalytically active unit can lead to synergistic effects of the catalyst with the POM surface, and new or improved reactivity [6a,f].
The use of organo-POMs is particularly crucial for POM-based organocatalysis since POMs are inorganic structures, which cannot be used in organocatalysis except (rarely) as counterions of protic organocatalysts [2]. The POM in these systems remains mostly passive, limiting the dehydration of the products, [2a] possibly by buffering the reaction medium [6f]. In addition, the charge in the POM is diffused on the surface, and mainly concentrated inside the polyoxotungstic cage [7]. This might limit the scope of the method when weaker interactions (such as H-bonding) are considered.
Using covalent hybrids should bring new possibilities. We have recently illustrated this potential by tethering a substituted DMAP catalyst to a Dawson hybrid polyoxotungstate, which not only improved the reactivity, but also changed the outcome of the reaction relative to purely organic systems [6a]. We deduced that the cationic charge that developed when the pyridinium intermediate was formed led to folding toward the POM surface by way of an electrostatic interaction with the polyanionic cluster. A handful of other examples of synergistic behavior of POMs with a Lewis acidic corner, [4b–c] organometallic species, [6f] or catalytic counterions [8] have been reported. Because this reactivity enhancement is specific to organo-POMs we wish to extend its scope.
As a follow-up of our assessment of POMs in organocatalysis, we wondered whether hydrogen bonds could be harnessed to change reactivities. Since H-bond-based organocatalysis is another very important ensemble of organic reactions [9a] and H-bonds are increasingly seen as crucial features that direct organo-POM reactivity, [10] we decided to examine whether the surface of organo-POMs could also work in synergy with H-bonding organocatalysts. We selected squaramides for this purpose [11].
The POM platform chosen was the α2 organotin-substituted Dawson polyoxotungstate of general formula [P2W17O61(SnR)]7– (Fig. 1). We selected an amide to tether the squaramides, [10a] which was further separated from the POM via a semi-flexible 1,3-xylylene diamine linker. The N–H bond of the amide is likely to establish an H-bond first to an oxo of the POM surface, directing the squaramide also toward the surface. At the same time, the xylylene spacer was introduced to prevent the association of being too tight and block any reaction.
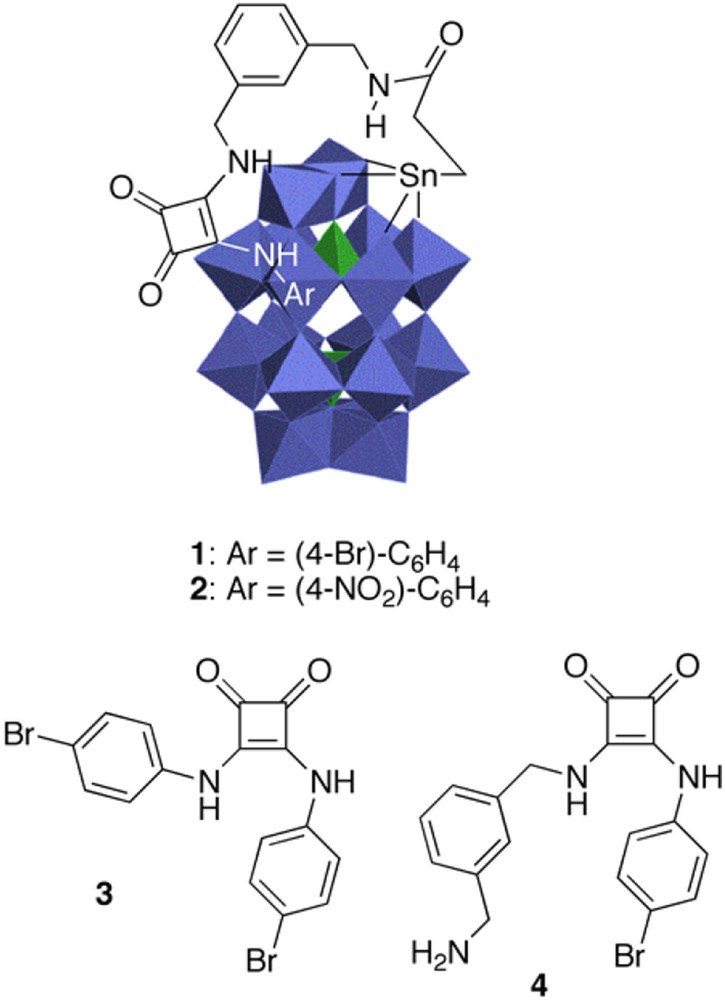
Catalysts used in this work.
Following our coupling method, an excess of squaramides (2 equiv.) was added to a solution of the mixed anhydride TBA6[α2-P2W17O61SnCH2CH2C(O)] in acetonitrile in the presence of Et3N [12]. Organo-POMs 1 and 2 were precipitated after 4 h at rt. The 31P signals moved slightly from δ = −12.2 and −10.0 ppm to −13.6 and −10.7 ppm in 1 and –13.9 and −11.0 ppm in 2. The 1H NMR signal of the amide proton at δ = 7.99 ppm in 1 (resp. 7.96 ppm in 2) confirms the covalent linkage between the Dawson structure and squaramides. In addition, benzyl CH2 and aromatic signals of the squaramide ligand were found on both hybrid structures. ESI-MS analysis of 1 and 2 showed the presence of molecular ions for both POMs stripped of 3–6 counterions, which confirmed their structures.
With the two tentative catalysts in hand, we examined the addition of diketones onto nitroalkenes. In the first experiment (Table 1, entry 1), pentanedione 5 was reacted with nitroalkene 6 with 0.5 mol % of 1 in toluene at rt. No reaction took place. A quick screening of solvents showed that no reaction took place in ethyl acetate or DCE (Table 1, entries 2–3), but that both acetonitrile and dichloromethane were suitable for the reaction (53% and 54% yield respectively, entries 4–5). We selected dichloromethane for the rest of the work. The yield increased upon heating and with a slightly increased catalyst loading (to 1.8 mol %, 99% yield, entries 6–7). These optimized conditions also worked with POM 2, albeit with a slightly lower yield (93%, entry 8).
Entry | Solvent | T | Catalyst (mol %) | Yield (%)a |
1 | Tol. | rt | 1 (0.5) | –b |
2 | AcOEt | rt | 1 (0.5) | –b |
3 | DCE | rt | 1 (0.5) | –b |
4 | MeCN | rt | 1 (0.5) | 53c |
5 | DCM | rt | 1 (0.5) | 54 |
6 | DCM | Δ | 1 (1.0) | 70 |
7 | DCM | Δ | 1 (1.8) | 99 |
8 | DCM | Δ | 2 (1.8) | 93 |
9 | DCM | Δ | – | –b |
10 | DCM | Δ | K10[P2W17O61] | –b |
11 | DCM | Δ | 3 (1.8) | 45 |
12 | DCM | Δ | 4 (1.8) | 34 |
13 | DCM | Δ | TBA7[P2W17O61Sn(CH2CH2C(=)ONHBn] (1.8) | –b |
14 | DCM | Δ | TBA7[P2W17O61Sn(CH2CH2C(=)ONHBn] (1.8)/3 (1.8) | 12 |
a Unless otherwise specified the reactions took 13 h.
b No reaction took place.
c Reaction was stopped after 8 h.
We next carried out a series of control experiments to assess the role of all the components. First, no reaction took place without catalyst (entry 9), or in the presence of either the lacunary Dawson [P2W17O61]10– (which has basic oxido sites [4a,13]), or the benzylamide Dawson hybrid TBA7[P2W17O61Sn(CH2CH2C()ONHBn] (entry 13).
On the other hand, both squaramides 3 and 4 which mimic the organic part of the hybrid led to the product with average yields (45% and 34%, entries 11–12). Finally a binary 1:1 system consisting of the benzylamide hybrid and squaramide 3 led to only 12% of 7.
Taken together, these observations show that the squaramide part is required for reactivity, and that the POM alone does not catalyze the addition. We suggest that the squaramide complexes with the nitroalkene via H-bond activation (Fig. 2), [11] and that the POM surface helps the deprotonation of the dicarbonyl partner (which is only possible because we are in an apolar medium) [4a]. The two activations are needed: the hybrid is a bifunctional catalyst. Gratifyingly, when the squaramide and the POM are not tethered, the reaction is less productive than when they are attached (compare entries 7 and 11).

Tentative model for the reactivity.
We next examined the scope of the reaction under the optimized conditions (1.8 mol % of catalyst 1 or 2 in refluxing dichloromethane for 18 h). Aryl nitroolefin substrates with either electron-withdrawing or electron-donating groups (Table 2, entries 1–5) reacted equally well. On the other hand, if the substitution of one of the methyl substituents on the dicarbonyl derivative was possible (entry 9), switching from pentanedione to heptanedione led to a dramatic loss in yield (entry 6), while further increase of the steric requirement of the dione was even more detrimental (entries 7–8). Cyclic diketones did not react (entry 12). Finally, a β-ketoester with a steric requirement similar to that of pentanedione worked well (entry 10), but a malonate did not (entry 11).
Entry | R1 | R2 | Ar | Prod. | Yd. with 1 (%) | Yd. with 2 (%) |
1 | Me | Me | o-(NO2)-C6H4 | 7 | 99 | 93 |
2 | Me | Me | Ph | 8 | 99 | 90 |
3 | Me | Me | p-Me-C6H4 | 9 | 96 | 94 |
4 | Me | Me | p-Cl-C6H4 | 10 | 96 | 94 |
5 | Me | Me | p-F-C6H4 | 11 | 99 | 88 |
6 | Et | Et | Ph | 12 | 35 | 39 |
7 | R1 = R2 = p-MeO-C6H5 | Ph | 13 | 19 | 15 | |
8 | t-Bu | t-Bu | Ph | – | S.M. | S.M. |
9 | Ph | Me | Ph | 14 | 99 | 98 |
10 | MeO | Me | Ph | 15 | 97 | 83 |
11 | MeO | MeO | Ph | – | S.M. | S.M. |
12 | R1, R2 = (CH2)3 | Ph | – | S.M. | S.M. |
The reaction is sensitive to steric hindrance (compare entries 1, 6, and 8) on the dicarbonyl partner, as well as its acidity (compare entry 1 with the more acidic diketone with entry 11 with the less acidic dimethyl malonate), but not at all to modifications on the nitroalkene. This is consistent with the model presented in Fig. 2, in which the key fact is the deprotonation by the POM surface. Malonates do not work well, as only the more acidic aryl derivatives give some products (entries 7 and 11). It is not clear to us why cyclohexane diketone did not lead to the expected adduct despite its more acidic protons (entry 12).
Finally, we examined how the catalyst could be recycled. Diethyl ether was added to the reaction mixture presented in Table 2, entry 1. This led to the precipitation of 1. After filtration, 1 could be re-used in a new reaction, without significant loss of reactivity. The isolated yield dropped a little (95% vs. 99%). This could be achieved four times in a row, however with a regular (albeit low) decrease in yield (99%, 95%, 91%, 85%, and 83%).
To conclude, we have introduced two squaramide-containing organo-polyoxometalates which efficiently catalyzed the addition of dicarbonyl derivatives onto nitroolefins, and can be recycled. Seen from the POM point of view, this is a new addition to the family of organic reactions opened to POM catalysis and to the growing number of POM-based bifunctional catalytic systems in which the POM surface acts in synergy with the substituents embedded [4b,c] or grafted [6a,f] onto the POM, making the hybrid better than the mere sum of its components. Seen from the organic point of view, this is another evidence that specifically designed organic molecules can generate new applications in POM chemistry via supramolecular interactions. This interaction between organic molecules and POMs in organic solvents has been long overlooked. That is no longer the case. Its further study should lead to other significant advances in POM (organic) chemistry.
Acknowledgments
We thank the “Centre national de la recherche scientifique” (ICSN) for funding, and N. Elie, K. Hammad and J.-F. Gallard (ICSN) for NMR and MS analyses.