1 Introduction
Over the past two decades, an increasing interest in how the drugs are administered to patients was observed, this playing an important role in their effectiveness [1]. Such studies included systems in which the drug delivery degree is controlled by the rate at which the drug is osmotically pumped from a reservoir. Also, for more prolonged drug availability and sustained action, drug release through a membrane or sponge is revealed as a function of the amount of cross-linking agent or it is depending on drug or polymer concentration [2].
Current treatments for diseases and trauma of dental structures are based on carriers used for the local drug-delivery system [3]. Among carriers classified as non-biodegradable or biodegradable, polymeric biodegradable ones have widespread use in drug delivery because they can be degraded to non-toxic monomers inside the body. Several advantages like excellent biocompatibility, non-toxicity, as well as the fact they are well documented in terms of structural, physicochemical, biological, and immunological properties, made collagen the favourable matrix in medicine [4,5].
Previous studies [5–7] have revealed that the biological activities of collagen and its derivatives could be very useful for the treatment of diseases and trauma of dental structures. In many studies, collagen has been treated with glutaraldehyde, the widely used reagent for proteins [8], knowing the chemistry of glutaraldehyde cross-linking and its effect on the biological performance of a number of biomaterials, including drug delivery systems [9,10]. According to Kohlpaintner et al. [11], a 0.1% to 1% glutaraldehyde solution may be used for system disinfection and as a preservative for long-term storage, while 2% glutaraldehyde is the reference disinfectant for hospital instruments [12].
Additionally, different drugs are used for local delivery, including chloramphenicol and some of the most preferred ways by which drugs are loaded into this system based on collagen are hydrogen bonding, covalent bonding, or simple entrapment.
Chloramphenicol, an effective bacteriostatic antimicrobial against a wide variety of Gram-negative and Gram-positive bacteria, including most anaerobic microorganisms, is currently used for ocular and dermal local treatment [13–16]; moreover, due to its broad spectrum of activity, its use in infections and other sites is totally justified. Thus, several studies demonstrating its antibiotic application in gynaecological diseases have been reported (local treatment of bacterial vaginosis) [13,17].
Taking into account these considerations, chloramphenicol was chosen in this study as an antibiotic drug for the treatment and/or the prophylaxis of infected lesions in various dental structures. Its selection is supported by previous studies that have shown that this drug [18] does not produce chromosomal changes after direct administration in the oral cavity [19].
Moreover, there is currently a revival of the use of already consecrated drugs, the older generation, introduced in therapeutics for a long time, whose side effects and adverse reactions are well known. This has the advantage of already knowing the risk/benefit ratio of these drugs with established actions on patients [20–23].
In order to evaluate the in vivo stability of the collagen sponges, the in vitro enzymatic degradation is studied. Degradation of collagen can occur through either extracellular or intracellular collagenases, which are the only enzymes that completely cleave collagen. The rate of collagen digestion can be controlled by changing the degree of cross-linking of the collagen matrix [24].
Because the collagen is the primary resource in biomedical applications, this study was focused on the preparation and the characterization of new types of collagen matrix in sponge form for drug delivery systems. The obtained sponges based on collagen gel, chloramphenicol and different concentrations of glutaraldehyde as cross-linking agent were characterized by spectroscopic studies, water absorption, drug release test, and enzymatic degradation. Also, the new drug delivery sponges were tested for their antimicrobial susceptibility against Gram-positive or Gram-negative bacteria. Particularly, the prepared sponges were tested against Staphylococcus aureus, Streptococcus pneumoniae, and Escherichia coli [25], knowing that these microorganisms are usually found in the oral cavity environment and, on the other hand, these bacteria are important for clinical purpose due to their association with endodontic infections [26,27].
2 Materials and methods
2.1 Materials
For the obtained collagen sponges devoted to biomedical applications, especially as drug delivery systems in dentistry, the following materials were used: a natural polymer-like type-I collagen gel (2.11% dry substance/collagen gel) extracted from bovine skin (The National Research and Development Institute for Textiles and Leather, Bucharest) [28]; a bacteriostatic and antimicrobial drug such as chloramphenicol (CP) purchased from the Sigma Chemical Co., and a cross-linking agent as glutaraldehyde (GA) provided by Merck (Germany). Sodium hydroxide 1 M (NaOH) of analytical grade was used as a solution for pH adjustment.
For enzymatic degradation, test collagenase of Clostridium histolyticum from Sigma-Aldrich (USA) and a phosphate buffer solution (PBS) (pH 7.4) were used.
In order to evaluate the antibacterial activity of the sponges, the following human pathogenic microbial strains were tested: Escherichia coli ATCC 8738, Staphylococcus aureus ATTC 25923 and Enterococcus faecalis ATCC 29212 (facultative anaerobe). The bacterial strains were grown in Luria Bertani Agar (LBA) plates at 37 °C with the following composition: peptone (Merck), 10 g/L; yeast extract (Biolife) 5 g/L, NaCl (Sigma-Aldrich) 5 g/L and agar (Fluka) 20 g/L. The stock culture was maintained at 4 °C.
2.2 Preparation of collagen sponges containing chloramphenicol
Collagen gels prepared as a 1% water solution with a pH adjusted to 7.4 by adding a 1 M NaOH solution were mixed with a water solution of a 1 g/L active substance. After stirring, 1% GA solution is added for cross-linking and let for mixing for 10 min. The obtained homogenous mixture in the form of a gel was placed in a refrigerator for 24 h. The final step in the preparation method was a freeze-drying process using the freeze-dryer Delta 2-24 LSC equipment (Martin Christ, Germany) [29]. The resultant collagen sponges have a concentration of 4% CP relative to the dry substance (0.1% relative to the collagen gel), and a concentration of glutaraldehyde ranging from 0.25, 0.5, 0.75 to 1% related to the dry substance.
2.3 Characterization techniques
Our ATR/FT–IR study was performed using a PerkinElmer Spectrum 100 FT–IR spectrophotometer in attenuated total reflection mode (ATR). The IR spectra were registered between 4000 and 600 cm−1 as a result of the average of four scans with a resolution of 4 cm−1.
The UV–VIS–NIR spectroscopic analysis was realized using a Jasco UV–VIS–NIR spectrophotometer, model V 670 in the 200–2000 nm spectral region, with a step of 0.5 nm.
Water absorption analysis was evaluated to determine the amount of water absorbed under specified conditions [30]. Collagen sponges were firstly weighted (Wd) and then immersed in distilled water at 25 °C for different periods of time until a stable mass was obtained (Ww). Water absorption is expressed as a weight percent increase (Eq. (1)). Each test was performed in triplicate.
(1) |
The enzymatic degradation test was carried out using collagenase of Clostridium histolyticum from Sigma-Aldrich (USA) and a phosphate buffer solution (PBS) of pH 7.4. Each sponge with a known weight was immersed in PBS and incubated at 37 °C overnight. After that, collagenase (10 μg/mL) was added and the test tube was replaced at 37 °C. At imposed intervals (1 h, 2 h, 4 h, 8 h, 24 h, 48 h) the degradation was interrupted by removing the sponges from the degradation solution, squeezing and weighting again the sponge. The percentage weight loss was calculated according to Eq. (2) [30]:
(2) |
Each biodegradation test was made in triplicate.
For antimicrobial activity assays for Gram-positive or Gram-negative bacteria, the reference strains were included as controls and all the tests were performed in triplicate [31]. All collagen sponges evaluated for antimicrobial activity were firstly sterilised with a Vilber–Lourmat equipment.
The bacterial strains were cultured in a tube containing an LB medium, as reported by Ansari et al. [32] at 37 °C.
The antibacterial activities of the samples were determined by calculating the percentage inhibition of growth using the formula proposed by Jaiswal et al. [33]:
(3) |
The sterile samples were incubated for 18 h in test tubes containing 5 mL of a culture of a Gram-negative bacterium, Escherichia coli, of Gram-positive bacteria, Staphylococcus aureus and Enterococcus faecalis, respectively.
The sterile medium was inoculated with bacteria (1%). The optical density was determined after 18 h of incubation in the incubator Laboshake Gerhardt at 200 rpm.
The bacterial growth was determined by measuring the optical density of the samples and the control (bacteria culture without sample) at 600 nm using a Jenway UV–VIS spectrophotometer.
For the determination of the minimum bactericidal concentration (MBC) of chloramphenicol against the tested bacterial strains, each of the tested microorganisms was determined by the broth-dilution technique [34]. Serially diluted logarithmic concentrations of the drug ranging from 400 μg/mL to 0.195 μg/mL were inoculated with standardized overnight cultures of the microorganisms and incubated at 37 °C for 18 h. The MBC (defined as 100% killing) was defined as the lowest concentration of CP that completely inhibited bacteria growth [35].
2.4 Drug release studies
In vitro chloramphenicol release from collagen sponges was carried out using a sandwich device adapted to a paddle dissolution apparatus, as described in our previous studies [20,36]. In brief, each sponge containing CP with known size and weight was introduced into the release vessel where the phosphate buffer of pH 7.4 as a receptor phase was maintained at 37 °C. After each sampling (5 mL) for different periods of time during eight consecutive hours, the same total volume of pre-warmed phosphate buffer solution was restored. The absorbance of the withdrawn solutions was detected by ultraviolet–visible spectrophotometry (PerkinElmer UV-Vis Spectrophotometer). The chloramphenicol amount released from the sponges was determined from the calibration curve obtained in the same buffer at a wavelength of 278 nm (, R2 = 0.9995) [37].
For the evaluation of the drug release kinetics mechanism from spongious systems, the in vitro release data were fitted according to the power-law model:
(4) |
3 Results and discussion
3.1 ATR/FT–IR study
In order to identify the functional groups and to examine the interaction of the natural polymer with a bacteriostatic and antimicrobial drug, ATR/FT–IR spectra were recorded for all raw materials and collagen sponges [36] with and without active substance and cross-linking agent (Figs. 1 and 2).
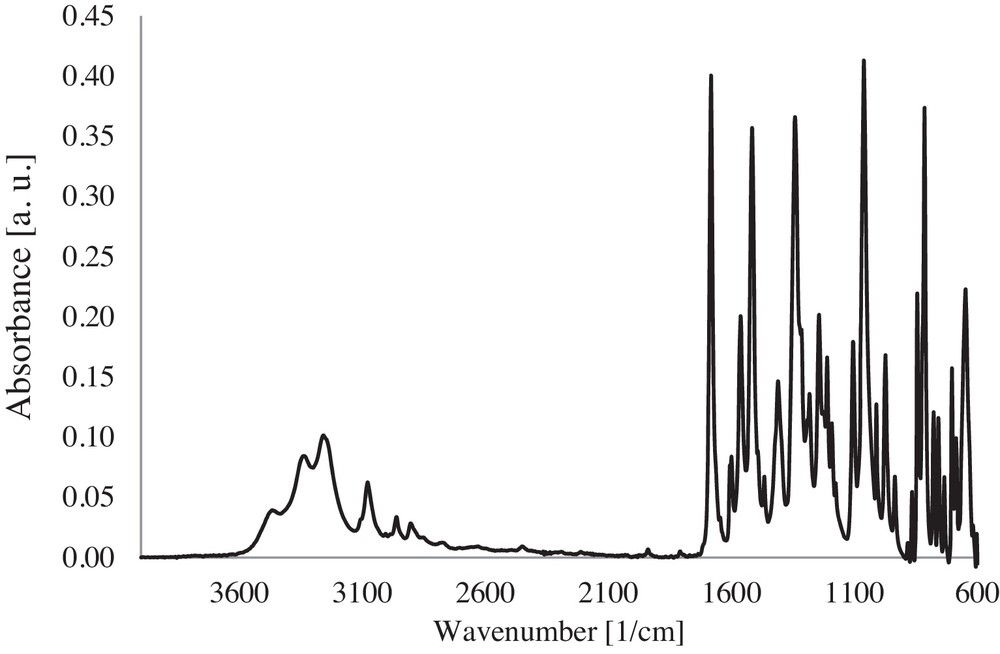
ATR/FT–IR spectrum of the CP powder.
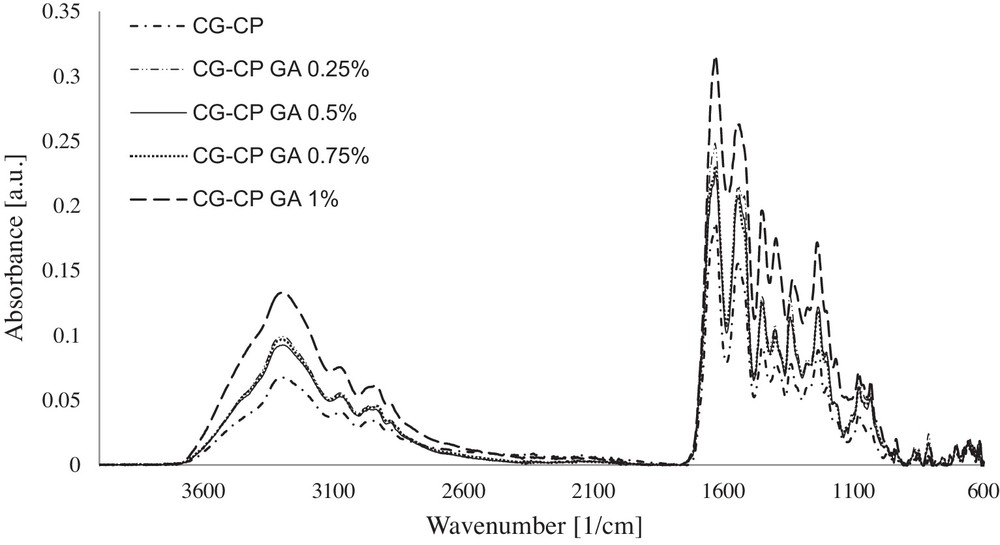
ATR/FT–IR spectra of collagen sponges with CP and different concentrations of cross-linking agent.
The main characteristic absorption bands of CP according to the IR spectrum (Fig. 1) are: a broad band at 3259 cm−1 specific to the stretching vibration of the O–H bond (νOH); a band at 2962 cm−1 corresponding to a stretching antisymmetric vibration of the C–H bond of CH2 (νCH2); an intensive deformation vibration of the N–H bond (δNH) at 1563 cm−1, accompanied by the stretching vibration of the CC bond (νC=C aromatic) at 1516 cm−1 and 1061 cm−1; a band due to the deformation vibration of the O–H bond (δO–H) at 1342 cm−1.
In the ATR/FT–IR spectra, the bands corresponding to the collagen sponge with CP, and also to collagen sponges with CP and different concentrations of GA are assessed as follows:
- • in collagen sponges with CP and GA, the absorption band at 3294 cm−1 attributed to νNHas and to νOHas present in the collagen sponge [36] is found at higher wavenumbers, reaching a maximum at 3302 cm−1 for 0.75% GA. This band shift indicates a contribution of the cross-linking agent and of the chloramphenicol on the growth of the association degree between the collagen and the active substance by hydrogen bonds;
- • in collagen sponges without GA, the amide I band due to νC=O is evidenced. The presence of the active substance in the case of sponges with CP favours a slight shift of the wavenumber to higher values (from 1630 to 1633 cm−1), which indicates the association by hydrogen bonds between collagen and the active substance;
- • the presence of a cross-linking agent (especially for 0.5% and 1% GA) contributes to the increase in the association degree by hydrogen bonds;
- • the amide II band at 1549 cm−1, specific to collagen from collagen sponge, is shifted to lower wavenumbers (1539 cm−1) for the collagen sponge with CP. At low concentrations of GA (0.25% and 0.5%) no shift of the amide II band is evidenced with respect to that obtained for a sponge with CP. However, increasing the GA concentration leads to an increase in the wavenumber of this band to 1548 cm−1 due to N–H bonds that become stronger. This fact shows a positive influence in enhancing the association degree among molecules;
- • in the ATR/FT- IR spectra of collagen sponges with CP, the absorption bands attributed to C–C and to C–N are identified in the region of 1334–1345 cm−1. The new C–N–C bonds formed between the polymeric molecules are detected by a strong absorption band at around 1237 cm−1.
Table 1 collects the data obtained by ATR/FT–IR spectra processing.
Characteristic spectral data for collagen sponge with/without CP and different concentrations of cross-linking agent (GA).
Collagen sponge | AOH/AI | AIII/ACOO– | AI/AOH | Δυ* |
CG | 0.4111 | 1.0017 | 2.4326 | 84 |
CG-CP | 0.3617 | 0.9726 | 2.7648 | 94 |
CG-CP GA 0.25% | 0.3998 | 0.9461 | 2.5015 | 94 |
CG-CP GA 0.5% | 0.4115 | 0.9698 | 2.4304 | 95 |
CG-CP GA 0.75% | 0.4200 | 0.9397 | 2.3808 | 85 |
CG-CP GA 1% | 0.4214 | 0.8747 | 2.3728 | 85 |
By analysing the data from Table 1, the following conclusions are obtained:
- • the presence of the active substance in the collagen composition determines a decrease in the AOH/AI, ratio showing that the hydrolysis degree is diminished. On the contrary, the addition of the cross-linking agent leads to an increase in the hydrolysis degree [39];
- • the structural integrity of the triple helix (AIII/ACOO–) is less affected in sponges with low cross-linking agent content [40];
- • high values of AI/AOH reveal the high degree of cross-linking [41];
- • the denaturation process is not present for Δυ < 100 [42].
3.2 UV–VIS–NIR spectroscopic analysis
UV–VIS–NIR spectra recorded on collagen sponges with bacteriostatic and antimicrobial drug and different concentrations of cross-linking agent are displayed in Fig. 3.
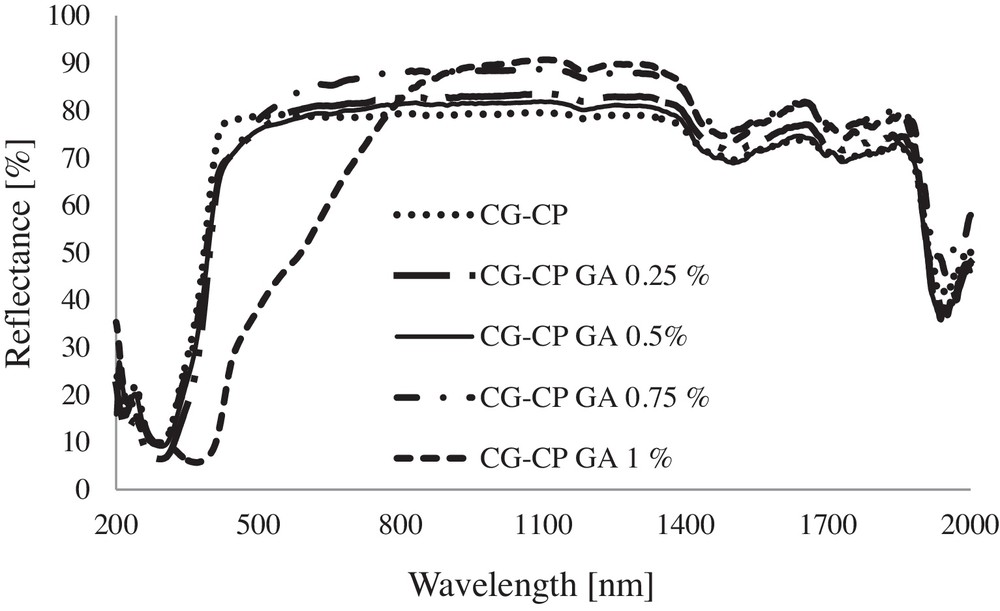
UV–VIS–NIR spectra for collagen sponges with CP and different concentrations of GA.
The main absorption bands evidenced in the UV–VIS–NIR spectra of sponges are: the band due to electronic transition n→π* of the –CO–NH– group of the amide structure of collagen, found in the 276–295 nm domain; the band assigned to CH2 at around 1183 nm; an intense peak attributable to νOHassociated evidenced at 1502 nm; a maximum absorption peak specific to δO–H registered at 1951 ± 6 nm showing the contribution of each compound.
3.3 Water absorption
The effect of the content of cross-linking agent on the water absorption capability of the sponges was investigated. Fig. 4 shows the results obtained for water absorption on collagen sponges with different compositions at room temperature.
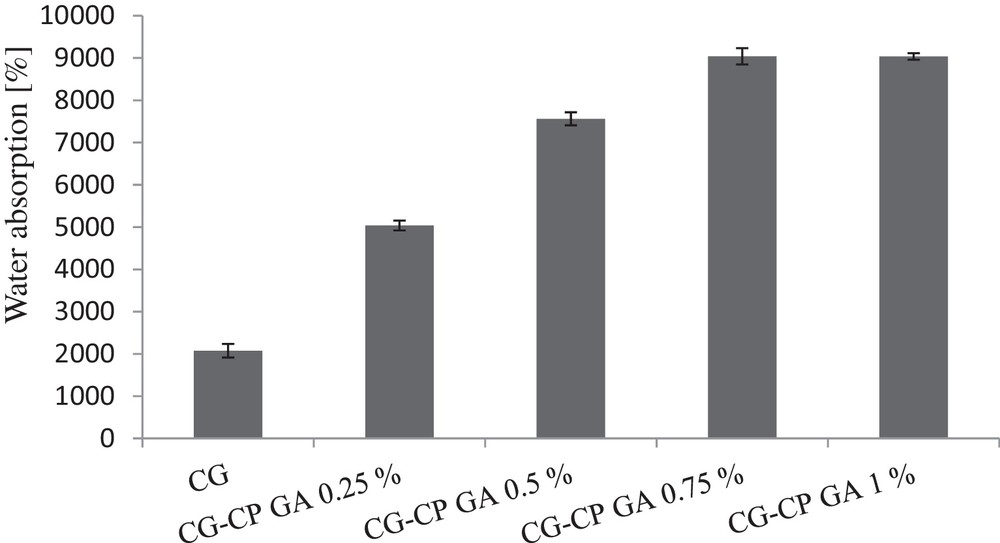
Water absorption on collagen sponges with different compositions.
A significant difference was observed between collagen sponges without GA and no CP content and those containing both drug and cross-linking agent. After changing the composition while keeping constant the CG content, and adding the same amount of CP and different amounts of GA, water absorption was modified. As expected, a direct relationship between the increase in the cross-linking agent content and that of water absorption capability was found. After they have absorbed water, collagen sponges expanded, and returned to their original shape after squeezing water.
3.4 Enzymatic degradation test
The biological stability of collagen sponges was evaluated by in vitro collagenase degradation. After digestion with collagenase solution, the weight loss of the collagen sponges is shown in Fig. 5. Comparing the sponges based on CG and CP with and without GA, different enzymatic degradation rates and different weight loss percents were obtained as a function of the cross-linking agent content. The collagen sponge with CP, but without GA, shows a complete enzymatic degradation after only 1 h of exposure to collagenase, while collagen sponges with both CP and GA show a total digestion after 4 h, 8 h, and 48 h, depending on the GA amount. Therefore, a higher content of the cross-linking agent (0.75% and 1% GA) leads to an increase in the resistance to degradation by up to 48 h. At the same time, the presence of GA in the smallest amount (0.25% and 0.5% GA) favours a slower weight loss than in sponges with high cross-linking agent content, proving a better behaviour regarding collagenase digestion.
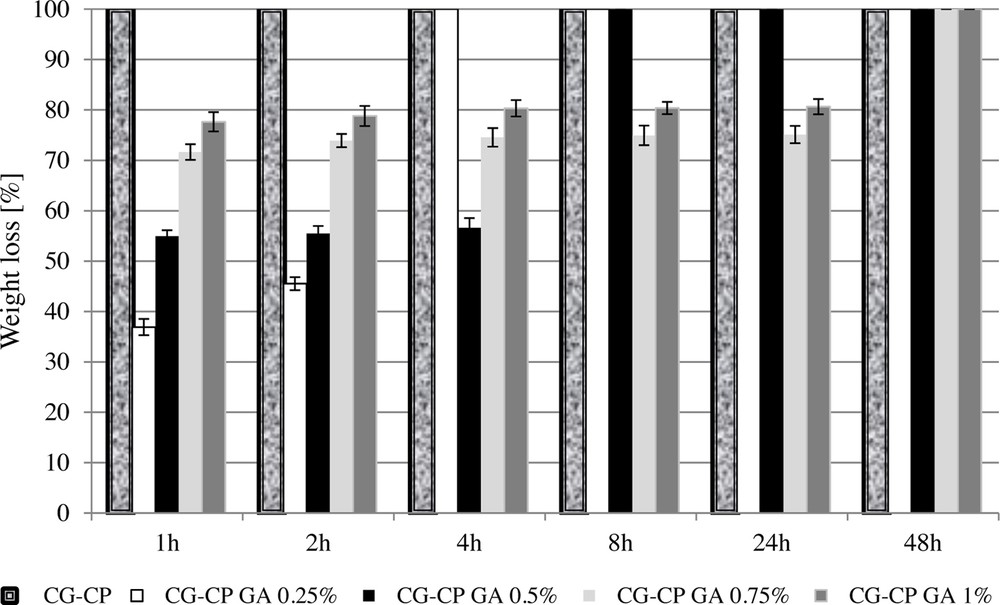
Weight loss versus time for collagen sponges after digestion with a 0.1% collagenase solution.
These results indicate that all sponges cross-linked with GA were more stable than untreated ones.
3.5 Antimicrobial susceptibility
The results obtained for the antimicrobial susceptibility test of CP on the chosen organisms showed MBCs (minimum bactericidal concentration) of 1.56, 0.39 and 6.25 μg/mL against Staphylococcus aureus, Escherichia coli, and Enterococcus faecalis, respectively (Table 2).
Antimicrobial susceptibility of the microorganisms to chloramphenicol.
Microorganism | Concentration of chloramphenicol used (μg/mL) | |||||||||||
400 | 200 | 100 | 50 | 25 | 12.5 | 6.25 | 3.125 | 1.56 | 0.78 | 0.39 | 0.195 | |
Escherichia coli | S* | S | S | S | S | S | S | S | S | S | S | R* |
Staphylococcus aureus | S | S | S | S | S | S | S | S | S | R | R | R |
Enterococcus faecalis | S | S | S | S | S | S | S | R | R | R | R | R |
Antimicrobial susceptibility was determined on three different days, and MBC values for each isolate were reported as the average of three experiments.
The aim of endodontic treatment is to eradicate the infection and to prevent infecting or re-infecting the root and periradicular tissues.
The presence of Enterococcus faecalis and Staphylococcus aureus in root canals has been reported in therapy-resistant periapical periodontitis [43] and Enterococcus faecalis has been frequently found in root-canal-treated teeth in values ranging from 30 to 90% of the cases [44].
Root-canal-treated teeth are about nine times more likely to harbour E. faecalis than in cases of primary infections [45].
Escherichia coli is not commonly found in root canals with necrotic pulp, but some studies found this bacterium in root canals with periapical lesions [46,47].
The bacteriological experiments performed in vitro demonstrated the effectiveness of all new drug delivery sponges treated with CP in inhibiting the growth of Escherichia coli, Enterococcus faecalis, and Staphylococcus aureus bacteria (Fig. 6).
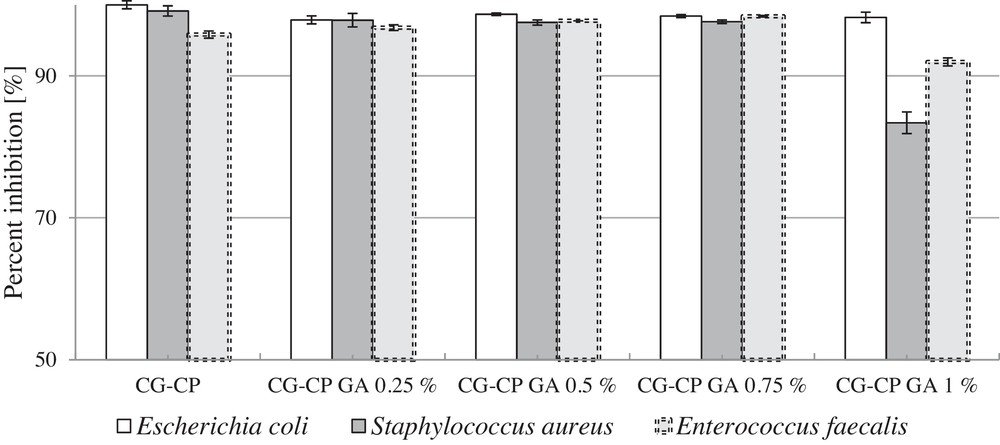
Percentage inhibition of bacteria growth.
The evaluation of the antibacterial activity (microbicidy) of the samples was made by calculating the percentage inhibition of growth using Eq. (3).
The results showed that the new drug delivery sponges exhibit an antibacterial activity against Gram-positive and Gram-negative bacteria, while different concentrations of GA do not influence significantly the antimicrobial activity.
3.6 Kinetic study on drug release
The cumulative amount of chloramphenicol released in terms of released drug percent was plotted against time and the kinetic profiles of the swellable systems un- and cross-linked with glutaraldehyde are presented in Fig. 7.
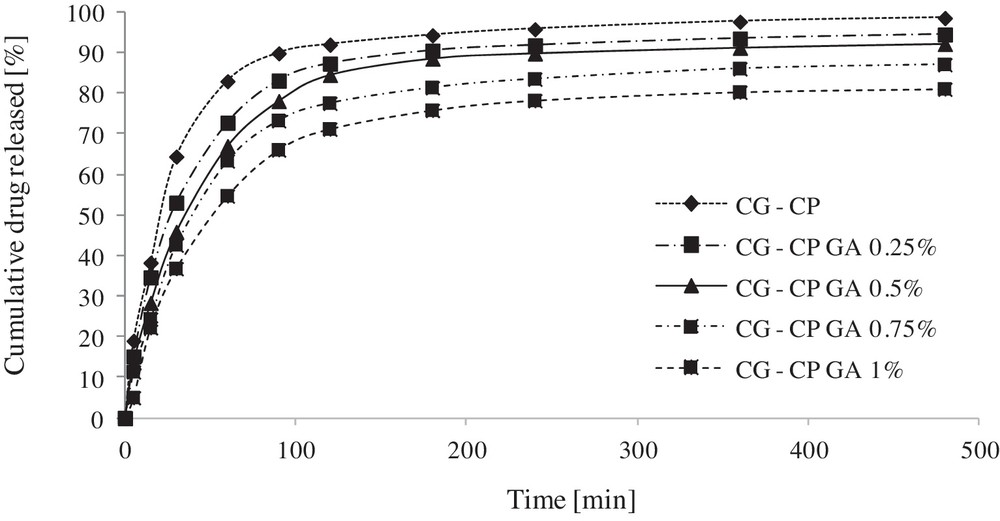
Cumulative release profiles of chloramphenicol from collagen sponges as a function of time.
As can be noticed in Fig. 7, the kinetic profiles are directly influenced by GA concentration. A higher amount of GA in the formulation induces a lower percentage of released drug.
The CP release from the designed systems follows a typical release in two steps, as described in our previous studies [20]: initially, in the first 60–90 minutes, a rapid drug release is recorded, followed by a slow release during approximately the seven next hours. The burst release phase is more evident for a low crosslinking degree (CG-CP, CG-CP GA 0.25%, CG-CP GA 0.50%). After 8 h of experiment, the cumulative percents released are 98.55%, 94.50%, 92.15%, 87.06%, and 81.02%, respectively. Such tow-stage release is adequate for antibiotic formulations as the initial rapid action is followed by a slower sustained release.
The kinetic data were checked by the power-law model. The obtained values of the correlation coefficient (R), of the release exponent (n), of the kinetic parameters, and of the drug percent release data are presented in Table 3.
Power-law model parameters obtained for the investigated systems (sponges).
Collagen sponge | R value for the power-law model | Release exponent | Kinetic constant (1/minn) | Percent released (%) |
CG-CP | 0.9453 | 0.226 | 0.275 | 98.55 |
CG-CP GA 0.25% | 0.9522 | 0.253 | 0.225 | 94.50 |
CG-CP GA 0.5% | 0.9531 | 0.277 | 0.189 | 92.15 |
CG-CP GA 0.75% | 0.9529 | 0.286 | 0.168 | 87.06 |
CG-CP GA 1% | 0.9515 | 0.311 | 0.136 | 81.02 |
It can be seen from the values of the kinetic constant that the release rate is decreasing by increasing the GA content.
Conclusions
In this study, new collagen sponges were developed by the freeze-drying method and characterized by different techniques. All collagen sponges favour water absorption, and, moreover, the absorbability of the sponge depends on its cross-linking agent content. Water absorption is increasing by increasing the GA content by the same amount of bacteriostatic and antimicrobial drug. In terms of resistance to collagenase degradation, chemical cross-linking with more than 0.5% GA provided a good protection for sponges in time. It was concluded that the new collagen sponges demonstrated a strong activity against all tested Gram-positive and Gram-negative bacteria. Chloramphenicol release from collagen sponges can be closely correlated with the physicochemical and enzymatic degradation characteristics, as a result of a porous chemical architecture.
Overall, this investigation indicated that the materials synthetized promise to be developed as new antibacterial sponges for the possible treatment of diseases and trauma of dental structures including bacterial infections. In the near future, these new collagen sponges are expected to become useful and safe, and to be promising matrices for drug release systems in dental applications.