1 Introduction
Extraction of compounds from natural solid matrices can be achieved by means of several techniques, such as hydrodistillation, maceration, low pressure solvent extraction and others. However, environmental restrictions, public health regulations and consumer demands have boosted the development of clean technologies. In addition, the presence of thermolabile constituents requires the use of techniques at low temperatures to avoid any hydrolysis or thermal degradation.
Supercritical fluid extraction using carbon dioxide is a technique which can be used to obtain volatile extracts from vegetable matrices without any trace of solvent [1]. Carbon dioxide is the mostly used supercritical fluid, particularly for the extraction of pharmaceutical and food compounds due to its chemical and physical properties and to its relatively low critical temperature and pressure values (304 K and 7.38 MPa, respectively) [2].
Extracts obtained by means of supercritical fluid extraction are of a much better quality than those obtained by solvent extraction with organic solvents or by steam and hydrodistillation. Also these classical techniques have some clear disadvantages like the difficulty in recovering all the organic solvent from the final product [3,4] in the case of solvent extraction and the high temperatures involved which may cause thermal degradation and partial hydrolysis of some constituents in the case of steam and hydrodistillation [5,6]. These techniques also require further unit operations, such as decantation, centrifugation and others [7], hence inducing further costs.
Supercritical fluid extraction is an important clean technology where the high solvent power of supercritical fluids is due to the influence of pressure and temperature on their properties, mainly their density [8]. However one of the major drawbacks of this technique is the equipment cost which limits its use in highly sensitive industrial fields where high qualities and purities of the final products are required [7].
Rosemary (Rosmarinus officinalis L.) is a widely cultivated plant in most of the Mediterranean regions like North Africa and northern and central Europe, northern Asia and America. It simply grows in climate with a yearly average rainfall between 200 and 600 mm. Morphologically it is a tree of up to 1.5 m height, always green, straight, with leaves and an aroma odor. Rosemary is relatively rich in essential oil which can be extracted from the flower itself or from the leaves where the essential oil glands are located. However the highest essential oil quality is obtained from the latter i.e. the leaves. It can be used for various applications such as an aroma in food. Rosemary (R. officinalis) belongs to the Laminaceae family and is used generally as a condiment, but its important antioxidant activity has also been shown [2]. Generally it contains camphor and 1.8 cineol as the major compounds but other compounds, such as carnosic acid, Rosmarinus acid, α-pinene, camphene, β-pinene, myrcene, limonene and borneol are also present but in much smaller proportions [2–4]. It has also interesting antiseptic and good antioxidant properties [9] and hence can be a very efficient bactericide for food conservation.
The aim of the present work is to apply the response surface methodology (RSM) in order to investigate and optimize the effects of pressure and temperature on the yield of extraction of Algerian rosemary oil (R. officinalis L.) by supercritical carbon dioxide. The extracted oil under optimal extraction conditions was analyzed by Gas chromatography-Mass Spectrometry.
2 Materials and methods
2.1 Plant material preparation
Rosemary was sampled from the last October local production (in Constantine, North east of Algeria). As a first step, dried rosemary leaves were ground by using a small coffee grinder for a sufficiently short period of time to get a uniform particle size distribution with a mean diameter of 1 mm. The obtained charge was sieved using a Retisch type vibrating system. The percentage of water content expressed as the ratio of the mass of humid material – mass of the dried material over the humid material – was determined to be equal to 5.92 wt%, by drying rosemary leaves in a vacuum oven at 378 K up to a constant mass.
The bulk density of the ground rosemary determined by means of a Helium pycnometer was 335.4 kg/m3.
2.2 Supercritical CO2 extraction
Referring to Fig. 1, the experiments were carried out in the dynamic extraction unit which mainly consists of three parts: 1- a CO2 reservoir; 2- an extractor vessel, and 3- three separator vessels in series, accompanied by a thermostatic bath, a metering pump, a cold exchanger, the necessary instrumentation to control the pressure, temperature, mass flow rate and valves for the extract collection. A mass of 20 g was weighed in a precise scale instrument balance and then packed into a sample unit, with glass wool placed at its top and bottom in order to prevent the entrainment of rosemary during the extraction process. The sample was then allowed to reach the constant extraction pressure and temperature. After 1 h, a time corresponding to static extraction, the extractor valve was opened and the intermediate valves between the separators were continuously adjusted in order to regulate the pressure and, hence keep a constant flow rate of 0.42 kg h−1. The samples were taken every 15 min and the dynamic extraction was pursued for 3.5 h. However it was noted that the extracted mass was very low.
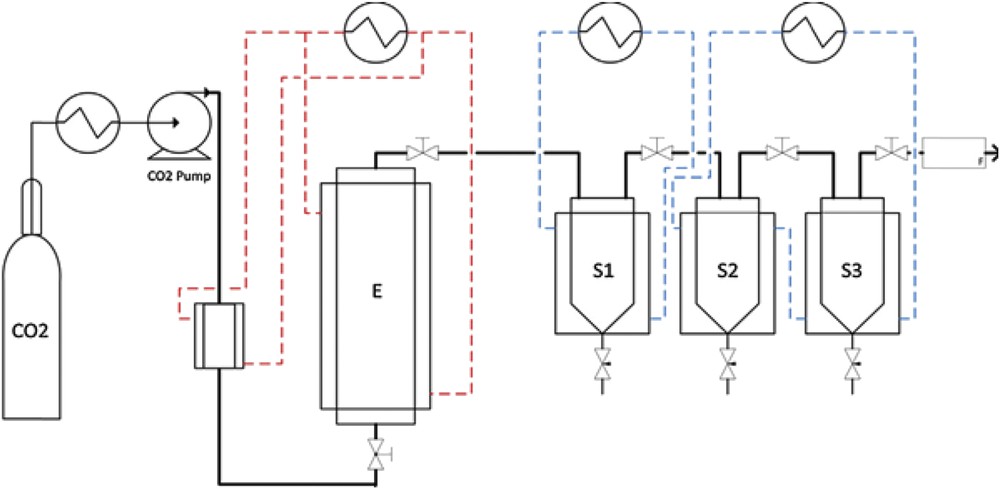
Supercritical fluid extraction unit, E: extractor; S1, S2, S3: separators.
2.3 Experimental design
An experimental design was applied for determining extraction temperature (X1) and pressure (X2) to optimize the yield (Y) of a supercritical CO2 extraction of essential oil from rosemary plant leaves.
For each investigated factor, three levels (two values at the extremity and one in the center) were tested and a full plan composed of 32 experiments was performed, as shown in Table 1.
Codes and levels of independent variables used in the surface methodology(RSM) design.
Symbol | Independent variables | Coded levels | ||
Low(−1) | Middle (0) | High (+1) | ||
X1 | Temperature (K) | 313 | 323 | 333 |
X2 | Pressure (MPa) | 14 | 18 | 22 |
The experimental yields were fitted with a second-order response surface model expressed by the following polynomial equation (Eq. 1):
(1) |
2.4 Gas chromatography-Mass spectrometry analysis
The composition of the extracted oil was obtained by means of GC–MS analysis using a gas chromatograph (Shimadzu GC-2010) coupled to a mass spectrophotometer (Shimadzu MS QP-2010), equipped with an autoinjector AOC-20i series, and using a column AT-5ms (30 m, 0.25 mm, 0.25 μm). The column temperature was initially set at 60 °C (held 1 min), then increased up to 250 °C at a rate of 2 °C/min (held 5min). The mass spectrometer was operated with an injected volume of 2 μl and Helium as the carrier gas at an inlet pressure of 37.1 kPa, a velocity of 32.4 cm/s and ionization energy of 70 eV. The identification of oil components was based on matching their recorded retention indices and mass spectra with those in NIST (National Institute of Standards and Technology) general library (Standard reference Data Program Gaithersburg, MD 20899).
The results of such analysis carried out under the obtained optimum conditions T = 313K and P = 22 MPa are presented in Table 2 as well as by the corresponding chromatogram of Fig. 2 where it can be seen, as mentioned in the introduction, that most of the main oil primary components such as 1,8-cineole, a-pinene and camphor, are present in good proportions and are specific contributors to the rosemary flavor.
Composition of the essential oil extracted from local rosemary leaves.
Component | Retention time (min) | Percentage (%) | |
1 | α-Pinene | 6.435 | 6.05 |
2 | Camphene | 6.765 | 7.55 |
3 | β-Pinene | 7.845 | 0.51 |
4 | Myrcene | 8.355 | 0.31 |
5 | α-Phellandrene | 8.620 | 0.26 |
6 | α-Terpinene | 8.955 | 0.18 |
7 | p-Cymene | 9.820 | 0.42 |
8 | 1,8-Cineole | 10.593 | 9.65 |
9 | γ-Terpinene | 11.834 | 0.08 |
10 | Camphor | 16.379 | 52.12 |
11 | Borneol | 17.645 | 3.52 |
12 | α-Terpinol | 18.286 | 1.78 |
13 | Verbenone | 19.498 | 1.97 |
14 | Bornylacetate | 24.795 | 0.42 |
15 | Copaene | 30.342 | 1.07 |
16 | Aromadendrene | 33.186 | 2.11 |
17 | β-Caryophyllene | 36.252 | 0.79 |
18 | α-Caryophyllene | 39.876 | 1.71 |
19 | α-Bisabolol | 49.794 | 0.97 |
Total | 91.47 |
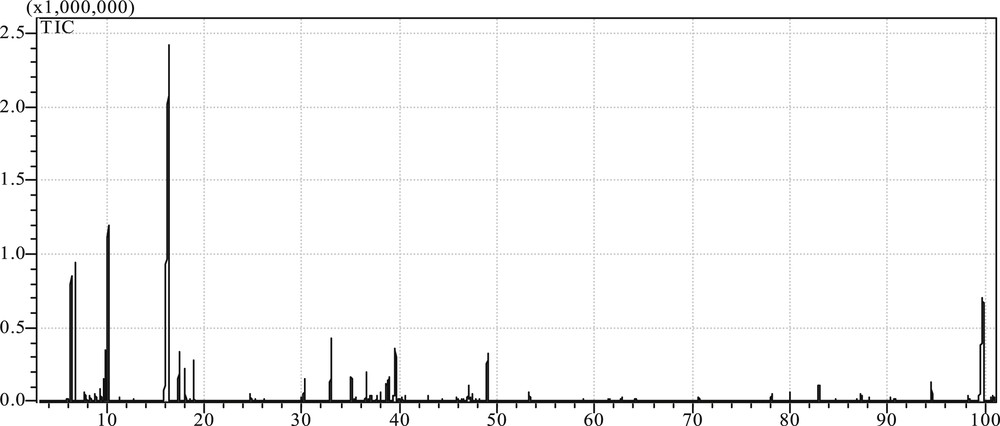
Chromatogram of rosemary oil by GCMS.
From the literature these components do generally influence the properties of many different essential oils, although secondary components are also present and are mainly terpinen-4-ol, α-terpineol, β-caryophyllene, 3-octanol, geranyl acetate and linalyl acetate [4]. However, the most active components are the phenolic diterpenes such as carnosic acid, rosmanol, isorosmanol, rosmadial, etc. [5].
It is also important to note that differences in the composition and properties of different oils extracted from the same plant may also result from various factors like rainfall, climate and soil quality. For instance, essential oil from Moroccan rosemary showed important proportions of α-pinene (37.0–40.0%), cineole (58.7–63.7%) and camphor (41.7–53.8%) [10]. Essential oil from Tunisian rosemary was rather rich in cineole and contained usual monoterpenes [11]. Also GC-MS analyses of supercritical fractional extracts from Italian [5] and Spanish rosemary leaves showed mainly the same major compounds as the Algerian essential oil but with additional traces of other minor constituents [12].
3 Results and discussion
3.1 Response surface methodology analysis
The oil extraction yield was simply calculated as the ratio of the extracted oil mass per 100 g of dry vegetal material, whereas the CO2 density at given temperature and pressure was obtained by means of DIAGSIM a physical proprieties estimation program [13].
The response values (oil yield) for different temperature and pressure combinations are given in Table 3 which shows considerable variations in oil yield depending on the extraction conditions.
Experimental extraction yield.
Run | T | P | Yield exp (%) |
1 | 313 | 14 | 2.020 |
2 | 313 | 18 | 3.040 |
3 | 313 | 22 | 3.520 |
4 | 323 | 14 | 1.380 |
5 | 323 | 18 | 2.810 |
6 | 323 | 22 | 2.940 |
7 | 333 | 14 | 0.950 |
8 | 333 | 18 | 2.570 |
9 | 333 | 22 | 2.690 |
For all the tested pressures, an increase in temperature led, as expected, to a decrease in oil recovery. At a fixed pressure an increase of temperature led to a decrease of the fluid density, hence a decrease of the solubility of the extracted compounds and a less important oil recovery [2,14]. Thus, concerning the effects of these two parameters, i.e. pressure and temperature, it should be noted that the oil solubility is controlled by a balance between the solvent density and the solute vapor pressure, as reported in [15].
The experimental oil yields were used to determine the β coefficients of the response surface (Eq. (1)) and the results are shown in the following table (see Table 4):
Regression coefficients of the polynomial response surface equation.
Coefficient | Value | Standard error | Significance α(%) |
β0 | 2.748 | 0.127 | 0.021c |
β1 | −0.395 | 0.069 | 1.080a |
β2 | 0.800 | 0.069 | 0.140b |
β11 | 0.088 | 0.120 | 51.600 |
β22 | −0.557 | 0.120 | 1.900a |
β12 | 0.060 | 0.085 | 53.100 |
a α < 0.05.
b α < 0.01.
c α < 0.001.
The second-order polynomial equation expressing the total extraction yield as a function of independent variables is as follows:
(2) |
Fig. 3a illustrates the response surface representing the influence of temperature and pressure on the yield of rosemary oil. It can be seen that whatever the temperature, an increase in pressure from 180 to 220 bar led to an increase in oil recovery. This can be explained by the high solvency acquired by the fluid with the increase of the density. However a pressure increase induced a diffusivity decrease of the fluid into the solid pores and may reduce the solute solubility. Also the solubility of solutes decreased when the temperature increased. This means that the experimental conditions of pressure studied corresponded to the retrograde zone where indeed, the solubilities of solutes decreased when the temperature increased [2]. The results also confirm the general trend reported in the literature [2,14] where for a given pressure, the best extraction yield is obtained at low temperatures as clearly shown by the two-dimensional plot in Fig. 3b.
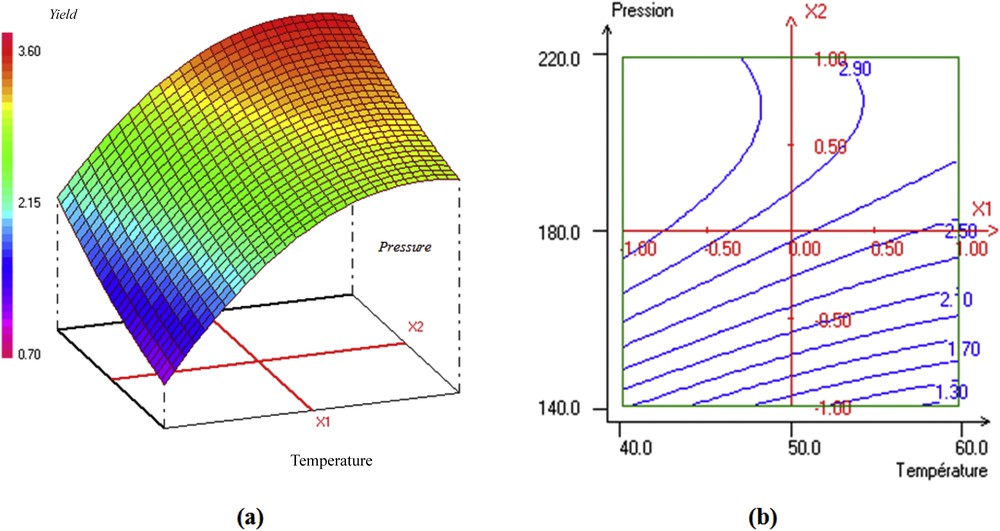
The 3D response surface and 2D contour plots of the oil yield as related to temperature and pressure.
However calculations of the statistical significance percentage α of each regression coefficient showed that the linear terms of pressure and temperature had a significant effect on the oil extraction yield, followed by the quadratic term of pressure, contrarily to the quadratic term of temperature and interactions between temperature and pressure which were not statistically significant (51.6 and 53.1%, respectively). Therefore the approximation can safely be expressed as follows:
(3) |
Sample calculations showed that the effects of the discarded quadratic term of temperature and the interaction contribution are less than the experimental error.
Table 5 also shows a comparison between the experimental extraction yield values and the calculated ones by means of the surface response method, as well as the deviations which vary from 0.011 to 0.234%, indicating satisfactory results.
Calculated and experimental oil extraction yields.
Run | T | P | Yield cal (%) | Yield exp (%) | Deviation (%) |
1 | 313 | 14 | 1.786 | 2.020 | 0.234 |
2 | 313 | 18 | 3.143 | 3.040 | 0.103 |
3 | 313 | 22 | 3.386 | 3.520 | 0.134 |
4 | 323 | 14 | 1.391 | 1.380 | 0.011 |
5 | 323 | 18 | 2.748 | 2.810 | 0.062 |
6 | 323 | 22 | 2.991 | 2.940 | 0.051 |
7 | 333 | 14 | 0.996 | 0.950 | 0.046 |
8 | 333 | 18 | 2.353 | 2.570 | 0.217 |
9 | 333 | 22 | 2.596 | 2.690 | 0.094 |
The plot in Fig. 4 shows an excellent agreement between the experimental and calculated values for rosemary oil yield with a correlation factor very close to unity.
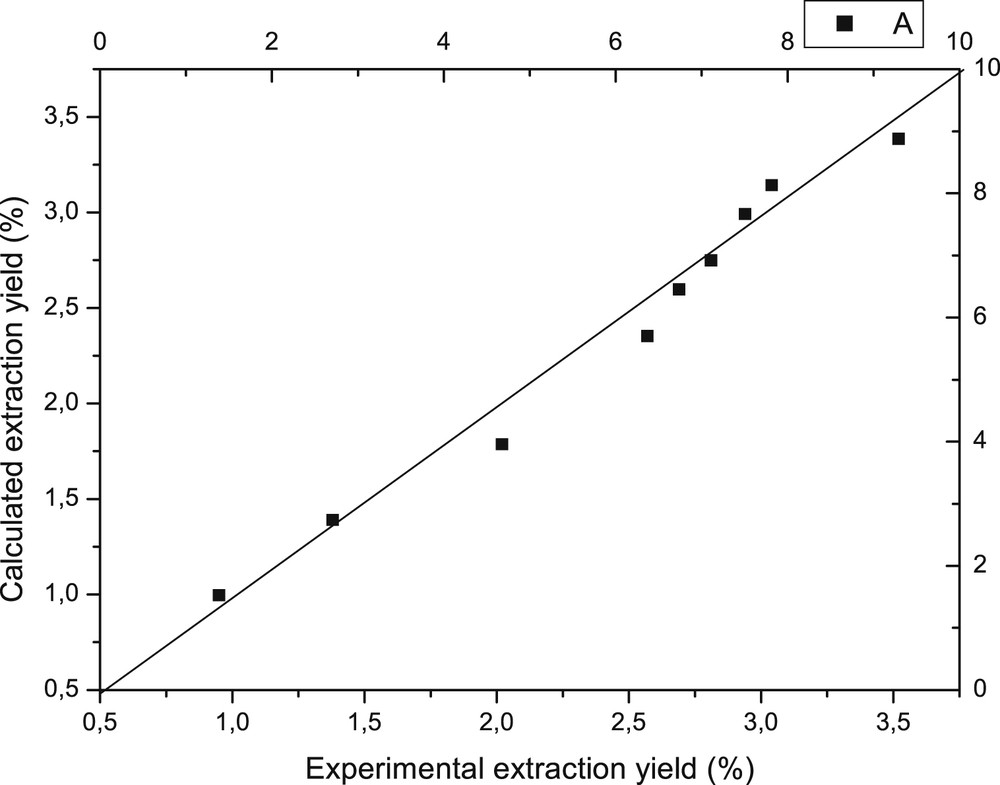
Comparison between calculated and experimental yields.
3.2 Experimental extraction curves
Fig. 5 shows the obtained extraction curves which represent the variation of the cumulated recovered mass of essential oil with time under different operating conditions of temperature and pressure, a particle mean diameter, Dp = 1 mm, a CO2 mass flow rate kept constant and equal to 0.42 kg h−1 and an extraction time of 3.5 h. The shapes of these extraction curves indicate that the extraction is limited by the solubility of solutes in SC–CO2 and show two main distinct parts. The first one corresponds to the great availability of essential oil at the solid surface and hence its extraction by the supercritical fluid at a fast and constant rate, and this under all considered conditions. For this step, the extraction process is controlled by the external mass transfer resistance. The second part of the curve can be explained by the fact that the superficial oil gets exhausted and then oil is extracted from deeper sections of the solid substrate by the solvent. At this point, diffusional and internal mass transfer resistances dominate the extraction process.
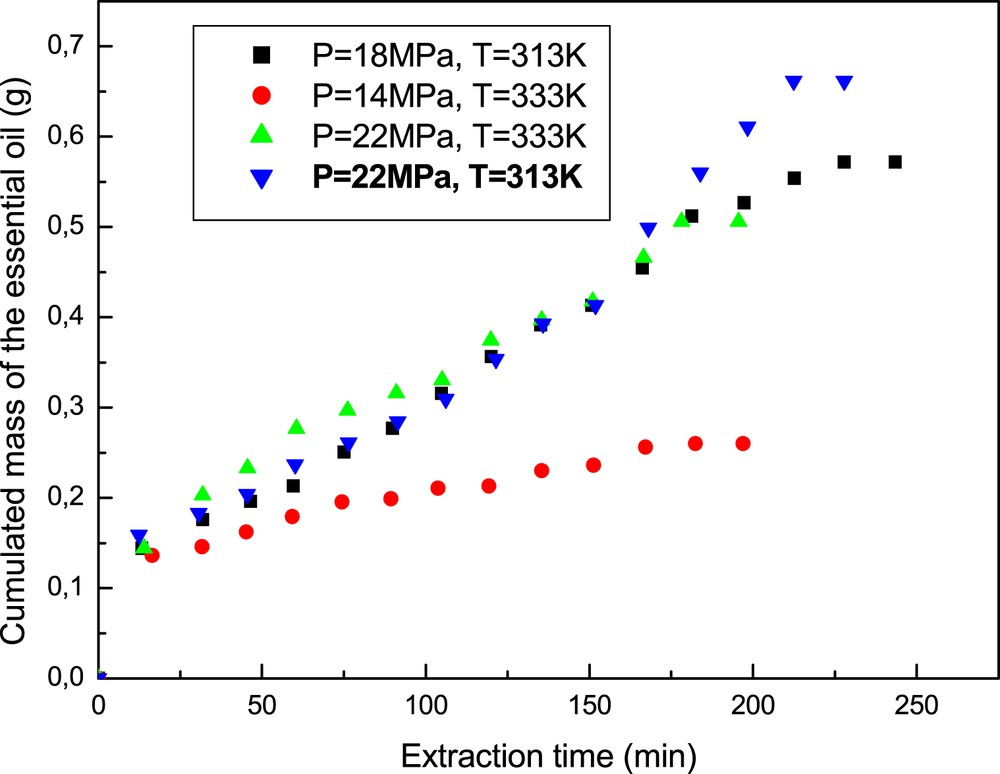
Experimental extraction curves under different conditions.
4 Conclusion
In the present study, supercritical fluid extraction from rosemary leaves was performed at temperature and pressure ranges of (313–333 K), (14–22 MPa) respectively, and a solvent flow rate of 0.42 kg/h (particle diameter of 1 mm) for 3.5 h extraction time. The oil extraction yield was optimized by RSM considering temperature and pressure as independent variables and a second-order polynomial model was used to describe and predict the response variable of the rosemary oil yield obtained by supercritical CO2 extraction within the experimental ranges. A good agreement between the experimental and predicted rosemary oil yield values was observed with (R2 = 0.99). The experimental results showed that maximum oil recovery was 3.52 wt% (relative to the initial mass of dry biomass) obtained at 313 K, 22 MPa.
Consequently, we conclude that the response surface methodology is a technique which can give a large amount of information from a small number of experiments and the interaction effects of the independent parameters can be observed on the response [16].
The chemical composition of the Algerian rosemary oil, determined by GC-MS analysis under optimal conditions, revealed the presence of camphor as the major compound (52.12%), 1,8-cineole (9.65%), camphene (7.55%), α-pinene (6.05%), borneol (3.52%), aromadendrene (2.11%), verbenone (1.97%), and α-caryophyllene (1.71%).