1 Introduction
During the recent years multi-component reactions (MCRs) have received great attention due to their wide range of applications in pharmaceutical chemistry for the construction of diversified structural scaffolds [1], synthesis of natural products [2], and combinatorial chemistry [3]. Compared to the classical stepwise reactions, MCRs offer significant benefits such as high atom economy, straightforward experimental procedures, high overall chemical yields, short reaction times and avoidance of costly and energy consuming intermediate separation and purification steps which minimize waste generation, making the chemical transformations environmentally-benign [4–7].
Pyrrole and its derivatives have attracted considerable attention because of their presence in a variety of natural products [8], biologically active compounds [9], and drugs [10]. Various pyrrole derivatives have been shown to possess interesting biological properties such as antibacterial [11], antiviral [12], anti-inflammatory [13], antifungal [14], antioxidant [15] and anticancer [16] activities. Furthermore, these compounds are widely used in materials science [17], as organic conducting materials [18] and as key intermediates for the synthesis of polymers [19], porphyrins [20], dyes [21a], and functionalized materials [22]. Due to their importance and widespread applications, several synthetic routes such as Hantzsch [23], Knorr [16,24], Paal-Knorr [25], and Clauson–Kaas [26] reactions, transition metal-catalyzed cyclizations [27], and multi-component reactions [28] have been reported for the synthesis of pyrroles. However, many of the reported methods suffer from one or more drawbacks including unsatisfactory yields, long reaction times, the use of toxic organic solvents, expensive and moisture sensitive catalysts, and cumbersome experimental procedures. Thus, the development of an alternative method for the synthesis of pyrroles by using a readily available, environmentally friendly and cheap catalyst under mild conditions is still highly desired.
The application of Keggin-type heteropoly acids as environmentally friendly and green homogeneous or heterogeneous catalysts has been recognized and established because of their non-corrosive nature, low cost, safety, low quantity of waste, stability, low toxicity, recoverability, and reusability. Heteropoly acids, especially H3PW12O40, have gained importance owing to their stronger acidity, higher thermal stability and selectivity properties. Therefore, a wide variety of synthetically valuable organic transformations using these solid acid catalysts have been reported in the literature [29].
As a part of our ongoing efforts on the development of environmentally-benign synthetic transformations using heteropoly compounds [30], herein we would like to report a convenient one-pot multi-component method for the synthesis of polysubstituted pyrroles, symmetrical and unsymmetrical bis-pyrroles using H3PW12O40 as a commercially available catalyst under solvent-free conditions at room temperature (Scheme 1).
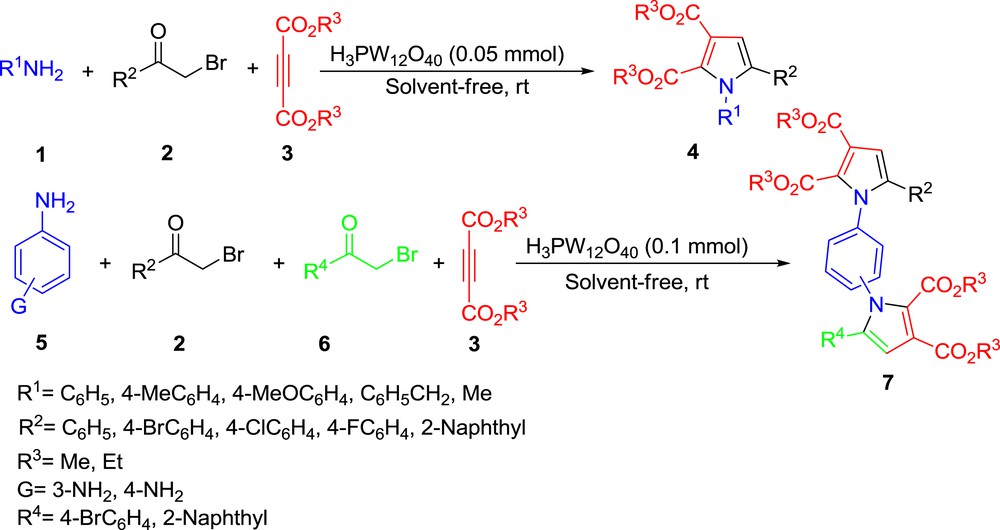
Synthesis of polysubstituted pyrroles and bis-pyrroles catalyzed by H3PW12O40.
2 Results and discussion
Initially, we attempted the three–component reaction between aniline (1 mmol), α-bromo acetophenone (1 mmol) and dimethyl acetylenedicarboxylate (1 mmol) in the absence of the catalyst under solvent-free conditions at room temperature. No reaction was observed even after 10 h (Table 1, entry 1). Then, the reaction was performed in the presence of various Lewis acids such as ZnCl2, MgCl2, MnCl2, BiCl3, Bi(OTf)3 and heteropoly acids such as H3PMo12O40, H4SiW12O40 and H3PW12O40 (Table 1, entries 1–9). Of these, H3PW12O40 was found to be the most effective catalyst to carry out this reaction. Finally, the same reaction was carried out using various amounts of H3PW12O40 (0.03, 0.05 and 0.07 mol%) (Table 1, entries 9–11); the best yield of the product was obtained using 0.05 mol% of the catalyst (Table 1, entry 9). It was noted that increasing the amount of the catalyst from 0.05 mol% to 0.07 mol% did not alter either the reaction time or the yield of the desired product.
Optimization of reaction conditions for the synthesis of polysubstituted pyrroles.a
Entry | Catalyst (mmol) | Yield (%)b |
1 | No catalyst | – |
2 | ZnCl2 (0.05) | 40 |
3 | MgCl2 (0.05) | 22 |
4 | MnCl2 (0.05) | 20 |
5 | BiCl3 (0.05) | 40 |
6 | Bi(OTf)3 (0.05) | 47 |
7 | H3PMo12O40 (0.05) | 40 |
8 | H4SiW12O40 (0.05) | 63 |
9 | H3PW12O40 (0.05) | 94 |
10 | H3PW12O40 (0.03) | 60 |
11 | H3PW12O40 (0.07) | 94 |
a Reaction conditions: aniline (1 mmol), α-bromo acetophenone (1 mmol), dimethyl acetylenedicarboxylate (1 mmol), catalyst (0.05 mmol), at rt for 1 h.
b Isolated yield.
In order to expand the generality of the present method, the synthesis of a wide range of pyrroles was examined under the optimized conditions and the results are summarized in Table 2. A variety of aromatic amines were reacted smoothly with various α-bromo ketones and dialkyl acetylenedicarboxylate in the presence of H3PW12O40 at room temperature to afford the desired polysubstituted pyrroles in excellent yields (Table 2, entries 1–10). Aliphatic amines such as benzyl amine and methyl amine were also reacted efficiently to give the corresponding polysubstituted pyrroles in high yields (Table 2, entries 11, 12).
Synthesis of polysubstituted pyrroles catalyzed by H3PW12O40.a
Encouraged by the excellent results obtained for polysubstituted pyrroles, the potential of this catalytic protocol was further explored for the synthesis of bis-pyrroles and the results are given in Table 3. The reaction of diamines such as 1,4-phenylenediamine and 1,3-phenylenediamine with α-bromo ketones and dimethyl acetylenedicarboxylate proceeded in the presence of catalytic amounts of H3PW12O40 and gave the corresponding symmetrical and unsymmetrical polysubstituted bis-pyrroles in moderate to good yields. To the best of our knowledge, no examples of the synthesis of polysubstituted bis-pyrroles via the multi-component reaction of diamines, α-bromo ketones and dimethyl acetylenedicarboxylate have been reported and consequently, such a synthesis can be considered as a remarkable characteristic of the present protocol in the preparation of these important heterocyclic compounds.
Synthesis of polysubstituted bis-pyrroles catalyzed by H3PW12O40.
A probable mechanistic pathway for the synthesis of polysubstituted pyrroles is illustrated in Scheme 2. First, H3PW12O40 activates dialkyl acetylenedicarboxylate to give A. Then, Michael-type addition of amine 1 with A provides the α,β-unsaturated N-arylamine intermediate B which upon reaction with α-bromo ketone 2 produces the intermediate C. Intramolecular cyclization of C, followed by dehydration in the presence of the catalyst generates the target heterocyclic compound 4 and liberates the catalyst for the next run.
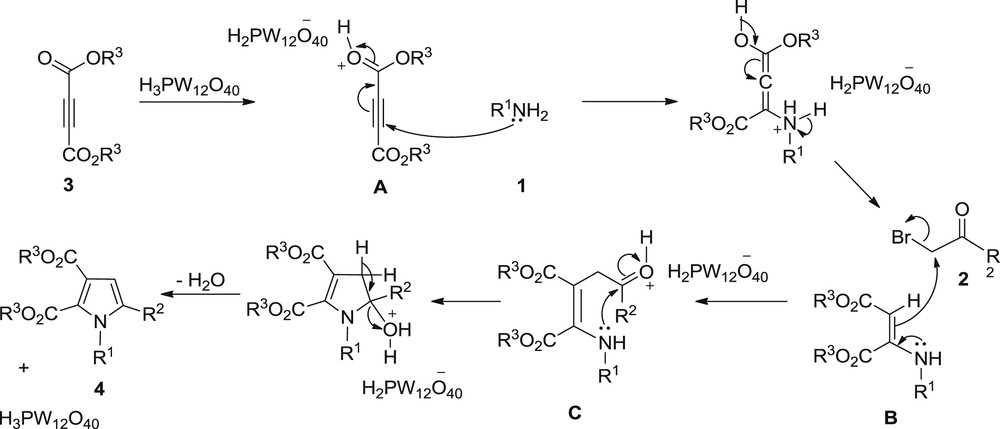
Probable mechanistic pathway for the synthesis of polysubstituted pyrroles.
The recyclability and reusability of the H3PW12O40 catalyst were scrutinized in the model reaction under the optimized conditions and the results are shown in Table 4. When the reaction was completed, ethyl acetate (10 mL) was added and the catalyst was easily separated by simple filtration. The recovered catalyst was washed with ethyl acetate, dried and reused in another set of reaction. It was observed that the catalyst can be recycled and reused up to four runs without any significant loss in its efficacy and activity.
3 Conclusion
In conclusion, an efficient and mild protocol has been developed for the synthesis of polysubstituted pyrroles using H3PW12O40 as a commercially available, inexpensive, environmentally friendly, non-corrosive, and reusable catalyst under solvent-free and ambient reaction conditions. We have also devised the first example of a straightforward synthesis of symmetrical and unsymmetrical polysubstituted bis-pyrroles via a one-pot multi-component reaction of diamines, α-bromo ketones and dimethyl acetylenedicarboxylate which makes this process attractive for the synthesis of these important heterocyclic compounds.
4 Experimental
4.1 General
Melting points were determined using a Stuart Scientific SMP2 apparatus. FT-IR spectra were recorded on a Nicolet-Impact 400D instrument in the range of 400–4000 cm−1. 1H and 13C NMR (400 and 100 MHz) spectra were recorded in a CDCl3 solution on a Bruker-Avance 400 spectrometer. Elemental analysis was done on a LECO, CHNS-932 analyzer.
4.2 General procedure for the synthesis of polysubstituted pyrroles
To a mixture of amine 1 (1 mmol), α-bromo ketone 2 (1 mmol) and dialkyl acetylenedicarboxylate 3 (1 mmol), was added H3PW12O40 (0.05 mmol, 144 mg). The reaction mixture was stirred at room temperature for 1 h. The progress of the reaction was monitored by TLC (eluent: ethyl acetate/petroleum ether, 1:7). After completion of the reaction, ethyl acetate (10 mL) was added; the catalyst was separated by simple filtration and washed with ethyl acetate (10 mL). Evaporation of the solvent followed by purification of the crude product by column chromatography on silica gel (eluent: ethyl acetate/petroleum ether, 1:7) afforded the pure product (Table 2).
4.3 General procedure for the synthesis of polysubstituted bis-pyrroles
A mixture of diamine 5 (1 mmol), α-bromo ketone 2 (2 mmol), dimethyl acetylenedicarboxylate 3 (2 mmol) and H3PW12O40 (0.1 mmol, 288 mg) was stirred at room temperature 1.5 h (for unsymmetrical bis-pyrroles, the reaction was performed with 1 mmol α-bromo ketone 2 and 1 mmol α-bromo ketone 6). The progress of the reaction was monitored by TLC (eluent: ethyl acetate/petroleum ether, 1:5). The work-up was performed as described in the general procedure for the synthesis of polysubstituted pyrroles and the crude product was purified by column chromatography on silica gel (eluent: ethyl acetate/petroleum ether, 1:5) to give the pure product (Table 3).
5 Spectroscopic data of the products
5.1 Dimethyl 1,5-diphenyl-1H-pyrrole-2,3-dicarboxylate (Table 2, entry 1)
Yellow oil [28c]. IR (KBr): νmax = 2931, 1725, 1501, 1452, 1345, 1257, 1085, 1015, 835, 805, 751 cm−1. 1H NMR (400 MHz, CDCl3): δ 3.62 (s, 3H), 3.66 (s, 3H), 6.82 (d, J = 8.0 Hz, 4H), 7.02 (t, J = 7.6 Hz, 2H), 7.20 (t, J = 8.4 Hz, 4H). 13C NMR (100 MHz, CDCl3): δ 51.2, 52.8, 118.6, 120.7, 122.3, 124.2, 125.4, 128.8, 129.2, 130.9, 131.9, 132.1, 140.3, 160.9, 166.3.
5.2 Dimethyl 5-(4-bromophenyl)-1-phenyl-1H-pyrrole-2,3-dicarboxylate (Table 2, entry 2)
Yellow oil [28d]. IR (KBr): νmax = 2927, 1723, 1498, 1450, 1351, 1257, 1080, 1017, 835, 755 cm−1. 1H NMR (400 MHz, CDCl3): δ 3.59 (s, 3H), 3.65 (s, 3H), 7.07 (s, 1H), 7.13 (d, J = 7.2 Hz, 2H), 7.21 (t, J = 7.6 Hz, 1H), 7.37 (t, J = 8.3 Hz, 2H), 7.59 (d, J = 8.4 Hz, 2H), 7.99 (d, J = 8.4 Hz, 2H). 13C NMR (100 MHz, CDCl3): δ 51.3, 52.8, 120.7, 122.3, 124.3, 125.5, 128.8, 129.2, 130.9, 132.2, 133.0, 140.3, 160.5, 166.6.
5.3 Dimethyl 5-(4-chlorophenyl)-1-phenyl-1H-pyrrole-2,3-dicarboxylate (Table 2, entry 3)
Yellow oil. IR (KBr): νmax = 2927, 1728, 1498, 1450, 1353, 1259, 1080, 1018, 835, 754 cm−1. 1H NMR (400 MHz, CDCl3): δ 3.59 (s, 3H), 3.66 (s, 3H), 7.15 (s, 1H), 7.57–7.67 (m, 7H), 8.00 (d, J = 8.4 Hz, 2H). 13C NMR (100 MHz, CDCl3): δ 51.4, 53.2, 118.6, 120.7, 123.0, 124.2, 125.4, 128.6, 129.2, 131.4, 131.7, 140.6, 160.1, 166.1.
5.4 Dimethyl 5-(4-fluorophenyl)-1-phenyl-1H-pyrrole-2,3-dicarboxylate (Table 2, entry 4)
Yellow oil. IR (KBr): νmax = 2933, 1721, 1505, 1452, 1355, 1261, 1085, 1021, 835, 749 cm−1. 1H NMR (400 MHz, CDCl3): δ 3.74 (s, 3H), 3.79 (s, 3H), 6.93 (s, 1H), 7.02 (t, J = 7.2 Hz, 2H), 7.35–7.47 (m, 7H). 13C NMR (100 MHz, CDCl3): δ 51.2, 52.8, 118.6, 120.7, 125.0, 126.6, 127.3, 127.8, 128.6, 129.2, 131.3, 132.4, 139.2, 140.8, 160.9, 167.3.
5.5 Dimethyl 5-(naphthalen-1-yl)-1-phenyl-1H-pyrrole-2,3-dicarboxylate (Table 2, entry 5)
Brown oil. IR (KBr): νmax = 2931, 1731, 1497, 1451, 1355, 1295, 1077, 1005, 829, 797, 753 cm−1. 1H NMR (400 MHz, CDCl3): δ 3.62 (s, 3H), 3.67 (s, 3H), 6.95–6.98 (m, 1H), 7.04–7.09 (m, 2H), 7.10–7.19 (m, 2H), 7.20–7.25 (m, 2H), 7.35–7.39 (m, 1H), 7.41–7.48 (m, 1H), 7.57–7.64 (m, 2H), 7.74 (dd, J = 8.3, J = 2.4 Hz, 1H), 7.80 (d, J = 8.2 Hz, 1H). 13C NMR (100 MHz, CDCl3): δ 52.4, 52.8, 112.7, 120.8, 120.9, 123.4, 124.4, 125.9, 126.0, 127.9, 128.4, 128.6, 128.9, 129.9, 130.8, 133.1, 141.7, 161.0, 165.0.
5.6 Dimethyl 5-phenyl-1-p-tolyl-1H-pyrrole-2,3-dicarboxylate (Table 2, entry 6)
Yellow oil [28e]. IR (KBr): νmax = 2924, 1727, 1498, 1447, 1347, 1297, 1255, 1073, 1005, 828, 795, 749 cm−1. 1H NMR (400 MHz, CDCl3): δ 2.42 (s, 3H), 3.62 (s, 3H), 3.66 (s, 3H), 7.10 (s, 1H), 7.18–7.23 (m, 7H), 7.65 (d, J = 8.1 Hz, 2H). 13C NMR (100 MHz, CDCl3): δ 22.7, 51.2, 52.8, 118.6, 120.1, 120.7, 124.3, 125.5, 128.1, 129.2, 130.9, 131.3, 133.0, 140.3, 160.2, 166.5.
5.7 Dimethyl 5-(4-methoxyphenyl)-1-phenyl-1H-pyrrole-2,3-dicarboxylate (Table 2, entry 7)
Yellow oil [28e]. IR (KBr): νmax = 2930, 1728, 1588, 1501, 1359, 1248, 1091, 829, 805, 755 cm−1. 1H NMR (400 MHz, CDCl3): δ 3.48 (s, 3H), 3.62 (s, 3H), 3.67 (s, 3H), 6.82 (d, J = 7.8 Hz, 2H), 7.03 (s, 1H) 7.41 (td, J = 7.8, 1.2 Hz, 2H), 7.49 (td, J = 7.8, 1.2 Hz, 2H), 7.59–7.52 (m, 3H). 13C NMR (100 MHz, CDCl3): δ 51.2, 52.7, 59.4, 116.7, 120.7, 122.3, 124.3, 125.4, 129.2, 130.9, 133.0, 140.3, 159.0, 162.1, 166.0.
5.8 Dimethyl 5-(4-bromophenyl)-1-p-tolyl-1H-pyrrole-2,3-dicarboxylate (Table 2, entry 8)
Yellow oil [28c]. IR (KBr): νmax = 2938, 1726, 1585, 1503, 1366, 1251, 1095, 832, 805, 761 cm−1. 1H NMR (400 MHz, CDCl3): δ 2.45 (s, 3H), 3.62 (s, 3H), 3.67 (s, 3H), 7.23 (d, J = 7.8 Hz, 2H), 7.27 (s, 1H) 7.30 (d, J = 7.3 Hz, 2H), 7.44 (d, J = 8.9 Hz, 2H), 7.53 (d, J = 8.5 Hz, 2H). 13C NMR (100 MHz, CDCl3): δ 22.7, 51.2, 52.8, 110.5, 118.6, 120.7, 124.3, 125.5, 128.1, 129.2, 130.2, 130.9, 131.3, 133.0, 140.3, 160.4, 166.4.
5.9 Diethyl 1,5-diphenyl-1H-pyrrole-2,3-dicarboxylate (Table 2, entry 9)
Yellow oil [28g]. IR (KBr): νmax = 2935, 1728, 1595, 1498, 1353, 1251, 1085, 835, 803, 755 cm−1. 1H NMR (400 MHz, CDCl3): δ 1.18 (t, J = 6.9 Hz, 3H), 1.37 (t, J = 7.1 Hz, 3H), 3.92 (q, J = 6.8 Hz, 2H), 4.16 (q, J = 6.7 Hz, 2H), 6.82 (d, J = 8.0 Hz, 4H), 7.02 (t, J = 7.6 Hz, 2H), 7.21 (t, J = 8.4 Hz, 4H). 13C NMR (100 MHz, CDCl3): δ 13.6, 14.2, 60.0, 62.4, 118.6, 120.7, 125.8, 122.6, 124.3, 125.8, 126.2, 127.1, 128.3, 129.2, 133.0, 140.3, 160.6, 166.4.
5.10 Diethyl 5-(4-bromophenyl)-1-phenyl-1H-pyrrole-2,3-dicarboxylate (Table 2, entry 10)
Brown oil [28f]. IR (KBr): νmax = 2943, 1738, 1578, 1521, 1364, 1266, 1085, 835, 803, 755 cm−1. 1H NMR (400 MHz, CDCl3): δ 1.11 (t, J = 6.9 Hz, 3H), 1.26 (t, J = 7.1 Hz, 3H), 3.92 (q, J = 7.1 Hz, 2H), 4.02 (q, J = 6.7 Hz, 2H), 7.44–7.49 (m, 3H), 7.55–7.58 (m, 2H) 7.63 (d, J = 8.4 Hz, 2H), 7.74 (d, J = 8.2 Hz, 2H). 13C NMR (100 MHz, CDCl3): δ 12.6, 14.7, 61.0, 63.2, 118.8, 121.3, 123.0, 124.3, 125.7, 126.5, 127.3, 128.8, 130.5, 133.0, 138.0, 141.7, 160.0, 166.2.
5.11 Dimethyl 1-benzyl-5-phenyl-1H-pyrrole-2,3-dicarboxylate (Table 2, entry 11)
Yellow oil [28d]. IR (KBr): νmax = 2931, 1725, 1497, 1451, 1355, 1295, 1077, 1005, 829, 797, 753 cm−1. 1H NMR (400 MHz, CDCl3): δ 3.68 (s, 3H), 3.72 (s, 3H), 5.53 (s, 2H), 7.22 (m, 2H), 7.34 (td, J = 7.5, 1.2 Hz, 2H), 7.46–7.49 (m, 3H), 7.63–7.66 (m, 2H), 7.71 (d, J = 9.0 Hz, 2H). 13C NMR (100 MHz, CDCl3): δ 51.2, 52.4, 52.8, 120.7, 124.2, 125.6, 126.9, 127.1, 127.2, 128.8, 129.2, 130.9, 132.5, 139.0, 161.5, 167.8.
5.12 Dimethyl 1-methyl-5-phenyl-1H-pyrrole-2,3-dicarboxylate (Table 2, entry 12)
Yellow oil [28f]. IR (KBr): νmax = 2928, 1731, 1501, 1435, 1339, 1288, 1081, 1012, 841, 805, 751 cm−1. 1H NMR (400 MHz, CDCl3): δ 3.62 (s, 3H), 3.66 (s, 3H), 3.86 (s, 3H), 7.02 (s, 1H), 7.25 (t, J = 8.4 Hz, 2H). 7.32–7.38 (m, 1H), 7.46 (d, J = 8.4 Hz, 2H). 13C NMR (100 MHz, CDCl3): δ 30.1, 52.1, 54.1, 118.5, 120.9, 122.3, 124.6, 125.6, 129.3, 134.6, 142.0, 160.1, 166.0.
5.13 Tetramethyl 1,1′-(1,4-phenylene)bis(5-phenyl-1H-pyrrole-2,3-dicarboxylate) (Table 3, entry 1)
Mp 105–108 °C. IR (KBr): νmax = 2933, 1731, 1512, 1352,1261, 1105, 841, 805, 751 cm−1. 1H NMR (400 MHz, CDCl3): δ 3.69 (s, 6H), 3.78 (s, 6H), 7.12 (s, 2H), 7.33 (t, J = 7.9 Hz, 2H), 7.38 (t, J = 7.7 Hz, 4H), 7.57 (s, 4H), 7.67 (d, J = 7.5 Hz, 4H). 13C NMR (100 MHz, CDCl3): δ 51.8, 54.2, 119.2, 121.0, 122.3, 127.7, 129.4, 130.7, 131.9, 138.0, 139.9, 140.2, 161.0, 167.0. Anal. Calcd. for C34H28N2O8: C, 68.91; H, 4.76; N, 4.73. Found: C, 68.80; H, 4.80; N, 4.81.
5.14 Tetramethyl 1,1′-(1,4-phenylene)bis(5-(4-bromophenyl)-1H-pyrrole-2,3-dicarboxylate) (Table 3, entry 2)
Mp 103–105 °C. IR (KBr): νmax = 2927, 1729, 1510, 1448, 1351, 1261, 1085, 1012, 841, 805, 755 cm−1. 1H NMR (400 MHz, CDCl3): δ 3.76 (s, 6H), 3.83 (s, 6H), 7.44 (s, 4H), 7.54–7.60 (m, 4H), 7.76 (d, J = 8.6 Hz, 3H), 7.92 (d, J = 8.6 Hz, 2H). 13C NMR (100 MHz, CDCl3): δ 51.2, 52.8, 118.4, 120.8, 122.34, 124.2, 125.5, 129.3, 130.9, 132.2, 140.3, 143.5, 160.9, 166.1. Anal. Calcd. for C34H26Br2N2O8: C, 54.42; H, 3.49; N, 3.73. Found: C, 54.53; H, 3.52; N, 3.65.
5.15 Tetramethyl-1,1′-(1,4-phenylene)bis(5-(4-chlorophenyl)-1H-pyrrole-2,3-dicarboxylate) (Table 3, entry 3)
Mp 98–101 °C. IR (KBr): νmax = 2927, 1729, 1510, 1448, 1351, 1261, 1085, 1012, 841, 805, 755 cm−1. 1H NMR (400 MHz, CDCl3): δ 3.59 (s, 6H), 3.74 (s, 6H), 6.92 (s, 2H), 7.54–7.60 (m, 4H), 7.75 (d, J = 8.3 Hz, 4H), 7.92 (d, J = 8.7 Hz, 4H). 13C NMR (100 MHz, CDCl3): δ 51.2, 52.8, 118.6, 120.7, 124.3, 125.65, 128.0, 129.2, 130.9, 133.0, 140.3, 143.78, 160.9, 165.8. Anal. Calcd. for C34H26Cl2N2O8: C, 61.73; H, 3.96; N, 4.23. Found: C, 61.85; H, 3.92; N, 4.29.
5.16 Dimethyl-1-(4-(2,3-bis(methoxycarbonyl)-5-phenyl-1H-pyrrol-1-yl)phenyl)-5-(4-bromophenyl)-1H-pyrrole-2,3-dicarboxylate (Table 3, entry 4)
Mp 89–91 °C. IR (KBr): νmax = 2945, 1763, 1438, 1351, 1287, 1115, 855, 805, 755 cm−1. 1H NMR (400 MHz, CDCl3): δ 3.60 (s, 6H), 3.64 (s, 6H), 7.3 (s, 2H), 7.46 (td, J = 7.1, 1.6 Hz, 1H), 7.54 (td, J = 6.8, 1.4 Hz, 2H), 7.60–7.62 (m, 4H), 7.67 (d, J = 8.8 Hz, 2H), 7.85–7.89 (m, 4H). 13C NMR (100 MHz, CDCl3): δ 51.2, 52.8, 116.7, 118.6, 120.7, 122.3, 124.3, 126.8, 128.1, 128.8, 129.2, 132.1, 138.0, 138.6, 140.3, 160.8, 166.6. Anal. Calcd. for C34H27BrN2O8: C, 60.81; H, 4.05; N, 4.17. Found: C, 60.71; H, 4.09; N, 4.23.
5.17 Dimethyl-1-(4-(2,3-bis(methoxycarbonyl)-5-(naphthalen-2-yl)-1H-pyrrol-1-yl)phenyl)-5-phenyl-1H-pyrrole-2,3-dicarboxylate (Table 3, entry 5)
Mp 97–99 °C. IR (KBr): νmax = 2935, 1741, 1501, 1459, 1359, 1305, 1081, 1005, 831, 797, 753 cm−1. 1H NMR (400 MHz, CDCl3): δ 3.64 (s, 6H), 3.69 (s, 6H), 7.29 (s, 2H), 7.43–7.55 (m, 4H), 7.62–7.64 (m, 2H), 7.82 (t, J = 6.7 Hz, 4H), 7.90 (d, J = 7.8 Hz, 2H), 7.97 (dd, J = 8.6, 1.56 Hz, 2H), 8.40 (s, 2H). 13C NMR (100 MHz, CDCl3): δ 53.0, 54.4, 118.6, 120.2, 122.2, 123.9, 126.8, 127.2, 127.8, 128.4, 128.5, 128.8, 129.6, 130.2, 130.9, 132.5, 134.5, 135.6, 139.7, 161.2, 166.1. Anal. Calcd. for C38H30N2O8: C, 71.02; H, 4.71; N, 4.36. Found: C, 71.14; H, 4.75; N, 4.28.
5.18 Tetramethyl-1,1′-(1,3-phenylene)bis(5-phenyl-1H-pyrrole-2,3-dicarboxylate) (Table 3, entry 6)
Yellow oil. IR (KBr): νmax = 2945, 1751, 1461, 1367, 1275, 1105, 1005, 843, 805, 755 cm−1. 1H NMR (400 MHz, CDCl3): δ 3.63 (s, 6H), 3.66 (s, 6H), 7.30–7.34 (m, 4H), 7.35–7.39 (m, 4H), 7.40–7.44 (m, 4H); 7.61 (dd, J = 6.7, 2.1 Hz, 4H). 13C NMR (100 MHz, CDCl3): δ 52.3, 54.3, 112.0, 116.4, 119.4, 121.0, 122.5, 127.4, 128.8, 129.6, 130.3, 138.2, 140.2, 142.2, 160.3, 165.7.
5.19 Tetramethyl-1,1′-(1,3-phenylene)bis(5-(4-bromophenyl)-1H-pyrrole-2,3-dicarboxylate) (Table 3, entry 7)
Brown oil. IR (KBr): νmax = 2939, 1756, 1455, 1369, 1277, 1105, 851, 805, 755 cm−1. 1H NMR (400 MHz, CDCl3): δ 3.68 (s, 6H), 3.78 (s, 6H), 7.2 (s, 2H), 7.30–7.40 (m, 4H), 7.67 (d, J = 7.4 Hz, 4H); 7.79 (d, J = 8.1 Hz, 4H). 13C NMR (100 MHz, CDCl3): δ 52.1, 54.5, 117.5, 118.6, 121.5, 123.3, 124.1, 127.4, 128.4, 129.8, 130.7, 132.6, 139.6, 141.3, 160.8, 166.1.
Acknowledgments
The authors are grateful to the Research Council of the University of Isfahan for financial support of this work.