1 Introduction
During the past few decades, biomaterials manufactured from natural or synthetic polymers [1], ceramics and metals have been studied and widely used in the medical field [2,3]. The high biocompatibility, similarity to native extracellular matrices and well-established safety profiles have made collagen the primary resource for tissue regeneration and drug delivery applications. The main applications of collagen as a drug delivery system (DDS) are collagen sponges in dentistry [4], collagen membranes for transdermal delivery [5], shields in ophthalmology [6], tablets, nanoparticles for gene delivery [7] and bone substitutes.
Matrices in the form of sponges are able to encapsulate different active substances for controlled delivery systems due to their flexibility to obtain a desirable release profile and cost effectiveness. In dentistry, pain management is an important part that encompasses a number of procedural issues, including drug delivery, management of postoperative [8] or preoperative pain for chronic pain and diagnosis. As an analgesic and anti-inflammatory drug, ibuprofen (2-(4-isobutylphenyl) propionic acid) (IB) (Fig. 1) is one of the NSAID (non-steroidal anti-inflammatory drugs) prototypes widely used for the treatment of dental pain and inflammation following surgical procedures [9].

Chemical structure of ibuprofen.
The purpose of the controlled release systems is to maintain the drug concentration as long as possible in the target tissues at a desired value. The research on the efficacy and effectiveness of treatments in preventing and controlling dental caries extends beyond the ways to administer an active substance to exert a control on the drug release rate and time. In most cases, drug release from hydrophilic supports takes place by diffusion from swollen matrices or by enzymatic matrix degradation. To reduce water uptake and to prolong the release for an extended period of time, collagen matrices have been chemically or dehydrothermally cross-linked. Collagen cross-linking can be achieved in several ways such as chemical [10,11], enzymatic [12] or physical [13]. The most used cross-linking agent is glutaraldehyde (GA), the treatment with GA being fast, efficient and inexpensive.
In this study, preparation and characterization of collagen-based biomaterials containing analgesic and anti-inflammatory drugs such as IB, and GA as a cross-linking agent are presented. The structural and morphological characteristics of the obtained collagen sponges were examined by infrared spectroscopy (FT-IR) and water uptake. Another objective of this work was to evaluate in vitro drug release data using various kinetic models and to determine the mechanism of drug release. The biomaterial with the best properties will be chosen for treatment of some inflamed and painful lesions at the dental level.
2 Materials and methods
2.1 Materials
Pure collagen in the form of gel with an initial concentration of 2.11% was extracted from bovine by the Collagen Department of Division Leather and Footwear Research Institute, Romania. The analgesic and anti-inflammatory drug – ibuprofen (IB) – was purchased from ICN Biomedicals Inc. (USA), and glutaraldehyde (GA) was purchased from Merck (Germany). For pH adjustment, sodium hydroxide 1M (NaOH) of analytical grade was used, and a phosphate buffer solution (PBS) with a pH of 7.4 was prepared and used in the drug release study. For the enzymatic degradation test, the collagenase of Clostridium histolyticum from Sigma–Aldrich (USA) was used.
2.2 Preparation of collagen-based biomaterials
Traditional methods for producing biomaterials include gas foaming [14], freeze-drying [4], casting solution [15], phase separation and sintering depending on the material used to produce the scaffold. Freeze-drying is the easiest method to obtain porous sponges. Collagen-based biomaterials with or without GA and IB, and with defined shapes and sizes were prepared by the freeze-drying process in a few steps. Firstly, the pH of the collagen gel with a fibrillar structure was adjusted from 2.5 to 7.4 under mechanical stirring with 1M NaOH solution. Then, the collagen gel (CG) was mixed with a water solution of IB 1 g/L and cross-linked with 1% GA solution. The concentration of GA with respect to the dry substance was 0, 0.25, 0.5, and 0.75–1%. All the samples have a concentration of 4% IB with respect to the dry substance. As the final step, the homogeneous gel was freeze-dried using a freeze-dryer Delta 2-24 LSC (Martin Christ, Germany), in order to obtain disc-shaped porous collagen sponges with a thickness of 10 mm. The obtained collagen sponges were coded as follows: CG IB GA 0%, CG IB GA 0.25%, CG IB GA 0.50%, CG IB GA 0.75% and CG IB GA 1%, respectively.
2.3 Methods
Infrared analysis was performed using Attenuated Total Reflection FT-IR (ATR-FTIR) with a PerkinElmer spectrum 100 FT-IR spectrophotometer where biomaterials were placed directly onto the diamond crystal for data acquisition. Spectral data were recorded by collecting four scans from 4000 cm−1–600 cm−1 with a resolution of 4 cm−1.
In order to establish the swelling behaviour, collagen based-biomaterial discs (diameter: 5 mm, thickness: 10 mm) were weighed (Wd) and then immersed in distilled water at 25 °C for different time intervals until a stable mass was obtained (Ww). The water uptake W (%) of the obtained sponges was determined as defined by equation (1). The test was performed in triplicate.
(1) |
The in vitro degradation test was performed using bacterial collagenase of C. histolyticum from Sigma–Aldrich (USA) in PBS with pH 7.4. Each sponge was accurately weighed, placed in PBS solution, incubated at 37 °C and then, collagenase (10 μg/mL) was added and the test tube was incubated again at 37 °C. At regular moments in time (1 h, 2 h, 4 h, 8 h, 24 h, 48 h, and 72 h), the samples were removed from the medium, squeezed and their weight loss was calculated according to equation (2). Each test was performed 3 times.
(2) |
The drug release kinetics from sponges were studied using a sandwich device adapted to a paddle dissolution apparatus (Essa Dissolver, Italy) [16]. The release medium was phosphate buffer (pH 7.4) maintained at a constant temperature of 37 ± 0.1 °C. 5 mL aliquots were extracted at predetermined time intervals for 10 h, and the withdrawn volume was replaced with 5 mL of fresh phosphate buffer. The concentration of the drug in the release medium was spectrophotometrically evaluated at a wavelength of 264.5 nm (, R2 = 0.9999). Each experiment was performed in triplicate. To confirm the drug release kinetics mechanism, the in vitro release data were fitted according to the power-law model (equation (3)) and the Higuchi model (equation (4)) [17].
(3) |
(4) |
3 Results and discussion
3.1 Infrared spectroscopy
All collagen sponges with and without anti-inflammatory drugs and cross-linking agents were characterized by FT-IR spectrometry in order to find out the peak position of functional groups for collagen, IB and GA.
The IR spectrum of pure IB (Fig. 2) shows the next major bands [18–20]:
- • the band at 2955 cm−1 and 2924 cm−1 corresponding to the alkyl stretching vibration;
- • a well defined infrared band at 1701 cm−1 attributed to the CO stretching vibration;
- • the absorption band from 1365 cm−1 attributed to the stretching vibration of C–C–O (νc–c–o), and to the deformation vibration of C–H (δC–H) and CO–H (δCO–H);
- • CO–H in plane bending (H-bonded) appeared at 1230 cm−1;
- • a maximum absorption peak corresponding to ΦCH in plane bending and to the C3–C24 stretching (νc–c) at 1168 cm−1;
- • an important band at 780 cm−1 specific to the aromatic stretching bending vibration.

FT-IR spectra of ibuprofen and collagen based-biomaterials.
In the case of the collagen sponge without GA or IB the main functional groups [16] are registered at 3294 cm−1 corresponding to νNHas and νOHas, at 1630 cm−1, 1549 cm−1 and 1339 cm−1 assigned to the stretching vibration of CO (νco) from amide I, to the deformation vibration of N–H (δN–H) and to the stretching vibration of the C–N bond (νC–N) from amide II, and also to the stretching vibration of C–C (νc–c) from amide III, respectively.
Incorporation of drug inside the collagen matrix was discussed based on the presence of vibration bands typical of IB besides the bands attributed to the other components used. Taking into account the above information, the FT-IR spectra of collagen sponges with IB and different concentrations of GA (Fig. 2) showed characteristic absorption bands of collagen [16], IB and the cross-linking agent as follows: the band corresponding to νNHas + νOHas appeared in the 3329–3309 cm−1 domain, while for the simple collagen sponge without IB and GA the same band was registered at 3294 cm−1. This behaviour is characteristic of hydrogen bond interactions between the collagen molecules and anti-inflammatory active substance. The absorption band specific to the CH2 group in IB is registered at 2925 cm−1 in collagen sponges with IB and GA. In the 1638–1633 cm−1 domain, the sponges with IB and GA clearly show that the amide I band from collagen is present. The presence of IB favours the shifting of the wavenumber from 1630 cm−1 (in CG sponge) [16] to higher values, and this fact indicates the association (cross-linking) between collagen and drug by hydrogen bonds. The amide II and amide III bands are also registered in all prepared sponges at around 1549 cm−1 and 1340 cm−1 . Other three significant bands for IB are registered in collagen sponges with IB and GA at 1365 cm−1, 1165 cm−1 and 787 cm−1.
3.2 Swelling behaviour
The swelling test was performed in order to evaluate the sponge water uptake ability. We have found previously [16] that plotting the water uptake against the concentration of the drug and cross-linking agent in the biomaterials facilitates the interpretation of swelling behaviour of sponges. Fig. 3 shows the swelling percentage dependence of the collagen based-biomaterial on its composition.
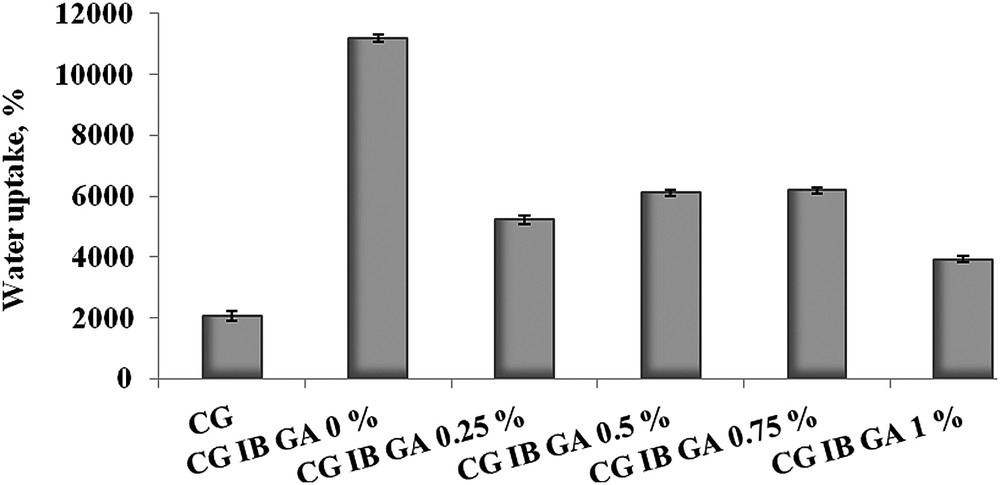
Swelling percentage corresponding to collagen-based biomaterials.
Comparing the collagen sponge without IB or GA and the collagen sponge with IB showed that the presence of the anti-inflammatory drug significantly increased the water uptake. When GA is introduced into the sponge composition an important decrease in the percentage of water absorbed was observed. Considering the final application of these new sponges the swelling behaviour is very important. A high value recorded for water uptake is not a desired phenomenon, and further in vitro degradation tests and IB release measurements will indicate the optimum amount of cross-linking agent required for drug release systems used for the treatment of dental problems.
3.3 In vitro degradation
The in vitro degradation test of collagen-based biomaterials was investigated by monitoring the weight loss depending on the exposure time to the collagenase solution. The biodegradation results of the collagen sponges with or without IB and different percentages of the cross-linking agent are presented in Fig. 4.

In vitro degradation of collagen-based biomaterials with IB and GA.
It can be seen that the CG IB sponge uncrosslinked with GA was totally degraded after only 1 h. As we expected from FT-IR data, the collagen sponges with IB and GA were digested at different times depending on the cross-linking agent. Therefore, a complete degradation was noticed after 24 h for sponges cross-linked with 0.25% GA and after 72 h for sponges cross-linked with more than 0.25% GA (0.5%, 0.75% and 1% GA, respectively). Comparing the results for collagen sponges with 0.5% GA and 0.75% GA after 24 h a small degradation is obtained for 0.5% GA, but after 48 h the weight loss values are similar for both cases. In order to obtain a good material with the best resistance to the action of collagenase, the efficient cross-linking degree is considered to be 0.5%.
3.4 Ibuprofen release from collagen-based biomaterials
The kinetic profiles [21,22] corresponding to the controlled release of IB in time from collagen-based biomaterials with and without cross-linking agent are presented in Fig. 5.

In vitro ibuprofen release in phosphate buffer at pH 7.4.
The percent of IB released at different time intervals depends on the amount of cross-linking agent, the increase of GA leading to a slower release and to the achievement of the equilibrium a long time. This behaviour is due to the strong chemical cross-linking determined by the increase in the GA amount. It was noticed that collagen-based biomaterials without GA showed the highest drug released percent (90.95%), while for the biomaterials with the highest content of GA a percent of 74.09% was obtained (Table 1).
Correlation coefficients R for ibuprofen release from collagen sponges obtained using the power-law and Higuchi models; the kinetic parameters specific to the power-law model.
Sponge | Kinetic constant (1/minn) | Release exponent | IB released percent (%) | R (power-law model) | R (Higuchi model) |
CG IB GA 0% | 0.165 | 0.279 | 90.95 | 0.9742 | 0.9307 |
CG IB GA 0.25% | 0.111 | 0.335 | 86.51 | 0.9647 | 0.9441 |
CG IB GA 0.50% | 0.083 | 0.373 | 82.07 | 0.9643 | 0.9504 |
CG IB GA 0.75% | 0.060 | 0.413 | 77.25 | 0.9743 | 0.9679 |
CG IB GA 1% | 0.049 | 0.438 | 74.09 | 0.9821 | 0.9788 |
To quantify the drug release experimental data two kinetic models were used (equation (3) and equation (4)). Comparing the correlation coefficients obtained for the power-law and Higuchi model, the highest values were obtained for the power-law equation, suggesting that this model describes better the release process.
Table 1 presents the kinetic constant and the release exponent values characteristic of the power-law model, the percentage of IB released and the correlation coefficients for both tested kinetic models.
The kinetic constant values confirm that the increase in the cross-linking agent concentration promotes a slow IB release.
Taking into account that an adequate drug release system should provide a moderate release rate with a large amount of drug, the release data corroborated with those from swelling behaviour and enzymatic degradation lead to the conclusion that the best formulation of collagen-based biomaterials with IB is with 0.5% of GA.
4 Conclusions
Systems based on collagen and ibuprofen as an analgesic and anti-inflammatory active substance for drug delivery were obtained. The presence of IB inside the collagen sponges is confirmed by the IR spectral method. Measurements related to enzymatic and hydrolytic degradation show that the increase in the GA concentration inhibits collagen degradation and decreases the water uptake. The kinetic profiles for controlled release of anti-inflammatory drug from cross-linked collagen sponges with different amounts of GA emphasize the influence of the cross-linking agent on the release constant and on the percentage of drug release. Drug formulations for dental applications must release the active substance in a long time and this can be controlled by the amount of GA added. Concerning the results obtained for all prepared and analysed sponges, the most biologically stable collagen-based biomaterial is the collagen sponge with IB and 0.5% GA. As collagen is nontoxic, biodegradable and biocompatible, it can be successfully used for delivery systems of IB using an optimum amount of GA which enhances the resistance of enzymatic degradation and ensures a moderate release rate for a large amount of IB. The properties of the designed formulations demonstrate that these new biomaterials containing IB could be adequate for treatment of some inflamed and painful lesions at the dental level.