1 Introduction
Benzimidazole and benzothiazole derivatives possess a variety of biotic activities. These heterocyclic compounds have shown different pharmacological activities such as antibacterial, antiulcer, antihypertensive, antiviral, antifungal, anticancer, and antihistamine activities [2–7].
Benzimidazole derivatives exhibit significant activity against several viruses such as HIV [3,8], influenza and human cytomegalovirus (HCMV) [8]; they also act as topoisomerase inhibitors [3], selective neuropeptide YY1 receptor antagonists [11], angiotensin II inhibitors [13], and smooth muscle cell proliferation inhibitors [14] and have much more importance in organic synthesis.
Collagen is the most common protein family in the body of living creatures [17]. Collagen has an important role in the formation of tissues and organs and is involved in various functional expressions of cells. Skin, bone, tendon, teeth and blood vessels are some of the living organs where collagen can be found. The main sources of commercial collagen are limited to those of land-based creatures, such as bovine or porcine skins and bones [19].
Collagen has usefulness in a wide variety of biomedical biological applications. As the primary reason, collagen has superior biocompatibility compared with other natural polymers, such as albumin and gelatine. In addition, it can form fibres with extra strength and stability. These features are derived from its enriched functional groups, cross-linkage of its fibres and its supramolecular structure [21].
Until now, a number of methods have been developed for the synthesis of benzimidazoles [22–25]. Generally, 2-substituted benzimidazoles are synthesized using 1,2-phenylendiamine with aldehydes and/or acyl chlorides, carboxylic acids, and orthoesters. However, some synthesis conditions comprise the use microwave irradiation in an acidic medium [26] and/or refluxing in the presence of homo/heterogeneous catalysts such as acetic acid [27], silica supported sulfuric acid [28], Zinc-proline [29], Yb(OTf)3 [30], etc.
In addition, a lot of methods have been reported for the synthesis of benzothiazoles by condensation of benzene, 2-aminothiophenol with acyl chlorides or aldehydes. In this case, synthesis procedures include the use of ionic liquid [31] and/or microwave irradiation with a SiO2 catalyst [32] and/or refluxing in the presence of homo/heterogeneous catalysts such as acetic acid [33], active carbon in toxic solvents [34] and Chitosan-supported Fe3O4 [35].
To the best of our knowledge, most of the methods for the synthesis of benzimidazoles and benzothiazoles suffer from one or some disadvantages such as low yields, harsh reaction conditions, time consuming process, use of expensive catalysts and tedious workups. Thus presently, the development of environmentally benign, high-yielding and fast synthesis of benzimidazole and benzothiazole derivatives remains a desired goal in organic synthesis. In this work, we report a highly efficient procedure for the preparation of benzimidazole and benzothiazole derivatives in ethanol (EtOH) media using Fe3O4@SiO2/collagen as an efficient magnetic reusable nanocatalyst.
2 Experimental
2.1 Materials and methods
Hydrolysed collagen was of industrial grade (Parvar Novin-e Tehran Co., Mw ¼ 2000–20,000 Da), which is available in the market, and it has approximately 20% insoluble inorganic salts [36,37]. All chemicals were purchased from Merck, Fluka and Sigma–Aldrich companies and were used without further purification.
All reactions and the purity of benzimidazole and benzothiazole derivatives were monitored by thin-layer chromatography (TLC) using aluminium plates coated with silica gel F254 plates (Merck) using ethyl acetate, n-hexane and methanol as eluents. Melting points were determined in open capillaries using an Electrothermal 9100 instrument.
Fourier transform infrared (FT-IR) spectra were recorded on a Shimadzu FT-IR 8400s using KBr plates of samples. Proton nuclear magnetic resonance (1H NMR) spectra were recorded on a Bruker 400 ultrashield and DMSO-d6 was used as the solvent. A transmission electron microscope (TEM) from day-petronic company of Iran was used. Scanning electron microscopy (SEM) and energy dispersive X-ray (EDX) analysis were performed on a VEGA II TESCAN using 30 KV in high vacuum and Au spin coating for SEM sample preparation. Wide-angle powder X-ray diffraction (XRD) patterns of the solids were obtained in a JEOL with a Cu Kα (λ = 0.15420 nm) X-ray irradiation source in a 2θ range between 5° and 80°. Magnetic properties were recorded by the vibrational sampling magnetometry (VSM) technique in 1.5 T external magnetic fields at room temperature, by using a MDK-6 instrument.
2.2 Preparation of Fe3O4 magnetic nanoparticles
Fe3O4 nanoparticles were synthesised via a coprecipitation method by using ferric chloride (FeCl3·6H2O) and ferrous chloride (FeCl2·4H2O) that was introduced in our previous paper [38]. Briefly, Ferric chloride and ferrous chloride dissolved in degassed water and ammonia solution were added to this mixture under vigorous stirring. When pH increases, black colloids of Fe3O4 nanoparticles were formed. Fe3O4 nanoparticles were separated by using an external magnet in isoelectric point (pH ∼ 8). Then, the collected precipitate was poured in EtOH 50% and separated by using a magnet (rinsed using magnet separation) three times. Finally, Fe3O4 nanoparticles were dried in a vacuum oven.
2.3 Preparation of the Fe3O4@SiO2/collagen nanocatalyst
First, 2 g of Fe3O4 nanoparticles and 5 mL of tetraethyl orthosilicate (TEOS) were added in a 500-mL round bottom flask that contains 150 mL EtOH 50%. In the second vessel, 10 g of collagen was dissolved in 100 mL of a dilute acetic acid solution (5%) under vigorous stirring. A homogeneous supernatant of the second mixture was added to the Fe3O4 mixture. The flask was poured in an ultrasonic bath for 30 min. Then, NH4OH (10%) was added dropwise to the mixture until the pH reached about eight. In the next step, the residue was collected by using an external magnet; it was poured in a Teflon autoclave that contains EtOH (50%) and heated at 90 °C for 6 h. The resulting product was separated by external magnetic fields, dispersed in EtOH 50% and rinsed three times. Finally, it was dried in a vacuum oven at 60 °C for 12 h.
2.4 Synthesis of the non-magnetic nanocatalyst (SiO2/collagen)
The nonmagnetic nanocatalyst (SiO2/collagen) was synthesised by the same procedure (mentioned in 2.3.) without using Fe3O4 and tripling the amount of TEOS.
2.5 Synthesis of the non-silicate magnetic nanocatalyst (Fe3O4@collagen)
The non-silicate magnetic nanocatalyst (Fe3O4@collagen) was synthesised by the same procedure (mentioned in 2.3) without using TEOS in the synthesis procedure.
2.6 Synthesis of the collagen-less magnetic nanocatalyst (Fe3O4@SiO2)
The collagen-less magnetic nanocatalyst (Fe3O4@collagen) was synthesised by the same procedure (mentioned in 2.3) without the addition of the second mixture (collagen) in the synthesis procedure.
2.7 General procedure for the synthesis of benzimidazole derivatives
Aromatic aldehyde (1.0 mmol) and 1,2-phenylendiamine (1.0 mmol) were mixed with a catalytic amount of Fe3O4@SiO2/collagen (50 mg) in 4 mL of EtOH and stirred for indicated times (Table 2) at room temperature. Thin-layer chromatography (TLC) was used to monitor the progress of the reaction. After completion of the reaction, the catalyst was separated by external magnetic fields and reused four times in other fresh reactions without a considerable loss of activity. Then the products were crystallized using EtOH 96%. The products were characterized by melting point, FT-IR and 1H NMR. After each run, the catalyst was separated by using a magnet, rinsed with EtOH and dried. Then, it was used in the next reaction without impressive loss in catalytic reactivity.
Synthesis of benzimidazole derivatives in the presence of Fe3O4@SiO2/collagen.a
MP (°C) | Yield (%) | Time (min) | Product | Di-amine | Aldehyde | Entry | |
Found | Reported | ||||||
315–317 [39] | 314–318 | 78 | 12 | OPD | 4-NO2C6H4CHO | 1 | |
185–187 [40] | 186–188 | 97 | 15 | OPD | 3-NO2C6H4CHO | 2 | |
261–263 [41] | 260 | 78 | 15 | OPD | 2-NO2C6H4CHO | 3 | |
297–298 [42] | 296.5–298 | 85 | 15 | OPD | 4-BrC6H4CHO | 4 | |
262 [43] | 261 | 90 | 20 | OPD | 4-CNC6H4CHO | 5 | |
281–283 [40] | 281–282 | 60 | 20 | OPD | 4-ClC6H4CHO | 6 | |
227 [44] | 225 | 50 | 20 | OPD | 2-ClC6H4CHO | 7 | |
220 [45] | 218–220 | 87 | 18 | OPD | 2,4-Cl2C6H3CHO | 8 | |
220 [46] | 218–222 | 73 | 25 | OPD | 2,6-Cl2C6H3CHO | 9 | |
180–182 [18] | 181 | 55 | 30 | OPD | OCH3C6H4CHO | 10 | |
456–458 [47] | >400 | 83 | 20 | OPD | CHOC6H4CHO | 11 | |
342–343 [48] | 345 | 97 | 25 | OPD | 2-Thenaldehyde | 12 | |
234–235 [49] | 234–238 | 65 | 60 | OPD | Furfural | 13 | |
183–185 [42] | 185 | 70 | 30 | m-COOH(OPD) | 4-CNC6H4CHO | 14 | |
191–192 [42] | 189 | 73 | 25 | m-NO2(OPD) | 4-CNC6H4CHO | 15 |
a Reaction conditions: 1 mmol 1,2 phenylendiamine(OPD), 1 mmol aldehyde, catalytic Fe3O4@SiO2/collagen (50 mg), 4 mL solvent at rt.
2.8 General procedure for the synthesis of benzothiazole derivatives
Aromatic aldehyde (1.0 mmol) and 2-amino benzothiazole (1.0 mmol) were mixed with a catalytic amount of Fe3O4@SiO2/collagen (50 mg) in 4 mL of EtOH and stirred for indicated times (Table 3) under refluxing conditions. The progress of the reaction was monitored by TLC. After completion of the reaction, the catalyst was separated by external magnetic fields, and reused four times in other fresh reactions without a considerable loss of activity. Then the products were crystallized using EtOH 96%. The products were characterized by melting point, FT-IR and 1H NMR. After each run, the catalyst was separated by using a magnet, rinsed with EtOH and dried. Then, it was used in the next reaction without impressive loss in catalytic reactivity.
Synthesis of benzothiazole derivatives the presence of Fe3O4@SiO2/collagen.a
Entry | Aldehyde | 2-ABT | Product | Time (min) | Yield (%) | MP (°C) | |
Found | Reported | ||||||
1 | 4-NO2C6H4CHO | 2-ABT | 90 | 70 | 223–226 | 224–225 [1] | |
2 | 3-NO2C6H4CHO | 2-ABT | 60 | 83 | 180–182 | 180–181 [9] | |
3 | 4-CNC6H4CHO | 2-ABT | 35 | 78 | 161–162 | 161–162 [10] | |
4 | 4-BrC6H4CHO | 2-ABT | 50 | 75 | 120.9–122 | 120–121 [12] | |
5 | 4-Cl2C6H4CHO | 2-ABT | 60 | 73 | 116 | 115–117 [10] | |
6 | 2,4-Cl2C6H3CHO | 2-ABT | 65 | 80 | 140–143 | 144–145 [15] | |
7 | 2,6-Cl2C6H3CHO | 2-ABT | 100 | 65 | 89–91 | 87–90 [16] | |
8 | C6H4CHO | 2-ABT | 100 | 70 | 112–114 | 110–112 [18] | |
9 | CHOC6H4CHO | 2-ABT | 140 | 63 | 198 | 196 [20] |
a Reaction conditions: Aromatic aldehyde (1.0 mmol), 2-Aminobenzothiazol (2-ABT) (1.0 mmol), catalytic amount of Fe3O4@SiO2/collagen (50 mg) and 4 mL of EtOH at reflux.
2.9 Selected spectral data
2.9.1 4-(1H-Benzo[d]imidazol-2-yl) benzonitrile (Table 2, entry 5)
Yellow powder (90%): mp 261 °C. IR (KBr) (υmax, cm−1): 3533, 2231, 1658, 1315. 1H NMR (400 MHz, DMSO-d6): δH (ppm) 13 (s, 1H, NH), 8.3–8.4 (d, 2H, ArH), 8 (d, 2H, ArH), 7.7 (d, 1H, ArH), 7.6 (d, 1H, ArH), 7.2 (m, 2H, ArH).
2.9.2 2-Phenylbenzothiazole (Table 3, entry 8)
Mp: 112–114 °C. IR (KBr): (υmax, cm−1) 3064, 1588, 1555, 1509, 1478, 1433, 1244, 962, 766. 1H NMR (400 MHz, DMSO-d6): δH (ppm) 7.41–8.08 (m, 9H, ArH).
3 Results and discussions
3.1 Characterization of Fe3O4@SiO2/collagen
The EDX spectrum of Fe3O4@SiO2/collagen as an exact elemental analysis is depicted in Fig. 1a. As could be seen, existence of Fe, Si, O, C and some of the other atoms in the structure of Fe3O4@SiO2/collagen is proved. In addition, it shows that the molar ratios of Fe:Si:C are 23:21:6. The FT-IR spectrum of the as-synthesized Fe3O4@SiO2/collagen in comparison to that of raw Fe3O4, Fe3O4@collagen and SiO2/collagen is shown in Fig. 1b and these spectra can be compared to the purified collagen spectrum [35,36]. Referring to FT-IR spectra, the presence of the strong FeO and SiO stretching bonds is confirmed. These bonds appear at around 530 and 1050 cm−1, respectively. Moreover, strong and wide peaks appear at approximately 3300 and 3500 cm−1 and a weak peak at around 2900 cm−1 in FT-IR spectra of the catalyst. These wave numbers prove the existence of NH, OH and CH aromatics. Regarding further hydrogen interaction of the end tails of collagen fibres with OH and NH moieties, their appeared peaks are broader and stronger than weak peaks of CH bonds.

a) EDX, b) FT-IR and c) XRD patterns of Fe3O4@SiO2/collagen.
XRD patterns were used to determine the crystalline structure of nanocatalysts. The XRD pattern of the as-prepared nanocatalyst in comparison to that of pure Fe3O4 nanoparticles is shown in Fig. 1c. As can be seen in the XRD patterns, the position and relative intensities of appeared peaks in the catalyst are similar to those of bare Fe3O4 nanoparticles. This result confirms the presence of the Fe3O4 crystalline structure in the final product.
Fig. 2a and b show the internal and surface morphology of the as-synthesised catalyst that was studied by TEM and SEM images. The TEM image proves the homogeneous spherical core@-shell morphology of Fe3O4@SiO2/collagen. In addition, the distribution diagram of the nanocatalyst diameter is shown in Fig. 2c. These diagrams were obtained by measuring 150 separated particles and show that the average particle size is 39 nm, while about 90% of particles are in the size range of 26–53 nm. Also, this evaluation shows that Fe3O4 cores were about 16–25 nm. According to these results, approximately 9–14 nm homogeneous shell covers the surface of the Fe3O4 internal core.
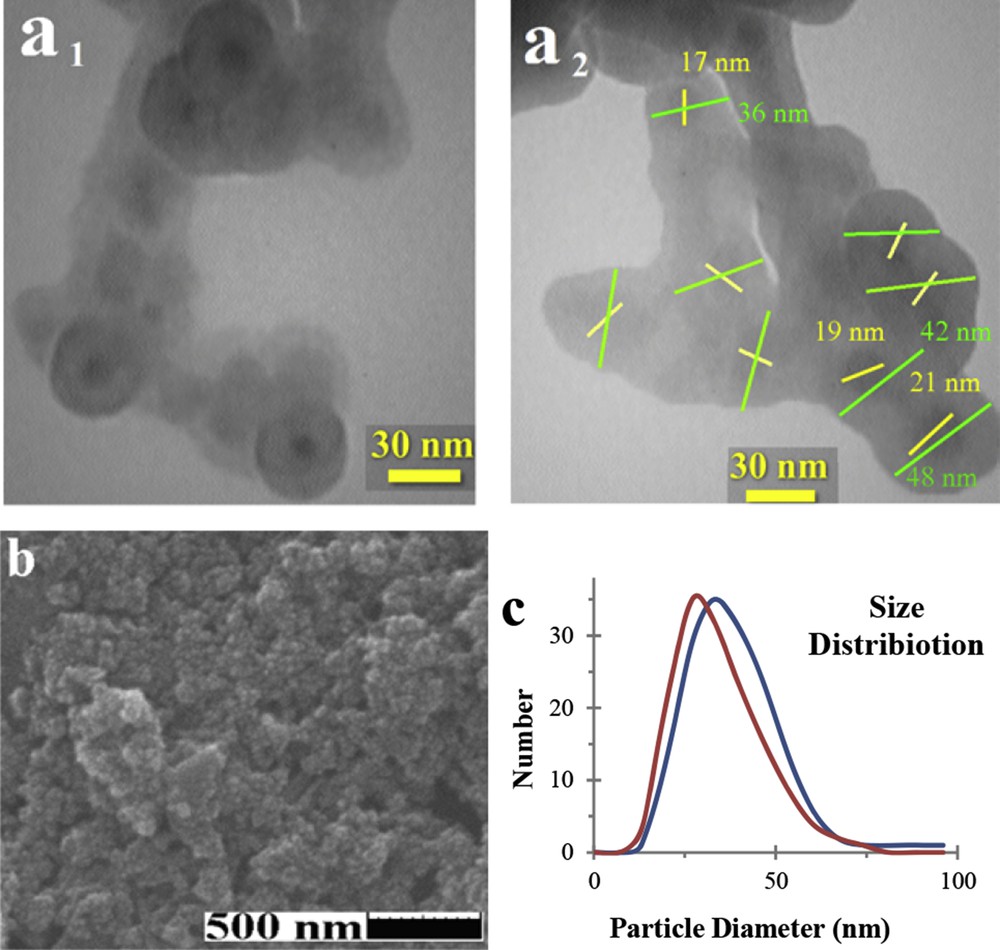
a) TEM (a1 and a2), b) and SEM images of Fe3O4@SiO2/collagen. c) Diagram of distribution particle size by SEM (red) and TEM (blue) images.
One of the important advantages of Fe3O4 nanoparticles is superparamagnetic properties which can be separated by external magnetic fields. These unique magnetic properties are due to the configuration of valance electrons in subatomic orbital clouds and arrangement of unique crystalline domains in Fe3O4 nanoparticles. Magnetic properties of the as-synthesised Fe3O4 were evaluated by VSM analysis. In VSM analysis, high magnetization saturation and low magnetic fields were coercively ascribed to superparamagnetic behaviour of samples. As shown in Fig. 3, magnetization saturation of Fe3O4 nanoparticles, Fe3O4@collagen and Fe3O4@SiO2/collagen reduced respectively. Probably, these reductions were affected by establishment of deterrent shells on the surface of Fe3O4 nanoparticles.
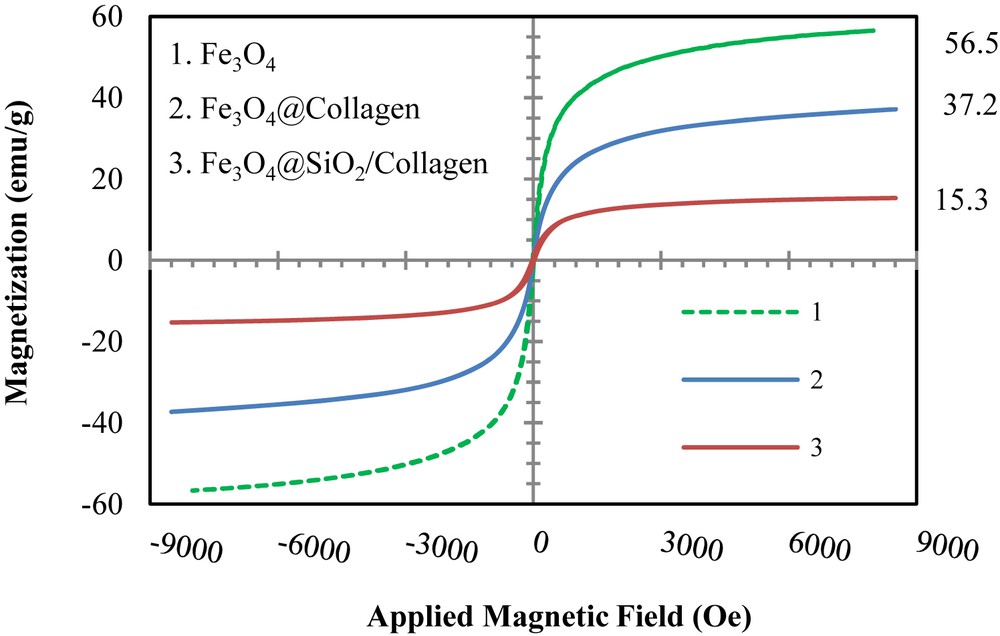
VSM analysis of 1) Fe3O4, 2) Fe3O4@collagen and 3) Fe3O4@SiO2/collagen
These results confirmed that Fe3O4@SiO2/collagen with a core@shell structure and high superparamagnetic properties has been successfully synthesized.
3.2 Catalytic application of Fe3O4@SiO2/collagen in organic synthesis
To investigate the catalytic activity, the synthesized Fe3O4@SiO2/collagen was used for the preparation of benzimidazole and benzothiazole compounds as model reactions. Initially, Fe3O4@SiO2/collagen as the nanocatalyst was used for the synthesis of benzimidazole and its derivatives in the presence of 1,2-phenylendiamine and 3-nitrobenzaldehyde (1:1 molar ratio) (Scheme 1).

General procedure for the synthesis of benzimidazole (X:N) and benzothiazole (X:S).
Table 1 shows optimization conditions with different solvents and catalysts of this reaction. Some suggested solvents such as EtOH, CH2Cl2, H2O in other literature studies were tested in the model synthesis. The results revealed that EtOH is the best solvent for the reaction. Consequently, the effect of the catalyst on the time and yield of reaction was optimized and the best result was obtained with 50 mg/mmol of Fe3O4@SiO2/collagen. In the next step, structural properties of Fe3O4@SiO2/collagen were compared with those of similar nanostructures (Scheme 2).
Optimization of the catalysts and solvents for synthesis of benzimidazole.a
Entry | Type & amount of catalystb | Solvent | Time (min) | Yield (%) |
1 | Non-additive | EtOH | 1080 | 25 |
2 | 50 (Collagen) | H2Oc | _ | _ |
3 | 50 (Collagen) | EtOHd | 50 | 85 |
4 | 50 (Fe3O4) | EtOH | 240 | 30 |
5 | 50 (Fe3O4@collagen) | EtOH | 20 | 96 |
6 | 50 (SiO2@collagen) | EtOH | 25 | 95 |
7 | 50 (Fe3O4@SiO2) | EtOH | 150 | 53 |
8 | 10 (Fe3O4@SiO2/collagen) | EtOH | 20 | 68 |
9 | 50 (Fe3O4@SiO2/collagen) | EtOH | 20 | 97 |
10 | 80 (Fe3O4@SiO2/collagen) | EtOH | 20 | 56 |
11 | 160 (Fe3O4@SiO2/collagen) | EtOH | 20 | 30 |
12 | 50 (Fe3O4@SiO2/collagen) | EtOH | 15 | 97 |
13 | 50 (Fe3O4@SiO2/collagen) | H2O | 15 | Trace |
14 | 50 (Fe3O4@SiO2/collagen) | CH2Cl2 | 15 | 80 |
15 | 50 (Fe3O4@SiO2/collagen) | CHCl3 | 15 | 49 |
16 | 50 (Fe3O4@SiO2/collagen) | CH3CN | 15 | – |
17 | 50 (Fe3O4@SiO2/collagen) | Solvent free | 15 | – |
a Benzimidazoles synthesis conditions: 1 mmol 1,2 phenylendiamine, 1 mmol 3-nitrobenzaldehyde, 4 mL solvent at rt.
b Catalyst (mg).

Type of catalyst that was tested in organic synthesis.
Collagen and hydrolysed collagen have high intumescent affinity and get swollen when they were used in aprotic and aqueous media [36,37]. Then, they cannot be used directly as catalysts and should be loaded and/or stabilized on a nanostructure substrate.
Collagen inflates in H2O and the reaction cannot be surveyed. So, it was loaded on the surface of Fe3O4.
In the surveyed synthesis methods, Fe3O4@collagen has shown efficient catalytic and magnetic properties. In this case, collagen was loaded on the surface of Fe3O4 nanoparticles but it cannot be properly stabilized on them. Thus, some of the unconsolidated collagen was removed, regardless of the loaded collagen amount on the catalyst, after each run, collagen content was reduced and reaction yields decreased. Therein, it does not have good reusability in comparison to Fe3O4@SiO2/collagen (see Fig. 4a). SiO2/collagen is a stable nanocatalyst and shows the same reactivity in synthesis method, but it does not have convenient magnetic properties for use as a magnetic recoverable nanocatalyst. In the next experiment, the catalytic reactivity of Fe3O4 and Fe3O4/SiO2 was compared with that of Fe3O4@SiO2/collagen as the main catalyst. The results show that collagen-less magnetic nanocatalysts do not have remarkable catalytic reactivity in comparison to collagen containing catalysts. Fe3O4@SiO2/collagen has both stability and superparamagnetic properties that facilitate magnetic recovery after each run. To expand this method Fe3O4@SiO2/collagen was used in the synthesis of benzimidazole and benzothiazole derivatives and the results are summarized in Tables 2 and 3, respectively.
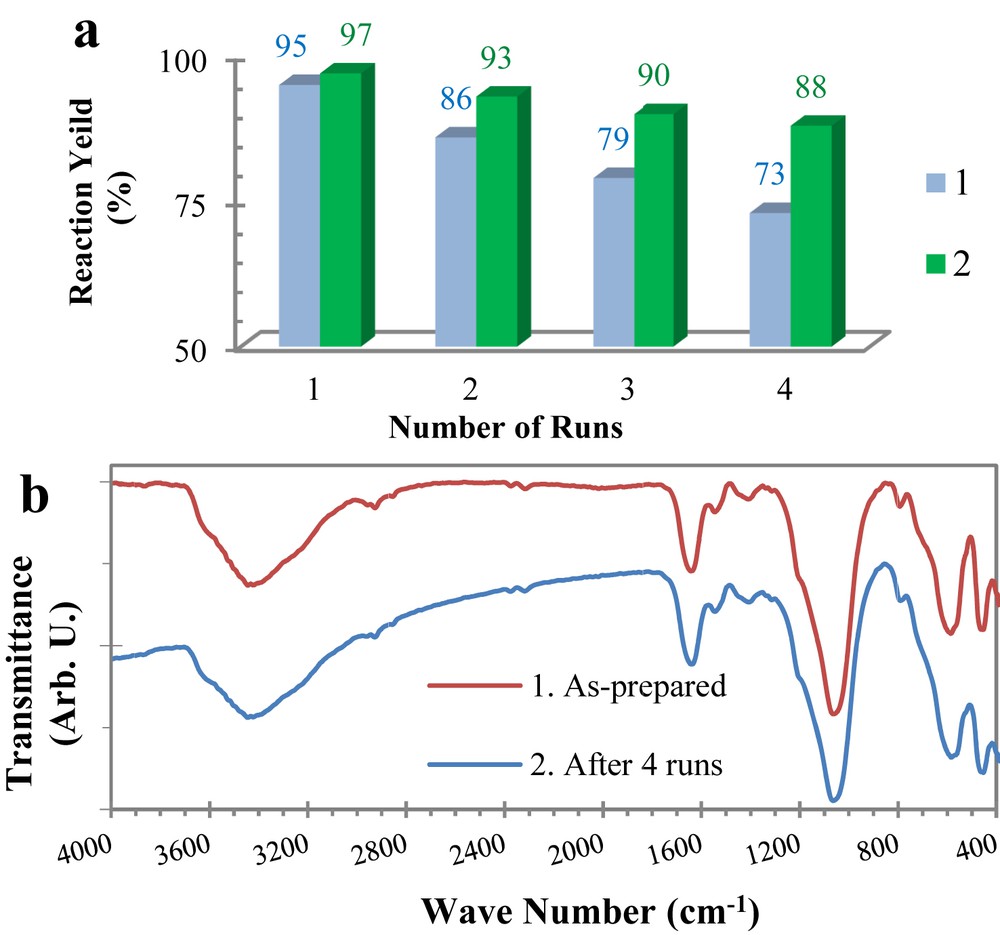
a) Nanocatalyst reactivity for the synthesis of entry 2 in Table 2; 1) Fe3O4@Collagen and 2) Fe3O4@SiO2/Collagen; b) FT-IR spectra of 1) as-prepared Fe3O4@SiO2/Collagen and 2) after four catalytic runs.
One of the important advantages of the Fe3O4@SiO2/collagen catalyst is its easy and reliable separation from the reaction mixture. Then, the reusability of this nanocatalyst was investigated in the benzimidazole model reaction. The heterogeneous nature and magnetic properties of the catalyst allowed its facile recovery via a simple separation process by an external magnet. The collected catalyst was rinsed with EtOH and dried at room temperature for reuse in other reactions. The separated nanocatalyst was reused in the mentioned reaction for the synthesis of entry 2 in Table 2 four times without considerable loss of its catalytic reactivity. The catalytic reactivity of reused Fe3O4@SiO2/collagen in comparison to that of Fe3O4@collagen is depicted in Fig. 4a. In addition, FT-IR spectra of the recovered catalyst after four runs in comparison with those of the as-synthesized Fe3O4@SiO2/collagen are depicted in Fig. 4b. As could be seen, there are no obvious differences in the FT-IR spectra of the as-prepared and recovered nanocatalyst. These appearances confirm the stability of the nanocatalyst under the reaction conditions.
Due to the mentioned benefits and as could be compared by the literature, Fe3O4@SiO2/collagen is one of the best heterogeneous nanocatalysts for the synthesis of benzimidazole and benzothiazole derivatives.
In order to indicate the efficiency of our method it was compared with that of some other reported methods and the results are summarized in Table 4. The results clearly show the high efficiency of Fe3O4@SiO2/collagen in the synthesis of benzimidazole. Also, Table 5, shows the efficiency of our synthesized nanocatalyst in the preparation of benzothiazole compared to that of other reported methods.
A comparison of the efficiency of Fe3O4@SiO2/collagen with that of other catalysts in the synthesis of benzimidazole.a
Year | Catalyst | Solvent | Temperature (°C) | Time (min) | Yield (%) |
2006 | Zeolite | Dioxane | 100–102 | 300 | 69 [50] |
2010 | Scolecite | EtOH | 70 | 45 | 91 [41] |
2013 | Nano-ZnO | EtOH | Reflux | 95 | 68 [18] |
2013 | Nano-alumina | EtOH | Reflux | 90 | 74 [18] |
This Work | Fe3O4@SiO2/collagen | EtOH | rt | 12 | 78 |
a All data compared for entry 2 in Table 2.
A comparison of the efficiency of Fe3O4@SiO2/collagen with that of other catalysts in the synthesis of benzothiazole.a
Year | Catalyst | Solvent | Temperature (°C) | Time (min) | Yield (%) |
2006 | CH3COOH | CH3COOH | Reflux | 300 | 65 [33] |
2013 | Nano-ZnO | EtOH | Reflux | 80 | 72 [18] |
2014 | FeCl3·H2O K2S2O8 | DMSO/H2O | 100 (oC) | 800 | 41 [22] |
This Work | Fe3O4@SiO2/collagen | EtOH | Reflux | 50 | 75 |
a All data compared for entry 3 in Table 3.
The suggested mechanism for the synthesis of benzimidazole and benzothiazole derivatives is proposed in Scheme 3. The surface of collagen in Fe3O4@SiO2/collagen has a variety of functional groups which can form hydrogen bonds with protic moieties. In addition, collagen fibres with organic chains can interact with aprotic moieties of organic compounds. This quality has the main role in the reaction times and reaction yields in reactions with collagen containing nanocatalysts [51–53].
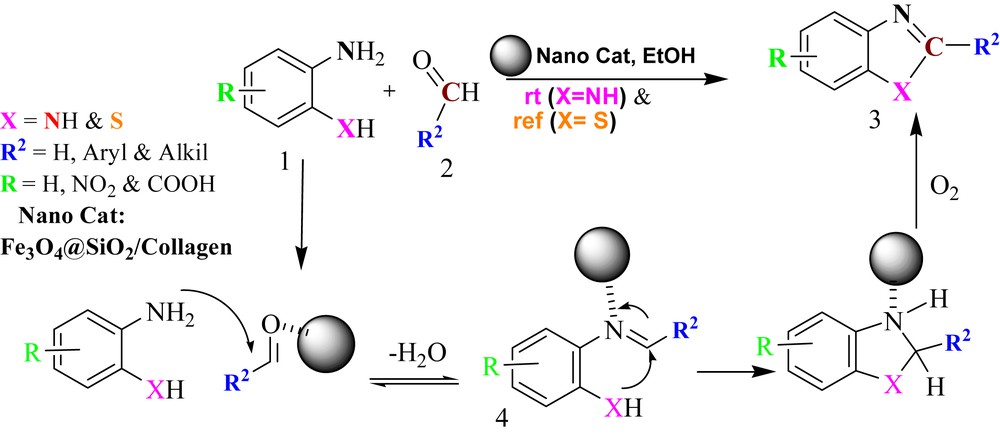
The proposed mechanism for the formation of benzimidazole and benzothiazole derivatives.
In aspect of green chemistry principles, Fe3O4@SiO2/collagen is a new nanocatalyst with some interesting advantages such as high magnetic properties, good selectivity (Scheme 4), easy separation and high yield in the synthesis of heterocyclic compounds.

Selectivity of Fe3O4@SiO2/collagen in the synthesis of benzimidazole derivatives.
4 Conclusions
In this work, after synthesis of Fe3O4@SiO2, it was combined with collagen for the preparation of Fe3O4@SiO2/collagen and characterization was done by various instrumental methods. Remarkable advantages of this nanocatalyst are the facile synthesis procedure, high catalytic activity, easy separation and acceptable reusability. This nanocomposite as an efficient nanocatalyst was used for the synthesis of benzimidazole and benzothiazole derivatives due to its excellent properties and high selectivity. Also, this procedure has some benefits such as the use of harmless solvents, facile conditions, easy isolation of the products with excellent purity, increasing reaction yields, reducing reaction time and waste-less chemical process.
Acknowledgements
The authors gratefully acknowledge the partial support from the research council of the Iran University of Science and Technology (IUST).