1 Introduction
Benzophenone-3 (BP-3) is commonly used in UV filters, which has a great ability to absorb and dissipate UV irradiation. A large panel of personal care products contains BP-3 that protects humans and materials from harmful exposure to UV irradiation [1–3]. With an increasing use of UV filters, BP-3 has been released as an effluent into aquatic environment by the direct discharge from the UV filters industry and the indirect discharge from municipal wastewater, thus leading to a potential threat to aquatic environment and ecology. It has been detected in rivers, lakes, oceans and even in tap water at concentrations between several ng/L and μg/L [4–9]. Conventional wastewater treatment technologies are usually adapted for the removal of BP-3 [10,11]. Hence, there is a need to remove BP-3 from industrial and municipal wastewater by applying various enhanced technologies. Due to the photo-stability under both UV and artificial solar light, BP-3 cannot be easily degraded by photolysis reactions [6,12]. Although BP-3 can be rapidly oxidized by free chlorine, it is also converted into mono- and di-halogenated by-products as well as several halogenated forms of 3-methoxyphenol, resulting in an increase of toxicity [13]. Therefore, it is urgent to find new approaches for the removal of BP-3.
Recently, advanced oxidation processes (AOPs) became promising technologies for the removal of refractory chemicals at an acceptable cost [14]. The hydroxyl radicals (˙OH) generated in AOPs play a key role in the degradation of organic pollutants at a fast reaction rate [15–20]. The Fenton reaction has been proven to be one of the most effective technologies to degrade organic pollutants in wastewater because of its simplicity, low price, availability, and small toxicity of its reagents [21,22]. However, there are some limitations: the use of ferric ions resulted in colored effluents, and the formation of iron sludge. In addition, the Fenton reaction works well only under highly acidic conditions (pH 2–3), which seriously hinders its application and raises the cost [23,24]. In order to overcome the drawbacks of the traditional Fenton reaction, many efforts have been made to develop heterogeneous catalysts free of iron [25–29].
In this study, we report a novel hybrid catalyst containing Cu1.4Mn1.6O4, Mn2O3 and Mn3O4 as an efficient catalyst for the degradation of BP-3 in a wide pH range (2.6–7.1) in the heterogeneous Fenton-like reaction. The influence of some key parameters including the Cu/Mn molar ratio in the synthesis procedure, initial pH, and titration of H2O2 was systematically evaluated. Reactive radicals generated during the reaction were also investigated by using a radical quencher.
2 Experimental section
2.1 Synthesis of samples
The catalyst precursor was synthesized by a traditional co-precipitation method. In brief, a salt solution (100 mL) containing a mixture of Cu(NO3)2·3H2O and Mn(NO3)2 was added dropwise to a Na2CO3 aqueous solution (100 mL). The pH of the precipitation solution was controlled at ∼10.0 using a NaOH (4 M) solution. The resultant solution was transferred to a Teflon-lined stainless steel autoclave and heated at 150 °C for 18 h. The as-obtained precipitate was filtered and rinsed with deionized water and acetone, followed by drying at 60 °C for 24 h. After careful grinding, the as-prepared samples were calcined at 500 °C for 5 h at a heating rate of 10 °C/min.
2.2 Characterization of samples
X-ray diffraction (XRD) measurements of the catalyst powders were recorded using a Shimadzu XRD-7000 instrument in the reflection mode with Cu Kα radiation. The accelerating voltage was set at 40 kV with 30 mA current (λ = 1.542 Å) at 0.1°s−1 from 5 to 65°. Field emission scanning electron microscopy (FE-SEM) and energy-dispersive X-ray spectroscopy (EDX) analyses were performed on an SU-8010 scanning electron microscope (SEM, HITACHI, Japan) with an accelerating voltage of 5.0 kV. Powdered samples were spread on a carbon tape and then coated with a thin platinum layer to prevent charging and improve the image quality.
2.3 Fenton-like reaction procedure
The adsorption or oxidation of BP-3 was carried out in a 300 mL glass reactor containing 250 mL BP-3 solution (2.0 mg/L). In the experiments, certain amounts of catalysts (100 mg/L) and H2O2 (0.5–3.0 mM) were added to the BP-3 solution to start the Fenton-like reaction. About 3.0 mL samples were taken out and immediately filtered every 30 min. Before the addition of the catalyst, the initial solution pH was adjusted using nitric acid and/or sodium hydroxide solution. The residual concentration of BP-3 was measured by Waters2695 (Waters, USA) performance liquid chromatography (HPLC) equipped with a UV detector at 288 nm. The separation was performed with an Agilent C18 column (150 mm × 4.6 mm, 5 μm, Agilent, USA) using an isocratic elution of 40% buffered solution (H2O/H3PO4 = 1000/0.06, v/v) and 60% CH3CN. The elution flow rate was 1.0 mL/min and the injection volume was 30 μL for all samples.
3 Results and discussion
3.1 Synthesis and characterization of samples
Fig. 1(a) shows the XRD patterns of samples synthesized with different Cu/Mn molar ratios before calcination. When the quantity of manganese was superior to the loading and the molar ratio of Cu/Mn was 0.05 and 0.2, a hybrid phase of MnCO3 and Mn3O4 was obtained. With the Cu/Mn molar ratio increased to 0.5, the Mn3O4 phase disappeared and a new CuMnO2 structure was formed. However, the phase of the sample transformed to pure MnCO3 at Cu/Mn = 1.0. By further increasing the ratio of Cu/Mn to 5, the characteristic peaks of CuO were observed at 2θ = 32.50°, 35.54°, 38.70°, 48.71°, 58.26°, and 61.52°, respectively. Fig. 1(b) shows the XRD patterns of all the samples after calcination at 500 °C for 5 h. With the Cu/Mn ratio of 0.05, the sample was composed of both Mn2O3 (JCPDS No. 24-0508) and Mn5O8 (JCPDS No. 39-1218). Besides, the diffraction peaks at 2θ = 35.78°, 43.96°, and 63.93° might be attributed to Cu4O3 (JCPDS No. 49-1830). However, when the ratio of Cu/Mn increased to 0.2, a new hybrid compound of Cu1.4Mn1.6O4, Mn2O3 and Mn3O4 was obtained. The characteristic reflections of Cu1.4Mn1.6O4 were detected at 2θ = 18.49°, 30.42°, 35.83°, 57.60°, and 63.26°. Furthermore, the diffraction peaks at 2θ = 28.88°, 44.44°, and 64.65° might be attributed to Mn3O4 (JCPDS No. 24-0734). Further raise in the Cu/Mn molar ratio to 0.5 led to the vanishing of the Mn2O3 contribution. When a higher Cu/Mn ratio of 1.0 was used, pure Cu1.5Mn1.5O4 was obtained. As the ratio increased to 5.0, CuO (JCPDS No. 48-1548) emerged in the hybrid sample.
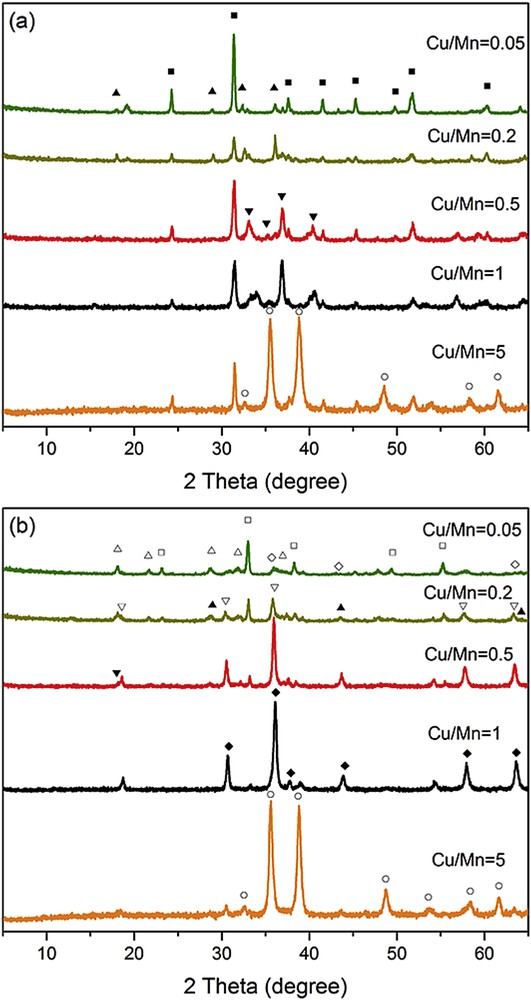
XRD patterns of samples synthesized with different Cu/Mn ratios. (a) before calcination, (b) after calcination, (■) MnCO3, (▴) Mn3O4, (▾) CuMnO2, (○) CuO, (□) Mn2O3, (▵)Mn5O8, (⋄) Cu4O3, (▿) Cu1.4Mn1.6O4, and (♦) Cu1.5Mn1.5O4.
The samples were further characterized by FE-SEM and EDX. Fig. 2 highlights a Cu/Mn ratio increased from 0.05 to 5.0, allowing us to produce all samples in the form of nanoparticles. Surprisingly, nanofibers were also found in the sample synthesized with the Cu/Mn molar ratio as 0.2. Elemental mapping (Fig. 3(b–d)) of Cu, Mn, and O clearly indicated that Cu, Mn, and O were uniformly distributed. This data were also confirmed by the EDX result in certain selected areas in Fig. 4, which demonstrated that the chemical composition of nanoparticles and nanofibers remained similar. The phase structure and morphology of the as-synthesized samples may have an influence on the performance of the Fenton-like reaction.
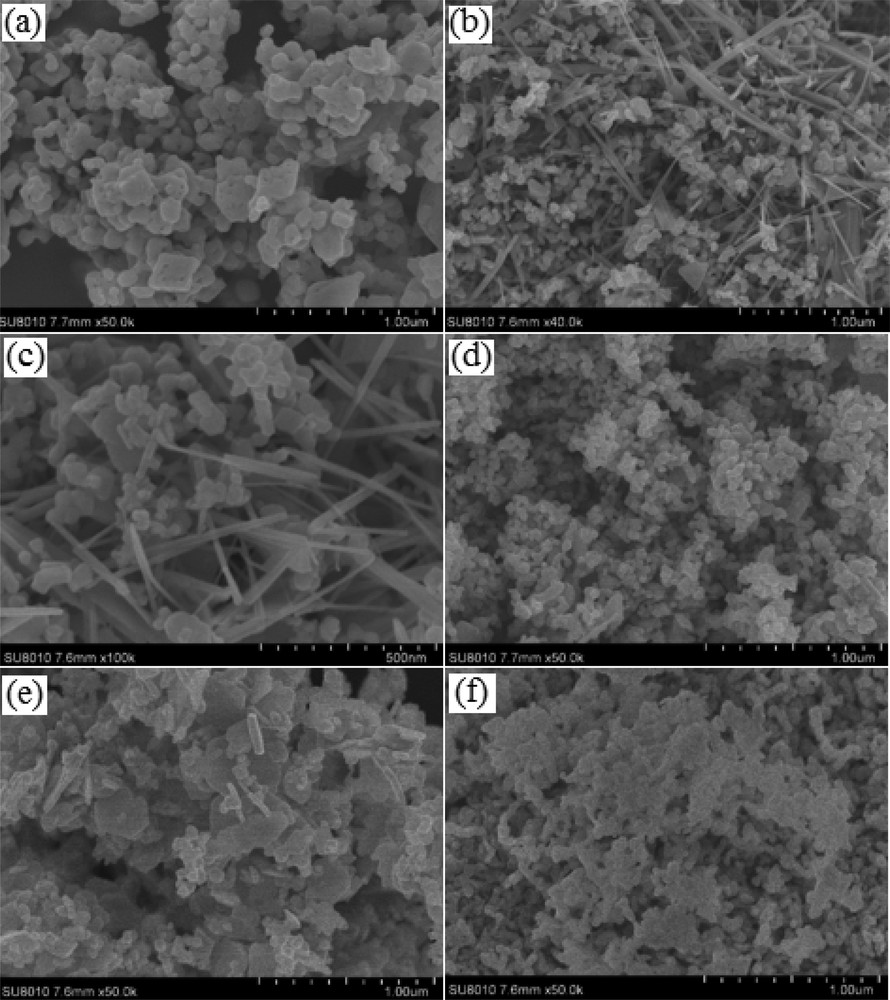
SEM images of samples synthesized with different Cu/Mn ratios (calcined at 500 °C for 5 h). (a) Cu/Mn = 0.05, (b) Cu/Mn = 0.2, (c) the high-resolution SEM of Cu/Mn = 0.2, (d) Cu/Mn = 0.5, (e) Cu/Mn = 1.0, and (f) Cu/Mn = 5.0.
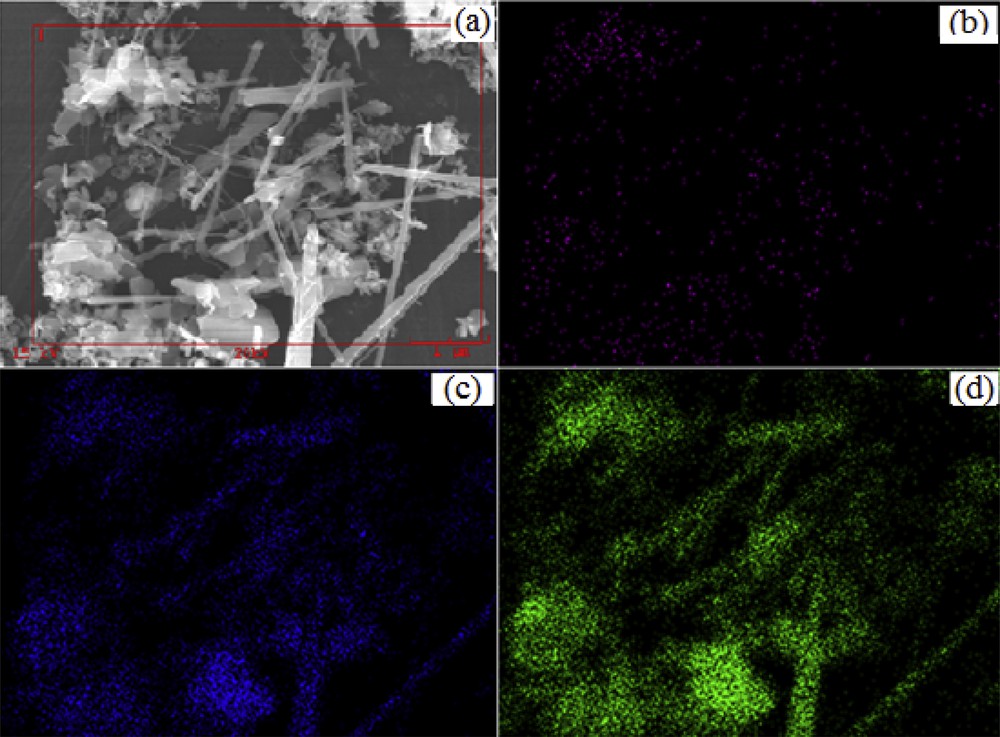
(a) SEM image of sample synthesized with a Cu/Mn molar ratio of 0.2. Elemental (b) Cu mapping, (c) Mn mapping, and (d) O mapping of the synthesized sample.

SEM-EDX analysis of the sample synthesized at Cu/Mn = 0.2. (a, b) Nanoparticles and (c, d) nanofibers.
3.2 Degradation of BP-3 in the Fenton-like reaction
3.2.1 Influence of the Cu/Mn ratio on BP-3 removal
Since the Cu/Mn molar ratio has a significant effect on the chemical composition and morphology of the samples, further studies were carried out to test their catalytic activities in BP-3 degradation. Without any catalyst addition, the removal of BP-3 was very low, only 39.3% of BP-3 could be decomposed after 240 min. As shown in Fig. 5, the removal efficiency of BP-3 increased while raising the Cu/Mn ratio at first (before 0.2) and then diminished. When the catalyst was composed of Mn2O3, Mn5O8 and Cu4O3 (Cu/Mn = 0.05), the degradation efficiency of BP-3 reached 73%. Such manganese and copper oxides have been proved to enhance the generation of reactive radicals, which are able to promote the degradation efficiency of pollutants in such reactions [30–32]. The catalyst synthesized with Cu/Mn = 0.2 with a hybrid phase composition of Cu1.4Mn1.6O4, Mn3O4, and Mn2O3 was observed to exhibit the highest activity, thus leading to a degradation efficiency of 81.5% within 240 min of the reaction. When the Cu/Mn molar ratio was raised to 0.5, the Mn2O3 phase disappeared and the oxidation efficiency decreased to 75.0%. With further increasing the molar ratio of Cu/Mn to 1.0 and 5.0, the catalytic activity diminished to 73.3% and 74.6%, respectively, with the phase composition of Cu1.5Mn1.5O4 and CuO. It can be concluded that Mn (III) oxides greatly enhanced the generation of radicals, which play a crucial role in the improvement of the catalytic activity of Cu–Mn–O4. Comparing to manganese oxides, the effect of copper oxides on the improvement of the catalytic activity of Cu–Mn–O4 in the Fenton-like reaction was not significant.

The influence of different molar ratios of Cu/Mn on the degradation of BP-3 in the Fenton-like reaction. Reaction conditions: [BP-3]0 = 2.0 mg/L, [H2O2]0 = 1.0 mM, [catalyst]0 = 100 mg/L, and pH = 7.0.
In addition to the effect of the chemical composition, the catalytic activity might also be affected by their particle morphologies. Indeed, all catalysts were observed as nanoparticles except the hybrid catalyst synthesized with Cu/Mn = 0.2, which was constituted of nanoparticles and nanofibers. To clarify how the morphology change influences the catalytic activity, much more work is needed.
3.2.2 Influence of the initial solution pH on BP-3 removal
Since the pH of the solution affects the H2O2 decomposition rate, the distribution of BP-3 species, and the surface charge of the catalyst, the effect of the initial solution pH on the performance of BP-3 degradation was investigated. Fig. 6 clearly demonstrates that the highest degradation efficiency of BP-3 was obtained at a pH around 7.1 (81.5% after 240 min). Interestingly, the influence of the initial solution pH (from 2.6 to 7.1) on the final performance and observed degradation rate constant was less than 12.7%. To the best of our knowledge, a traditional Fenton reaction system works well only under highly acidic conditions (pH < 4.0), which was one of the main drawbacks of this reaction system [33]. The catalyst synthesized at Cu/Mn = 0.2 showed high catalytic activity at a wide pH range, which made the successful application of this effective Fenton-like reaction for a real wastewater treatment.
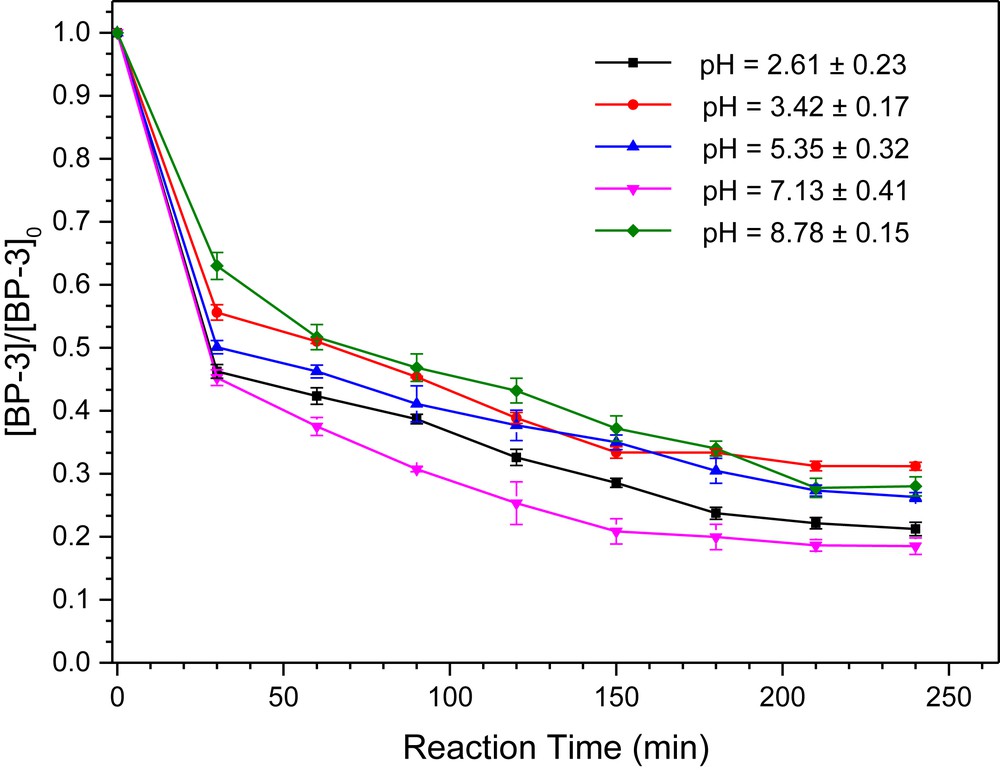
The influence of the initial solution pH on the degradation BP-3 by the sample synthesized at Cu/Mn = 0.2. Reaction conditions: [BP-3]0 = 2.0 mg/L, [H2O2]0 = 1.0 mM, [catalyst]0 = 100 mg/L, and the initial solution pH was adjusted by HNO3 and NaOH.
Moreover, the oxidation of BP-3 with different initial solution pH can be fitted by pseudo-first-order kinetics model described by the following equations (Eqs. 1–4), as [H2O2] >> [BP-3] in this study.
(1) |
(2) |
(3) |
(4) |

Variation of the pseudo-first-order rate constant as a function of the initial solution pH for BP-3 degradation. Reaction conditions: [BP-3]0 = 2.0 mg/L, [H2O2]0 = 1.0 mM, and [catalyst]0 = 100 mg/L.
3.2.3 Influence of the H2O2 quantity on BP-3 removal
In order to test the role of surface adsorption in this Fenton-like reaction, a pre-adsorption experiment was designed and carried out as well (Fig. 8). Only the catalyst with Cu/Mn = 0.2 was added in the first 30 min, and the adsorption efficiency was only 7.9% after 30 min. The pKa value of BP-3 is 9.57 [34], leading to the formation of BP-3− and BP-3 species under water pH = 7.0. The presence of BP-3− will lead to a surface adsorption on Cu–Mn–O by electrostatic attraction. The catalytic oxidation process can be observed by adding H2O2 to the reaction system. The degradation efficiency significantly increased to 89.0% after 240 min. This experiment clearly indicated that BP-3 adsorption on the catalyst was not obvious and its significant degradation came from the catalytic oxidation in the presence of H2O2. Moreover, at the beginning 150 min of H2O2 addition, the catalyst exhibited less catalytic activity for BP-3 removal, compared with the process without pre-adsorption. This phenomenon can be explained by the fact that BP-3 is firstly absorbed on the catalyst surface, which affected the mass transfer of H2O2 and hindered the Fenton-like reaction. As the oxidation proceeded, the surface adsorbed BP-3 was degraded, and dissolved BP-3 would be gradually adsorbed and then degraded by the Fenton reaction.

The influence of pre-adsorption and different initial H2O2 concentrations on the degradation of BP-3 by the sample synthesized at Cu/Mn = 0.2. The inset shows the variation of the pseudo-first-order rate constant as a function of the H2O2 concentration for BP-3 degradation. Reaction conditions: [BP-3]0 = 2.0 mg/L, [catalyst]0 = 100 mg/L, and pH = 7.0.
The influence of the H2O2 concentration on the degradation of BP-3 was also investigated. The experimental results revealed a faster and higher BP-3 removal at higher H2O2 concentrations. The pseudo-first-order rate constant (kobs) showed a linear relationship with H2O2 concentrations (inset of Fig. 8), which can be attributed to more reactive radicals generated from H2O2 in the aqueous medium. The results of over dosing H2O2 or ˙OH quenching by H2O2 were not obtained in this study.
3.2.4 Influence of the hydroxyl free radical on BP-3 removal
In order to better understand the role of ˙OH in BP-3 degradation, tert-butanol (TBA) that can quench ˙OH [35] was added into the reaction system. According to Fig. 9, when the TBA dose was 50 mg/L, the removal rate decreased to 71.5%, suggesting that the presence of ˙OH accounted for the oxidation of BP-3. By further increasing the concentration of TBA to 250 mg/L, the trend of BP-3 concentration variation was not significantly modified, indicating that the dose of TBA quenched all ˙OH generated in this Fenton-like reaction. Hence, ˙OH produced in the Fenton-like reaction might play a crucial role in the catalytic oxidation of BP-3.
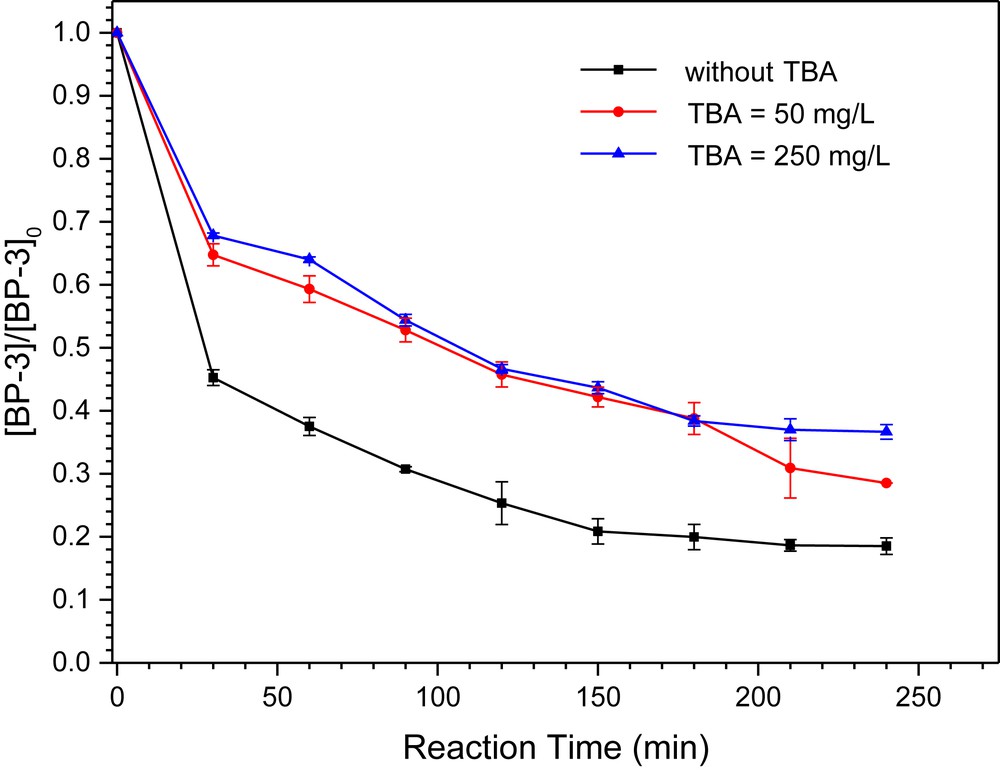
The influence of TBA on the degradation BP-3 by the sample synthesized at Cu/Mn = 0.2. Reaction conditions: [BP-3]0 = 2.0 mg/L, [H2O2]0 = 1.0 mM, [catalyst]0 = 100 mg/L, and pH = 7.0.
4 Conclusions
In conclusion, a novel hybrid Cu–Mn–O catalyst was synthesized using a co-precipitation and hydrothermal method, which showed high catalytic activity on the degradation of BP-3 in the heterogeneous Fenton-like reaction. XRD and SEM analyses suggest that this hybrid Cu–Mn–O catalyst (Cu/Mn = 0.2) consists of Cu1.4Mn1.6O4, Mn3O4, and Mn2O3, with a morphology containing both nanoparticles and nanofibers. The catalytic activity, the chemical composition, and the morphology of catalyst were highly dependent on the Cu/Mn ratio in the synthesis. The influence of the solution pH on the final performance and the observed degradation rate constant was less than 12.7%, suggesting that the heterogeneous Fenton-like reaction with this novel hybrid Cu–Mn–O catalyst was less sensitive to solution pH values. Since this hybrid Cu–Mn–O catalyst is applicable in a much wider pH range (2.6–7.1) than conventional Fenton systems, it is very promising for practical wastewater treatment systems. The dose of H2O2 was demonstrated to be good for raising the removal efficiency of BP-3, and the reaction was verified to be dominated by ˙OH radicals.
Acknowledgements
This work was carried out with the support of the Fundamental Research Funds for the Central Universities (BLYJ201615 and 2016ZCQ03) and the National Natural Science Foundation of China (51578520, 51572029, and 51308045).