1 Introduction
The worldwide cosmetic industry has largely developed over the past decade (worth expected to reach $390 billion by 2020; [1]) and its solid growth continues to be driven essentially by consumers expecting increasingly innovative products, as well as efficacy and quality. However, rising awareness about personal health and safety also led the consumers to look forward to safer cosmetics free of harmful chemicals. Hence, the dramatically increasing global trade in natural ingredients sourced from vegetable, mineral or marine renewable sources has perfectly illustrated this important trend in the last years. The predominance of such natural ingredients is largely due to the various roles they can play in a single cosmetic formula, acting as active ingredients (e.g., moisturizers), excipients (e.g., surfactants), additives (e.g., preservatives), etc., the last ones being particularly sought for by cosmetic manufacturers.
Microbial growth could occur in cosmetics and personal care products under usual manufacturing and usage conditions and numerous sources of contamination have been identified: the raw materials, the packaging, or even the manufacturing processes and environments that are often not sufficiently sterile. These products can also be exposed to temperature variations, and be notably subject to temperatures higher than recommended in shops and consumers' bathrooms, hence increasing the micro-organism growth rate. Furthermore, once the cosmetic or personal care product is opened, and until it is discarded, it is highly susceptible to further contamination due to consumer use (constant dipping of non-sterile fingers in the product, contact with non-sterile bodies, etc.). Micro-organisms can hence easily multiply and cause unpleasant discolouration and odour, and can degrade active compounds in products, leading to the instability of finished products [2,3]. Cosmetics and personal care products are hence expected to resist microbial attack within their intended period of use [4]: preservatives are then necessary to prevent product spoilage and subsequently consumers' infections caused by harmful microorganisms. Strict rules govern the inclusion of preservatives in cosmetics. Throughout Europe, manufacturers must carefully choose among preservatives listed in Annex V of Regulation (EC) [4] that have previously been subjected to scientific tests and approval procedures: every cosmetic formulation requires a tailor-made preservative system to meet its specific needs (selection of the appropriate nature and dosage of a specific preservative) [5].
Synthetic preservatives employed over decades such as p-hydroxybenzoic acid, phenoxyethanol and imidazolidinyl urea [3], display a whole lot of benefits including notably their more than affordable price, their broad-spectrum of activity against bacteria and fungi, their compatibility with other ingredients, and the fact that they generally do not interfere with fragrance, colour, or other aspects of a given formulation. However, although common synthetic preservatives do extend the lifespan of the products and help to keep them free from micro-organisms, many of them have developed negative reputations in consumers' minds and their use has become more and more controversial over the last years. In fact, often petroleum-based, they can be unhealthy and can notably promote irritations or infections, particularly on damaged skin, or the sensitive areas around the eyes [6]. Some of them can even cause higher health issues: 4-hydroxybenzoic acid esters, better known as parabens have been the most widely used preservatives (entering the composition of about 80% of the worldwide cosmetics; [7]), but have recently been reported to mimic oestrogen, to increase female breast cancer incidence and to influence the development of malignant melanoma [8]. Following the controversies about parabens and phenoxyethanol, cosmetic formulators are under increased pressure to turn more and more to innovative natural ingredients [7], because they are not only healthier alternatives for consumers but they also provide a wonderful marketing angle. In fact, the discovery and usage of a natural ingredient with preservative properties offers cosmetic industries the chance to communicate about the “preservative free” aspect of their products, as these new natural ingredients do not fall under the legislation and are hence not listed among preservatives in Annex V of Regulation (EC) [4]. Furthermore, if such a natural ingredient, besides its preservative action, also displays another interesting property, e.g., anti-oxidizing effect, the marketing communication opportunities will become even larger. Finally, rising consumer demand for products formulated with natural raw materials also offers cosmetic companies a chance to raise the awareness of consumers to biodiversity that becomes a strategic issue as a rich source for innovative molecules. Sourcing new natural ingredients engage cosmetic industries in a ‘green economy’ policy presided over by the respect of the principles of the Nagoya Protocol introduced on 29 October 2010, and issued from a number of implements of the original Convention on Biological Diversity (CBD) ratified in 1992 by 168 States at the United Nations' Earth Summit held in Rio de Janeiro [9]. The CBD constituted the first international document to recognize the urgent need to protect biodiversity, the sustainable use of its resources, and the equal and rightful sharing of the benefits it represents. Application of high throughput screening technologies to natural product samples accelerates the discovery, development and use of natural ingredients but such a screening is still a long-term undertaking as only five in every 100 genetic resources identified as being potentially of interest will ever end up in cosmetic and personal care formulae as they also have to pass all the efficacy, quality and safety tests right throughout the development chain [10].
Regions presenting high biodiversity and a high percentage of endemic flora and fauna that are especially endangered are recognized as biodiversity hotspots [11,12] and constitute protection priorities. With the generalized awareness of this richness threatened with extinction it appeared worth preserving this biodiversity and valuing plant species in the form of new ingredients intended notably for cosmetic and pharmaceutical industries. The Mediterranean region was identified as one of these biodiversity hotspots [12] due to its remarkable flora and specially its high rate of endemic species: representing only 2% of the world's surface, this area houses 20% of the world's total floristic richness [13,14]. This Mediterranean biome hence constitutes a choice target to source natural products intended both for cosmetics and drug design.
Considering this, 50 Mediterranean plants were initially selected from over 2000 for the present study, based on a literature survey and on the basis of their traditional use: 17 of them were accessible for further evaluation of their potential use as preservatives in cosmetics. A screening of their antimicrobial activities was engaged and the optimization of the most interesting crude extract was then investigated for practical cosmetic formulation.
2 Results and discussion
2.1 Antimicrobial activity screening
A screening of the antimicrobial activities of 17 of the 50 plants initially selected was undertaken to pinpoint plants presenting potential as cosmetic preservatives. For this, solvent crude extracts were prepared at respectively 2% (massic concentration in the raw extract; results displayed in Table 1) and 0.2% (data non shown): EtOH/H2O 75:25 (v/v) extracts were preferentially tested because such a solvent system is non-toxic and enables the extraction of a broad range of metabolites. Their antimicrobial activity was evaluated in compliance with the regulation against the yeast Candida albicans, the filamentous fungus Aspergillus niger and the bacteria Staphylococcus aureus and Pseudomonas aeruginosa, using 96-well microplate assays based on microbial growth inhibition assessed after respectively 24 and 48 h for yeast and fungus, and 48 and 72 h for bacteria. Tests were performed on several extracts from plants collected at different seasons over a 2–3 years period, and the results shown in Table 1 represent the mean activity of each extract.
Antimicrobial activity screening of Mediterranean plant extracts.
Plant name | Plant part | Localization | Extraction solvent | Antimicrobial activity at 2% | |||||||
P. aeruginosa | S. aureus | A. niger | C. albicans | ||||||||
48 h | 72 h | 24 h | 48 h | 48 h | 72 h | 24 h | 48 h | ||||
Cistus albidus L. | Twigs | 1 | 70% EtOH | − | ∼ | +++ | ++ | +++ | ∼ | ++ | ∼ |
Pistacia lentiscus L. | Twigs | 1 | 70% EtOH | ++ | ∼ | ++ | ++ | ∼ | − | − | − |
Salvia verbenaca L. | Aerial parts | 1 | 70% EtOH | − | − | ++ | − | + | − | − | − |
Ruta chalepensis L. | Aerial parts | 1 | 75% EtOH | − | − | +++ | +++ | ++ | + | ∼ | ∼ |
Coronilla emerus L. | Flowered aerial parts | 2 | 75% EtOH | − | − | ++ | − | ++ | + | − | − |
Laurus nobilis L. | Twigs | 1 | 75% EtOH | − | − | +++ | +++ | +++ | +++ | +++ | +++ |
Rosmarinus officinalis L. | Flowered branches | 4 | 75% EtOH | − | − | +++ | +++ | ∼ | ∼ | − | − |
Thymus vulgaris L. | Flowered aerial parts | 5 | 75% EtOH | − | − | +++ | +++ | +++ | ∼ | − | + |
Santolina chamaecyparissus L. | Flowered aerial parts | 2 | 75% EtOH | − | − | +++ | +++ | +++ | +++ | ++ | ∼ |
Juniperus oxycedrus L. | Twigs | 5 | 75% EtOH | ∼ | + | +++ | +++ | +++ | ∼ | + | ∼ |
Juniperus communis L. | Branches bearing fruits | 5 | 75% EtOH | − | − | +++ | +++ | + | − | − | − |
Myrtus communis L. | Flowered aerial parts | n.c. | 75% EtOH | − | − | ++ | +++ | ++ | − | + | ∼ |
Hypericum perforatum L. | Flowered aerial parts | n.c. | 75% EtOH | − | − | +++ | +++ | +++ | +++ | +++ | +++ |
Cupressus sempervirens L. | Twigs | n.c. | 75% EtOH | − | − | +++ | +++ | − | − | − | − |
Alliaria petiolata (M. Bieb.) Cavara & Grande | Aerial parts | 6 | 75% EtOH | − | − | +++ | +++ | − | − | − | − |
Helichrysum stoechas L. | Aerial parts | 7 | 75% EtOH | − | − | +++ | +++ | ∼ | − | − | − |
Satureja montana L. | Flowered aerial parts | 8 | 75% EtOH | − | − | − | − | − | − | − | − |
Positive standard (methylparaben tested at 0.4%) | / | / | / | +++ | +++ | +++ | +++ | +++ | +++ | +++ | +++ |
Ysaline – red algae extract (100 mg/ml) | / | / | EtOH/H2O 60/40 | − | − | − | − | − | − | − | − |
Totarol – Podocarpus totarol extract (100 mg/ml) | / | / | EtOH/H2O 60/40 | ++ | ++ | +++ | +++ | ++ | ++ | − | − |
As shown in Table 1, almost all of the tested extracts display good to excellent S. aureus growth inhibition activities. On the contrary, only the Pistacia lentiscus extract (EtOH/H2O 70:30 v/v) seems to present a good activity against the growth of P. aeruginosa after 48 h.
Interesting activities against the three remaining micro-organisms have been observed for the Laurus nobilis L. and Hypericum perforatum L. extracts (EtOH/H2O 75:25 v/v). The prominent antibacterial activity of the ethanolic extract of L. nobilis L. fresh leaves and twigs was already assessed [15]. Similarly, the effective antibacterial activity of the methanolic extract of L. nobilis L. seed oil was observed by Ozcan et al. [16] and one of the supercritical fluid extracts of L. nobilis L. leaves was observed by Santoyo et al. [17]. In a similar way, the antibacterial activity of various extracts of H. perforatum L. has already been assessed [18–20].
The EtOH/H2O 75:25 (v/v) crude extract of the flowered aerial parts of Santolina chamaecyparissus display the best unprecedented results against these micro-organisms (inhibition superior to 90% of the growth of C. albicans, A. niger and S. aureus), except for P. aeruginosa.
S. chamaecyparissus L., commonly known as cotton lavender, is a species of flowering plant belonging to the Asteraceae family. It is native to Southeastern (Croatia and Italy) and Southwestern Europe (France and Spain) and is mainly used as an ornamental plant [21].
This extract was analysed by using a High Performance Liquid Chromatography-Diode array Detector-Evaporative Light Scattering Detector (HPLC-DAD-ELSD; Fig. 1): besides polar (e.g., sugars and amino acids) and some hydrophobic compounds, this extract is mainly constituted by flavonoids and spiroketal derivatives, among which a major unknown compound eluting after 26 min is detected. This extract was then selected for further investigation and optimization for practical cosmetic formulation.
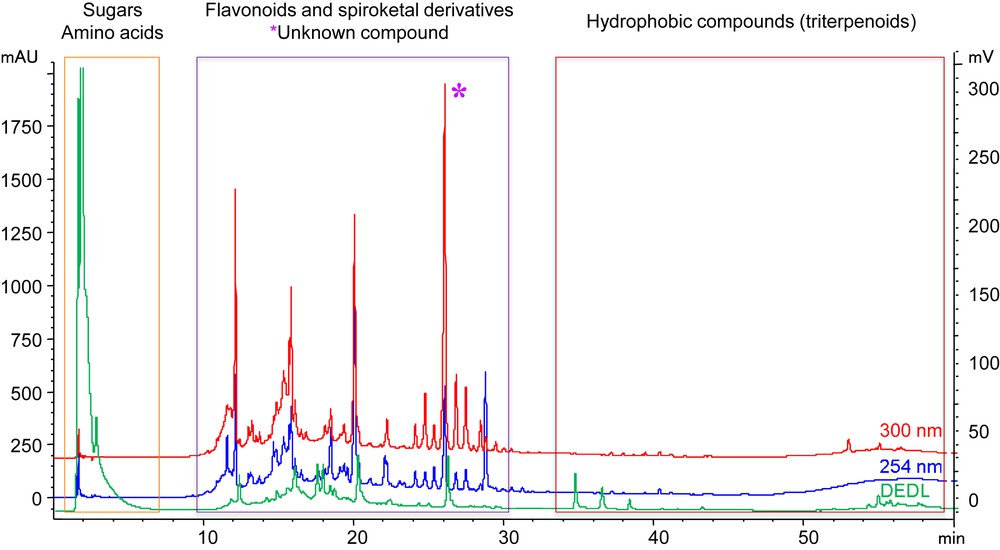
HPLC-DAD-ELSD profile of the crude extract of S. chamaecyparissus showing the major families of metabolites identified.
2.2 Bioguided fractionation of the S. chamaecyparissus extract
In order to identify the active compounds, the EtOH/H2O 75:25 (v/v) extract of S. chamaecyparissus was then fractionated over silica gel (normal phase). The fractionation of 2.2 g of the bulk extract led to the recovery of five distinct fractions: F1 (cyclohexane; 8 mg), F2 (cyclohexane/AcOEt 1/1; 249 mg), F3 (AcOEt; 88 mg), F4 (EtOAc/MeOH 1/1; 781 mg) and F5 (MeOH; 412 mg).
The resulting fractions were further evaluated for their antimicrobial activity. Fraction solutions were concentrated at 100 mg/mL in DMSO and their activities were assessed the same way as one of the crude extracts.
Table 2 shows the inhibition percentages of the five resulting fractions against four bacteria, yeast and fungi at a concentration of 0.4% (w/v) (the results obtained for the extract at a concentration of 0.04% are not shown in the present article). Methyl-paraben at 0.4% (w/v) was used as the positive control.
Antimicrobial activities of the S. chamaecyparissus crude extract and of the resulting F1–F5 fractions compared to those of a commercial preservative.
Sample | Strains | Growth inhibition at 0.4% | Average inhibition | ||
24 h | 48 h | 72 h | |||
Crude extract of S. chamaecyparissus | A. niger | 102 | 101 | +++ | |
C. albicans | 100 | 100 | +++ | ||
P. aeruginosa | 1 | − | − | ||
S. aureus | 97 | 98 | +++ | ||
F1 | A. niger | − | − | − | |
C. albicans | − | − | − | ||
P. aeruginosa | − | − | − | ||
S. aureus | − | − | − | ||
F2 | A. niger | 100 | 100 | +++ | |
C. albicans | 99 | 98 | +++ | ||
P. aeruginosa | 94 | 90 | +++ | ||
S. aureus | 105 | 113 | +++ | ||
F3 | A. niger | 90 | 103 | +++ | |
C. albicans | 96 | 44 | ∼ | ||
P. aeruginosa | 6 | − | − | ||
S. aureus | − | 15 | − | ||
F4 | A. niger | 68 | 7 | ∼ | |
C. albicans | 17 | 13 | − | ||
P. aeruginosa | 13 | 8 | − | ||
S. aureus | 36 | 56 | ∼ | ||
F5 | A. niger | 0 | 4 | − | |
C. albicans | − | − | − | ||
P. aeruginosa | − | 21 | ∼ | ||
S. aureus | 32 | 30 | ∼ | ||
Methylparaben | A. niger | 100 | 100 | +++ | |
C. albicans | 99 | 100 | +++ | ||
P. aeruginosa | 75 | 81 | ++ | ||
S. aureus | 80 | 93 | ++ |
No activity was observed for the cyclohexane (F1) and MeOH fractions (F5). The EtOAc/MeOH fraction (F4) was only active at 0.4% (w/v) against A. niger (inhibition percentage higher than 60%). The ethyl acetate fraction (F3) was significantly active at 0.4% (w/v) against A. niger and C. albicans, but no activity was observed against P. aeruginosa and S. aureus.
Finally, the cyclohexane/AcOEt fraction (F2) was the most interesting one, exhibiting a strong antimicrobial activity against the four evaluated strains over 48 h (C. albicans and S. aureus) and over 72 h (P. aeruginosa and A. niger).
2.3 Isolation and identification of the active metabolite
HPLC-DAD-ELSD analysis of both the crude extracts of S. chamaecyparissus and the five resulting fractions showed the presence of a major metabolite in F2 (Fig. 2) that is absent in other fractions: this major metabolite was suspected to be responsible for the antimicrobial activity of S. chamaecyparissus crude extracts or at least a contributor to it.
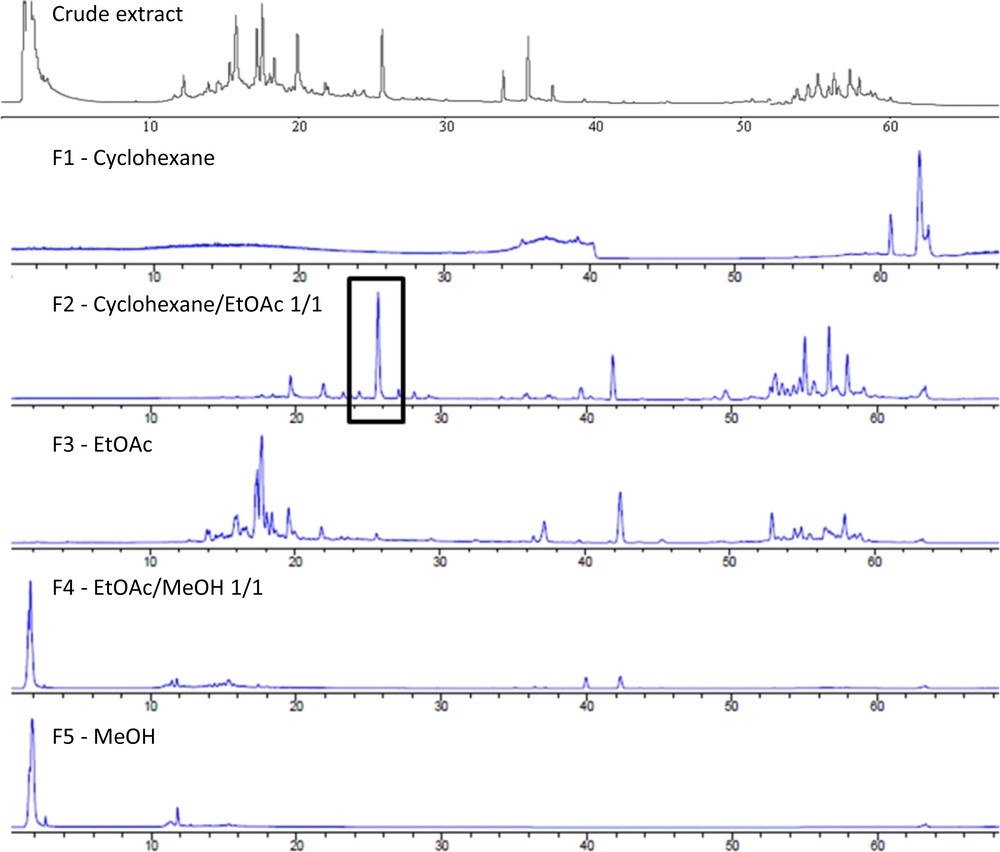
HPLC-ELSD profiles of the crude extract of S. chamaecyparissus and the resulting F1–F5 fractions.
Semi-preparative HPLC was then performed on this active fraction in order to confirm this assumption: multiple injections of this fraction concentrated at 50 mg/mL were carried out and 9 mg of the molecule were obtained.
Its molecular formula C15H12O4 was deduced from UPLC-ESI-HRMS experiments ([M+H]+ m/z 257.0816, C15H13O4). The UV spectrum showed the band of maximum absorption centred at 310 nm.
1H and 13C NMR, associated with COSY, HSQC and HMBC experiments revealed the presence of an acetoxy group, as suggested by the signal at δH 2.1 (3H,s, H-15), and at δC 20.5 (C-15) and 170.5 (C-14). Then δH and δC signals suggested the presence of a polyacetylene chain (C2C3C4C5) while the four quaternary carbons present a δC from 64.8 to 80.5; the interpretation is further confirmed using HMBC. Complete correlations are presented in Table 3. The structural analyses enabled the identification of (5S,7Z)-7-(hexa-2,4-diyn-1-ylidene)-1,6-dioxaspiro[4.4]nona-2,8-dien-4-yl acetate, from the polyacetylene family, already found in Santolina species (Fig. 3) [22–25]: commonly named spiroketalenol, it is referred to as SKE in the present article. No mention of any kind of the assessed antimicrobial activity was reported for this substance in the literature.
1H and 13C NMR chemical shifts and multiplicity.
C | δC (ppm) | C | δH (ppm) | Multiplicity |
1 | 4.8 | CH3 | 2.0 | s |
2 | 80.5 | Cq | – | – |
3 | 64.8 | Cq | – | – |
4 | 77.7 | Cq | – | – |
5 | 70.3 | Cq | – | – |
6 | 83.1 | CH | 5.1 | br s |
7 | 168.9 | Cq | – | – |
8 | 133.8 | CH | 6.3 | dd (J = 5.7 ; J = 1.6 Hz) |
9 | 126.1 | CH | 6.8 | d (J = 5.7 Hz) |
10 | 117.3 | Cq | – | – |
11 | 148.6 | CH | 6.6 | d (J = 3.0 Hz) |
12 | 100.8 | CH | 5.2 | dd (J = 2.5 Hz; J = 3.0 Hz) |
13 | 75.2 | CH | 5.7 | d (J = 2.5 Hz) |
14 | 170.5 | Cq | – | – |
15 | 20.5 | CH3 | 2.1 | s |

Spiroketalenol structure (thick bonds correspond to COSY correlations).
2.4 SKE antimicrobial activity
Pure SKE appeared to be unstable once isolated, particularly in aqueous and acidic conditions. Since antimicrobial assays are performed in water, its antimicrobial activity could not be directly evaluated as for crude plant extracts or fractions. Thus there was a need to develop a methodology to establish a relationship between the biological activity of crude extracts and their SKE content.
Such a quantitation of SKE was performed by HPLC-UV at 310 nm (SKE absorption maximum): a calibration curve was constructed using (E)-cinnamic acid as a standard and the active substance concentration was expressed as mg equivalent of (E)-cinnamic acid per gram of the crude extract.
Once the SKE concentrations in various extracts were determined, the corresponding antimicrobial activities were assessed. As shown in Table 4, a relationship between the SKE concentration and the antimicrobial activity, especially against A. niger and C. albicans can be evidenced: no activity against these two strains was detected when no SKE was quantified in the extract. On the contrary, as the SKE concentration increases, antimicrobial activity is observed and appears to be maximal when the SKE concentration is higher than 9.1 mg equiv/g of the extract.
SKE concentrations (mg equiv. cinnamic acid/g of extract) and antimicrobial activities of S. chamaecyparissus crude extracts as functions of harvesting place and period.
Crude extracts | SKE concentration (mg equiv/g of extract) | Antimicrobial activity | ||||
Harvesting place | Harvesting period | P. aeruginosa | S. aureus | A. niger | C. albicans | |
HP1 | 06/2010 | 7.9 | − | + | + | − |
HP1 | 02/2011 | 1.1 | − | + | + | + |
HP1 | 03/2011 | 0.4 | − | + | − | + |
HP1 | 06/2011 | 0.2 | − | + | − | − |
HP2 | 06/2011 | 1.4 | − | + | − | + |
HP2 | 02/2012 | 9.1 | − | + | + | + |
HP2 | 09/2012 | 17.6 | + | + | + | + |
HP2 | 03/2013 | 9.3 | − | + | + | + |
HP3 | 04/2011 | 0.3 | − | − | ∼ | − |
HP4 | 06/2011 | 0.0 | − | + | − | − |
HP4 | 07/2011 | 0.0 | − | + | − | − |
HP5 | 12/2012 | 5.8 | − | + | + | − |
However, the activity against S. aureus seems to be independent of the SKE presence: in fact, almost all the extracts containing SKE or not were active against this strain.
Finally, it was highly difficult to conclude about the activity against P. aeruginosa as only one extract containing SKE at 17.6 mg equiv/g was active against this last strain.
2.5 Stability assessment
The instability of isolated SKE has already been stated; it appeared crucial to test its stability in the S. chamaecyparissus extract incorporated into a cosmetic formulation before any further ingredient development.
Creams formulated with 0.5 (data not shown) and 2% of the S. chamaecyparissus extract together with various cosmetic supports were then subjected to stability tests: these cosmetic products were kept either at room temperature or at 42 °C for 13 weeks. The evolution of the SKE concentration in these finished products was followed by HPTLC, using an appropriate mobile phase to properly separate SKE from the other ingredients (retention factor Rf = 0.42).
A stability test was performed over 6 weeks at room temperature; then one week later (day 49), the formulated creams were either stored at room temperature or at 42 °C. As shown in Fig. 4, the SKE concentration remained stable at room temperature with the three cosmetic supports tested. However, SKE degradation was observed in a cream placed at 42 °C after 7 weeks at room temperature (only 40% of SKE was measured in the cream after 20 weeks): one can suggest that SKE, stable at room temperature, is heat sensitive, but further investigations should confirm this observation and appropriate storage conditions could be defined.
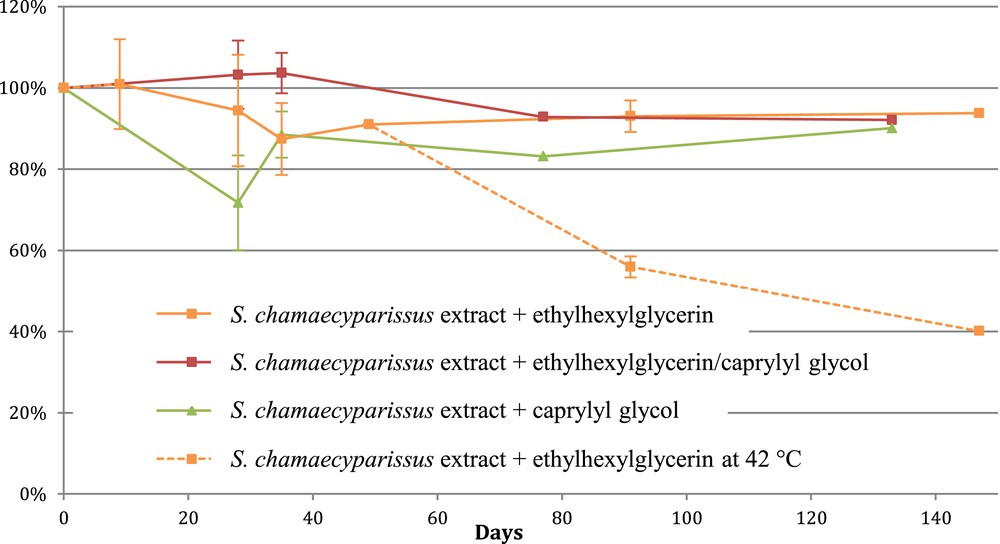
Evolution of the SKE content in creams formulated with 2% of the S. chamaecyparissus extract and subjected to stability tests.
Furthermore, it would be necessary to push these stability tests forward once the definite formulation has been determined: tests at room temperature and at 42 °C should be supplemented with tests in an ageing chamber where the effect of temperature variations mimicking the ones a finished product is subjected to, from formulation to consumer's use, could be monitored.
2.6 Natural ingredient design
Once the stability of the active molecule has been established in cosmetic formulae, the corresponding natural cosmetic ingredient can be developed.
The necessary requirements for such a development are quite numerous, as evidenced in Fig. 5: natural substances that show antimicrobial activity are usually not directly incorporable into a formulation as they may display undesirable qualities.
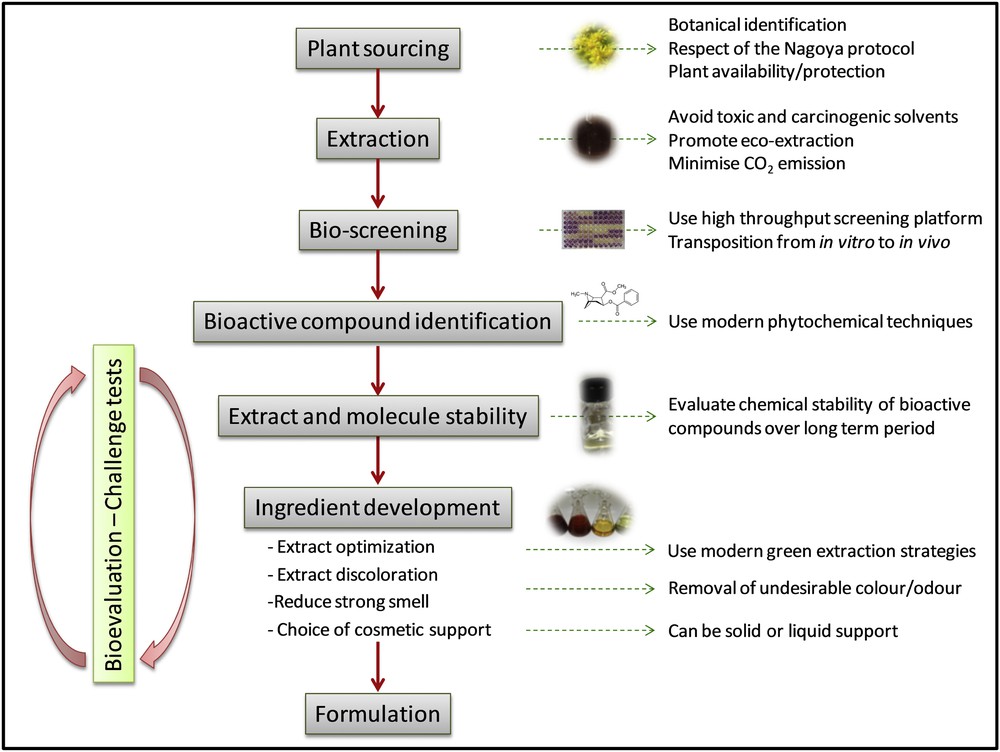
Strategy adopted to develop a natural cosmetic ingredient, from plant sourcing to formulation.
First, there is a necessity to respect the integrity of the active molecule during the extraction process, and hence to optimize this extraction process via the development of an appropriate non-toxic extraction solvent system or via the use of modern extraction techniques such as microwave assisted extraction, ultrasonic assisted extraction or supercritical fluid extraction. Secondly, some substances may display offensive odours and/or colours that would be unacceptable in skin care products [7] and therefore need to be subjected to further processing, including notably a discolouration procedure. Finally, the addition of a cosmetic support, either liquid or solid, may be necessary to facilitate its incorporation into a cosmetic formulation.
As the antimicrobial activities appeared to be correlated with extract's SKE concentration, optimization of the extraction process was in the first place performed via the modification of the solvent system used. Several extraction replicas were performed using each solvent system tested to testify individual repeatability: the extraction repeatability was good as the mean standard deviation per solvent system never exceeded 10%.
A comparison of EtOH/H2O gradients was initially performed, testing gradients ranging from 100% EtOH to 100% H2O. As shown in Table 5, the highest SKE concentration (19.9 mg equiv/g) was obtained using EtOH/H2O 80:20 (v/v). The more the water ratio in the extracting solvent system increased, the weaker the SKE concentration was. Similarly, using absolute EtOH rather than EtOH 96% leads to a decrease in the SKE concentration. An optimal ratio was thus established containing between 60 and 96% EtOH (v/v).
SKE concentrations (mg equiv cinnamic acid/g of extract) as a function of extraction solvent system.
Extraction solvent | SKE concentration (mg equiv/g) |
Absolute EtOH | 8.5 |
EtOH 96% | 15.0 |
EtOH/H2O 80:20 | 19.9 |
EtOH/H2O 60:40 | 2.9 |
EtOH/H2O 40:60 | 0.3 |
EtOH/H2O 20:80 | 0.0 |
H2O | 0.0 |
MeOH | 44.3 |
EtOAc | 20.3 |
THF | 12.0 |
Acetone | 31.0 |
DCM | 40.8 |
MethylTHF | 16.4 |
Other solvents were also compared to the extract of the plant. Methanol appears to be the extraction solvent giving the highest concentration of metabolite (44.3 mg equiv/g). Dichloromethane, acetone and ethyl acetate were also good candidates for the extraction of S. chamaecyparissus (40.8, 31.0 and 20.3 mg equiv/g, respectively). All these four solvents gave higher active concentration than ethanol/water 80:20. Methanol was subsequently selected for the following optimization of the extraction process to produce a cosmetic ingredient based on the S. chamaecyparissus extract [26]. However, caution should be taken as methanol as well as dichloromethane is CMR (carinogenic/mutagenic/reprotoxic) solvent, and its use in the extraction of the cosmetic ingredient is highly contraindicated and shall be progressively banned: any residual trace of extraction solvent in the finished product is scrutinized [27–29].
The antimicrobial activity of the methanolic extract was tested (see Table 6): a significant activity was observed against S. aureus, A. niger and C. albicans, whereas only a moderate activity was noted against P. aeruginosa and no activity at all was observed against Escherichia coli at 0.4%.
Antimicrobial activity of the MeOH extract of S. chamaecyparissus.
Crude extracts | Antimicrobial activity at 0.4% | ||||
P. aeruginosa | S. aureus | A. niger | C. albicans | E. coli | |
MeOH extract | ∼ | + | + | + | − |
As already stated, the presence of pigments (e.g., chlorophylls, anthocyanins, etc.) in solvent crude extracts dedicated to the development of cosmetic ingredients is unacceptable. Discolouration of such extracts can be performed using either activated carbon or molecular distillation. Both techniques were tested in the second phase of the optimization of a natural cosmetic ingredient based on the S. chamaecyparissus extract obtained using EtOH/H2O 80/20 (v/v).
Molecular distillation of cosmetic raw materials enables removing undesirable odours, colour and some of the impurities that are present in the starting materials. This distillation is performed in an extreme vacuum environment at high temperature for a very short contact time. This technique was applied on S. chamaecyparissus crude extracts using liquid medium-chained triglyceridic support: the resulting liquid product presents a translucent yellow to orange colour, quite interesting for cosmetic formulation. Unfortunately, once tested for anti-microbial activity, it appeared that the resulting extract do not display any interesting microbicidal activity. Furthermore, to perform such a distillation process, it is necessary to use an almost non-volatile solvent, traces of which, unacceptable in cosmetics, are quite difficult to remove thereafter.
Discolouration by adsorption of the undesirable molecule on activated carbon is quite easy as the powdered activated carbon can easily be added to the liquid extract, and then removed by settling and filtration. Discolouration of S. chamaecyparissus crude extracts was performed with 10% of active carbon. The active compound content was then dosed in discoloured extracts and in almost all cases, enrichment in SKE was observed as presented in Table 7.
SKE concentrations (mg equiv cinnamic acid/g of extract) in crude and discoloured extracts.
Crude extracts | SKE concentration (mg equiv/g) | ||
Harvesting place | Harvesting period | Crude extract | Discoloured extract |
HP1 | 04/2012 | 1.5 | 20.4 |
HP1 | 05/2012 | 29.4 | 26.7 |
HP1 | 06/2012 | 18.8 | 35.8 |
HP1 | 12/2012 | 24.0 | 51.2 |
HP1 | 01/2013 | 3.2 | 6.4 |
HP2 | 10/2012 | 5.3 | 44.0 |
The next step of this optimization procedure consisted in the addition of an adequate cosmetic support. Maltodextrine, a solid cosmetic support, was tested but it appears that after solvent evaporation, the S. chamaecyparissus extracts on maltodextrin were thick and doughy, inducing the necessity for a tedious solubilization step for subsequent incorporation into cosmetic formulations. Furthermore, this support only leads to the “dilution” of extract's antimicrobial activity, as maltodextrin itself do not display any interesting microbicidal potential.
Polyols and monoglyceride esters, liquid cosmetic supports, were then judiciously selected for the development of such a cosmetic preservative based on the S. chamaecyparissus extract, as they present potentially interesting antimicrobial activity, which could improve the antimicrobial activity of the final ingredient [7,30–32]. The solubility of the S. chamaecyparissus extract was hence evaluated in the following cosmetic supports: ethylhexylglycerin (S1), a mixture of ethylhexylglycerin and caprylyl glycol (S2), caprylyl glycol (S3), glyceryl caprate (S4), capryloyl glycine, glyceryl caprylate, glycerin, propylene glycol, butylene glycol and propanediol. The S. chamaecyparissus extract presented the highest solubilities in S1, S2, S3 and S4, and were only moderately soluble in the other raw materials tested. The first three were selected for further investigations and the resulting ingredients corresponding to the mixture of the crude extract of S. chamaecyparissus with either S1, S2 or S3 were subsequently named ingredient 1 to ingredient 3.
The antimicrobial activities of these three new ingredients were tested and compared to one of the crude extracts alone: results are presented in Table 8. Optimal activity (>90% of microbial inhibition) was observed for all ingredients against A. niger. The activity of ingredient 2 appears to be very good (>80% of inhibition) against E. coli and C. albicans and good (>60% of inhibition) against S. aureus, whereas it remained optimal with ingredients 1 and 3. Finally, ingredient 1 only displays a medium to poor activity against P. aeruginosa, whereas ingredient 2 presents a good one and ingredient 3, an optimal one against the latter strain.
Antimicrobial activities of the crude extract of S. chamaecyparissus and of ingredients 1, 2 and 3 (0.4% of extract).
Crude extracts | Antimicrobial activity at 0.4% | ||||
P. aeruginosa | S. aureus | A. niger | C. albicans | E. coli | |
Crude extract | ∼ | +++ | +++ | ∼ | − |
Ingredient 1 | ∼ | +++ | +++ | +++ | ++ |
Ingredient 2 | ++ | + | +++ | ++ | ++ |
Ingredient 3 | +++ | +++ | +++ | +++ | +++ |
So, all ingredients evaluated at this step of the study were highly active against the micro-organisms tested and were effectively more active than the crude extract alone: they were then selected for the next steps of the preservative development.
2.7 Cosmetic evaluation
The microbial safety of a cosmetic product constitutes a pre-requisite for its production and commercialization: the results of challenge tests are required to prove its microbiological stability [33].
To comply with this requirement, the three optimized ingredients were incorporated into various cosmetic formulations (O/W emulsions), one of which is described in Table 9. The features of the optimized cream were in accordance with those previously described by Kerdudo et al. 2015 [34].
Cream (O/W) formulation.
Phases | Ingredients commercial name | INCI name | Supplier | Quantity (%) |
A | / | Aqua | qsp 100 | |
Glycerin 99,5% | Glycerin | Interchimie | 4.00 | |
B | Carbopol EDT 2020 | Acrylates/C10-30 alkyl acrylate crosspolymer | Gattefosse | 0.15 |
C | Brij 72 | Polyoxyethylene(2) (and) stearyl ether | Croda France SAS | 2.00 |
Brij 721P | Steareth-21 (and) polyethoxylated alcohol | Quimica masso | 2.50 | |
Lanette 18 | Stearyl alcohol | Ami | 1.50 | |
Cutina GMS-V | Glyceryl stearate | Ami | 3.00 | |
Eutanol G | Octyldodecanol | Ami | 3.00 | |
Sophiderm | Squalane | Sophim | 2.50 | |
Arlamol HD | Isohexadecane | Quimica masso | 3.00 | |
Virgin jojoba oil | Simmondsia chinensis (Jojoba) seed oil | Olvea | 1.50 | |
Refined wheat germ oil | Triticum vulgare (wheat) germ oil | Olisud | 1.50 | |
Refined shea butter | Butyrospermum parkii butter | Interchimie | 2.00 | |
Silicone oil baysilone M350 | Dimethicone | Interchimie | 1.50 | |
Eusolex 9020 | Butyl methoxydibenzoyl-methane | IES | 1.00 | |
Uvinul MC 80 | Ethylhexyl methoxycinnamate | IES | 1.50 | |
Silicone oil SF1202 | Dimethicone | Brenntag specialties | 2.00 | |
D | TEA 99% | Triethanolamine | Brenntag Côte d'Azur | 0.11 |
E | – | Isononyl Isononanoate, Titanium Dioxide | – | 1.25 |
F | D panthenol 50P | Panthenol (and) propylene glycol | Laserson | 0.20 |
G | Vitamin E acetate | Tocopheryl | IES | 0.50 |
H | Sodium hyaluronate | 5.85 | ||
I | Crude extract of S. chamaecyparissus | – | – | 0.00–2.00 |
Cosmetics containing antimicrobial extracts at concentrations ranging from 0.5 to 2.0% were exposed, as recommended by the FDA, to challenge tests to ensure the safety (e.g., the ability of the preservative system to protect the product from microbial growth) of both the ingredients alone and the finished cosmetic products prior to marketing and consumer use. As expected, the control cream, e.g., without preservative of any sort, was not in accordance with the European Pharmacopoeia standards [35]. The three assays containing 2% of the respective ingredient produced interesting results. The cream containing ingredient 3 appears to be resistant enough to conform to criteria A of the European Pharmacopoeia against all microorganisms [26,35]. Furthermore, the cream containing ingredient 2 appears to be in compliance with criteria A of the European Pharmacopoeia against P. aeruginosa, S. aureus, E. coli and C. albicans and with the criteria B of the European Pharmacopoeia against Aspergillus brasiliensis. Finally, the finished product containing ingredient 1 was also of interest as it was active against all microorganisms (criteria A of the European Pharmacopoeia) except against A. brasiliensis.
These assays were stopped after 14 days of challenge tests.
3 Materials and methods
3.1 Materials
Water (Chromasolv® for HPLC, purity > 99.8%), acetonitrile (Chromasolv® for HPLC, purity > 99.9%), 2-propanol (Chromasolv® for HPLC, purity > 99.9%), formic acid (Puriss, purity > 98%), (E)-cinnamic acid, ethanol, methanol, acetone and dichloromethane were purchased from Sigma–Aldrich (Saint-Quentin Fallavier, France). Deuterated chloroform (CDCl3) for NMR experiments was purchased from Euriso-top (Saint-Aubin, France). Glycerin (99.5%), butylene glycol and propylene glycol were purchased from Interchimie (Bobigny, France). Sensiva SC50 (ethylhexylglycerin stabilized with vitamin E) and Sensiva SC10 (caprylyl glycol and ethylhexylglycerin stabilized with vitamin E) were obtained from Schülke (Gentilly France). Microcare Emollient CLG (caprylyl glycol) was distributed by Coptis (Croissy-sur-Seine, France). Dermosoft GMC (glyceryl caprate) and Dermosoft GMCY (glyceryl caprylate) were purchased from Dr. Straetmans (Hamburg, Germany). Zemea (propanediol) was obtained from Dupont Solvents.
3.2 Plant material
All the plants initially selected were collected in 2010 in the Provence-Alpes-Côte d’Azur region, in the Alpes-Maritimes, Var and Vaucluse departments.
Aerial parts of S. chamaecyparissus L. were collected from Cabris, Isola, Mouans-Sartoux in the Alpes-Maritimes department, and from Mont Caume and Mont Coudon in the Var department.
The plant material was botanically authenticated by J.-L. Polidori and F. Boillot, both botanists, and a specimen of S. chamaecyparissus L. collected from Mont Caume, Var, in April 2013 has been deposited at the Regional Botanical Conservatory of Nice (voucher n° C 3746).
3.3 Plant extraction
Aerial parts of S. chamaecyparissus L. were harvested, dried, ground into powder and extracted under mechanical stirring using organic solvents. Two consecutive 2 h-extractions were performed at 25 °C and the resulting extracts were filtered, gathered together and vacuum-concentrated.
When cosmetic support was added, solvent extraction was performed as previously: the raw cosmetic material was added before solvent extraction in proportion 1:1 (w/w).
3.4 Sample preparation
Crude extracts and fractions were diluted at 20 mg/mL in methanol (MeOH) and filtrated over 0.45 μm PTFE syringe filter before HPLC analysis.
For HPTLC analyses, crude extracts and creams were dissolved in MeOH at 2 and 20 mg/mL respectively, ultrasonicated for 15 min and centrifuged: 2 μL of each supernatant were systematically applied on the HPTLC plate for analysis.
3.5 HPLC
Crude extracts and fractions were analysed using an HPLC Agilent 1200 system (Courtaboeuf, France) equipped with a DAD and an ELSD operating under the following conditions: injection volume: 20 μL, flow rate: 1.0 mL/min, UV-detection performed at 310 nm. The mobile phase consisted in a gradient of water (A), acetonitrile (B) and 2-propanol (C), all acidified with 0.1% acid formic: 0–5 min, 5% B; 5–35 min, 5–100% B; 35–45 min, 100% B; 45–50 min, 100–40% B and 0–60% C, 50–60 min, 40% B and 60% C, 60–65 min, 40–5% B and 0–95% A, 65–70 min, 5% B and 95% A.
Quantification of the active molecule was performed by HPLC-UV at 310 nm (wavelength corresponding to the absorption maximum of the active molecule) under the same analytical conditions and with the same solvent gradient (but stopped after 43 min) as the one described previously. A calibration curve was constructed using (E)-cinnamic acid dissolved in methanol at concentrations ranging from 0.0078 to 1 mg/mL as a standard. SKE concentrations, expressed as mg equivalent of (E)-cinnamic acid per gram of the crude extract, were determined thanks to the linear regression equation generated by the calibration curve after integration and area measurement of the corresponding peak in various extracts.
Semi-preparative HPLC of the cyclohexane/ethyl acetate fraction resulting from the column fractionation of the S. chamaecyparissus extract (see Compound isolation) was performed on a Luna C18 column (Phenomenex, 250 × 10 mm; 5 μm). Elution was performed using a gradient of non-acidified water (A) and acetonitrile (B): 0–5 min, 60% B; 5–35 min, 75% B and 35–45 min, 100% B.
3.6 HPTLC
High performance thin-layer chromatography (HPTLC) was performed on 10 cm × 20 cm HPTLC silica (0.20 mm) gel 60F254 pre-coated glass plates (Merck). Standards and samples were applied as 8 mm large bands (15 tracks per plates, 50 nL/s delivery speed), 20 mm from the both plate edges by means of an automated ATS4 sampler (Camag). Two microlitres of each standard and samples were applied on silica gel plate. The mobile phase consisted of a solution of toluene/ethyl acetate/formic acid 98:2:1 (10 ml). The separation on the silica gel plate was performed in an automatic ADC2 developing chamber (Camag) with the tank previously saturated for 20 min with 25 ml of the developing solvent (humidity previously controlled for 10 min: 33–38%). Developing distance was 70 mm from the lower edge of the plate. Documentation of chromatographic plates (visualization and image capture) was performed at 254 nm, 366 nm and under white light by the TLC Visualizer documentation (Camag) operated with winCATS Version 1.4.6 software. Plates were scanned under the following conditions using the TLC scanner 4 controlled by the WinCATS software: scanning mode, reflectance mode at 315 nm, D2 (deuterium) and W (tungsten) lamps, slit dimension 8.00 mm × 0.40 mm, scanning speed 20 mm/s, and data resolution 100 μm/step.
SKE presents a good UV absorbance, reaching a maximum at λ at 315 nm, without any co-elution.
The quantification of SKE content in formulated creams was performed via calibration curves constructed with five relative concentrations of ingredients 1, 2 and 3 diluted in MeOH and applied in triplicate on the HPTLC plate (stock solution prepared at 1 mg/ml; application of respectively, 1, 2, 6, 8 and 12 μL of the corresponding solutions on the HPTLC plate).
The R2 coefficients were all acceptable above 0.999.
3.7 Compound isolation
Open column chromatographic fractionation of the crude extract was performed using silica gel (normal phase) and yielded five fractions: cyclohexane fraction (F1), cyclohexane/ethyl acetate 1:1 v/v fraction (F2), ethyl acetate fraction (F3), ethyl acetate/methanol 1:1 v/v fraction (F4) and methanol fraction (F5).
3.8 1H and 13C NMR analysis
NMR analyses were carried out on Bruker Avance DRX500 (500 MHz) (Brucker, Wissembourg, France). Mono- (1H and 13C) and bi-dimensional (COSY, HSQC, HMBC, NOESY) NMR spectra were recorded in CDCl3 at 25 °C (Euriso-top, Saint-Aubin, France); chemical shifts (δ) are given in parts per million (ppm), and coupling constants (J) are given in hertz (Hz).
3.9 UPLC-ESI-HRMS
UPLC-ESI-HRMS analyses were performed on a QStar Elite QTOF mass spectrometer (Applied Biosystems SCIEX) operating under atmospheric pressure ionization, in positive-ion mode. Samples diluted in methanol at a concentration of 1 mg/mL, were eluted on an Acquity UPLC BEH C18 column (Waters, 100 × 2.1 mm, 1.7 μm, 35 °C); the flow rate was set at 0.6 mL/min and the injection volume was 1 μL.
The mobile phase consisted of water (A) and acetonitrile (B), both acidified with 0.1% formic acid: 0–5 min, 0% B; 5–10 min, 0–100% B; 10–15 min, 100% B.
Compound 1: Powder, UV λmax (MeOH) 310 nm, C16H12O4, MW = 256.2534, HRMS m/z 257.0816 (calcd for C16H13O4, 257.0816).
1H NMR (500 MHz, CDCl3) δH 6.8 (d, J = 5.7 Hz, 1H), 6.6 (d, J = 3.0 Hz, 1H), 6.3 (dd, J = 5.7, 1.6 Hz, 1H), 5.7 (d, J = 2.5 Hz, 1H), 5.2, (dd, J = 2.5, 3.0 Hz, 1H), 5.1 (br s, 1H), 2.1 (s, 3H) and 2.0 (s, 3H) ppm.
13C NMR (125 MHz, CDCl3) δC 170.5, 168.9, 148.6, 133.8, 126.1, 117.3, 100.8, 83.1, 80.5, 77.7, 75.2, 70.3, 64.8, 20.5 and 4.8 ppm.
3.10 Antimicrobial activity
The crude extracts (tested at 2% and 0.2%), the subsequent fractions (tested at 0.4% and 0.04%) and the ingredients (tested at 0.4%) were evaluated for their antimicrobial activity against the fungus A. niger ATCC 16404, the yeast C. albicans ATCC 10231, the gram(−) bacteria P. aeruginosa ATCC 9027 and S. aureus ATCC and the gram(+) bacteria E. coli ATCC 8739 (only the ingredients were tested over E. coli). Bacterial and yeast trains were respectively grown in liquid nutrient broth (Difco, Surrey, England) at 37 °C, for 24 and 48 h before antimicrobial testing.
The assays were performed in 96-well plates as follows: extract samples were diluted in sterile water. Each concentration was assessed in triplicate with one supplementary control well containing no microbial strain. Negative control was constituted of the solvent mixture used to prepare the sample solutions, and positive control consisted of methyl-paraben, a synthetic preservative, at 0.4%. The 96-well plates were incubated at 37 °C, and the optical density (OD) reading was obtained at 620 nm, 24, 48 and 72 h after the beginning of the incubation to evaluate inhibition of microorganism growth. The results were expressed as a percentage of inhibition according to the formula:
3.11 Cosmetic formulation
All the formulations were prepared on a 100 g final mass-basis. Stirring was performed using a propeller mixer (Turbotest laboratory mixer equipped with an axial flow propeller, Rayneri, VMI). The cream formulations were prepared as described by Kerdudo et al. 2015 [34], using the ingredients listed in Table 9.
3.12 Challenge test
Antimicrobial preservative efficiency evaluation was performed by an external laboratory (Ideatest, Plouzané, France) following the procedure described in the European Pharmacopoeia [35].
Five potential pathogenic germs were chosen for this study (a cosmetic contamination by these microorganisms could notably cause skin infection or respiratory diseases): Pseudomonas aeruginosa ATCC 9027, S. aureus ATCC 6538, E. coli ATCC 8739, C. albicans ATCC 10231 and A. brasiliensis ATCC 16404 [36]. Tryptic soy agar and Sabouraud dextrose were respectively used as culture media for bacteria and for yeast/fungus. Cosmetic formulations were sampled in single-use sterile flasks and every flask was inoculated with strain suspension (at 22.5 ± 2.5 °C in the dark). The inoculation concentration was fixed at 106 CFU/mL, 105 CFU/mL and 104 CFU/mL respectively, for bacteria, yeast, and fungus. The validation of the neutralization of the preservative was realized on the five strains, in the 1/10th and 1/100th dilution, with LT100 broth. The microbial densities were counted by inclusion (results in CFU/g or mL), and compared with the logarithmic reduction criteria of the European Pharmacopoeia presented in Table 10.
European Pharmacopoeia criteria (logarithmic reduction as a function of days D).
European Pharmacopeia | |||||
Strains | Criteria | Logarithmic reduction | |||
D2 | D7 | D14 | D28 | ||
Bacteria | A | 2 | 3 | / | NIa |
B | / | / | 3 | NI | |
Yeast and molds | A | / | / | 2 | NI |
B | / | / | 1 | NI |
a NI: No Increase, /: no minimal reduction required.
4 Conclusion
The present paper presents the development of a natural preservative for cosmetics, from plant sourcing to the final product formulation and challenge test, via the isolation of the bioactive molecule. When it comes to the development of new active ingredients for cosmetics, biodiversity is a rich source for inspiration and innovation, but as already discussed, such a development is a long-term and often unrewarding procedure. In fact, only five in every 100 genetic resources identified as being potentially of interest will ever end up in a cosmetic formula once their efficacy, quality and safety have been established [10].
After antimicrobial activity screening of 17 Mediterranean plants, S. chamaecyparissus was selected and further bioguided fractionation led to the isolation and identification of a major bioactive metabolite, spiroketalenol (SKE).
Quantitation and antimicrobial assays helped to highlight the correlation existing between extracts' SKE content and their corresponding activity against microorganisms.
The stability of SKE in cosmetic formulation was successfully assessed at room temperature, so further optimization of the natural ingredient took place. Extraction assays enabled the selection of methanol as the best solvent to enrich the S. chamaecyparissus extract in SKE. Discolouration of the extract performed by adsorption of the molecules responsible for the colouration on activated carbon, led to further SKE enrichment of the S. chamaecyparissus extract.
Various cosmetic liquid supports adapted to the cosmetic formulation and those displaying intrinsic antimicrobial activity were successfully added during the extraction process and highly active ingredients were obtained.
Formulation assays were performed using the different ingredients developed and subjected to challenge tests: the cosmetic cream formulated with so-called ingredient 3 consisting of caprylyl glycol and the S. chamaecyparissus extract (1:1) conforms to criteria A of the European Pharmacopoeia and so, appears to be a good candidate for future cosmetic use as a natural preservative.
Acknowledgements
The authors are grateful to C. Monin (ACPHYTAROMA) who collected and supplied the plants included in the screening presented in this paper. They also wish to thank the botanists J.-L. Polidori and F. Boillot (Biophyto) for the identification of these plants.
This work was a part of the APRF NATUBAVAL project (Pole PASS), which was made possible thanks to the financial support provided by the FEDER project number 2009_26268/2010_07953 (Provence-Alpes Côtes d'Azur region and European Union) and the ANRT (French National Association for Research and Technology) grant No 748/2010.