1 Introduction
The selectivity and activity of homogeneous catalysts under mild reaction conditions are unbeaten by their heterogeneous counterparts. Nevertheless, only a few industrial processes use homogeneous catalysts due to their cost, together with the difficulties of final separation of the metal species and residues [1]. To overcome these drawbacks, a range of approaches has been developed to increase the productivity of homogeneous catalysts, the most preferred of which, is based on their subsequent recycling [2]. Organic Solvent Nanofiltration (OSN) is an emerging technology and a green approach for the separation and recovery of a homogeneous catalyst or organometallic residues because the separation is performed at room temperature without any phase change, thus favouring the complex stability [3]. The retention by OSN is due to complex and not fully understood mechanisms involving not only size exclusion but also physico-chemical interactions between the membrane and the solute to retain or transmit through the more or less dense polymeric membrane. Nevertheless, the size difference between the catalyst and the reactants/products remains a parameter to consider for the implementation of the filtration process considering that often the reactants/products are close in size and consequently their separation is more difficult because it is based on subtler mechanisms than only size exclusion. To take into account this parameter, the design of steric size enlarged catalysts has often been a method of choice [4]. Interesting examples of steric size enlarged catalysts in combination with nanofiltration appeared in the literature covering a variety of homogeneously catalysed reactions such as hydroformylation, olefin metathesis, and cross-coupling reactions [5].
Olefin metathesis reactions represent a powerful catalytic tool for the formation of carbon–carbon double bonds allowing the preparation of various chemical intermediates useful for fine chemistry, pharmaceuticals and polymer synthesis [6]. Recently, successful progress in olefin metathesis stems from the transformation of unsaturated renewable resources such as fats and oils [7], and other types of bio-sourced compounds [8]. Nevertheless, the cost of homogeneous catalysts is an impediment for the development of industrial processes [9]. Owing to their stability, activity and potential recyclability, Hoveyda pre-catalysts have received a great attention as homogeneous catalysts [10]. However, nearly 20 years after the first report on Hoveyda's catalyst, the recyclability of such catalysts based on the “boomerang” or “release and return mechanism” is still controversial [11]. OSN was first used in an olefin metathesis reaction by Van Koten et al. [12], who reported the performance of a dendrimer immobilized catalyst in a model Ring Closing Metathesis (RCM) reaction of diethyl diallylmalonate (DEDAM). Poor conversion was observed likely due to the deactivation of the catalyst by the MPS-60 (PolyDiMethylSiloxane (PDMS), Koch®, no more commercially available) nanofiltration membrane. Since this first report, several groups including ours have reported on the implementation of OSN in olefin metathesis transformations with the aim of improving catalyst productivity. Keraani et al. [13] prepared a variety of tailor-made pre-catalysts of different molecular weights, ranging from 625 to 2195 g mol−1, in which the Molecular Weight Enlargement (MWE) group was attached to the benzylidene ligand of the Hoveyda catalyst. Plenio et al. [14] reported the synthesis of enlarged Hoveyda pre-catalysts (1080 g mol−1), in which the MWE unit was attached to the NHC ligand of an unmodified Hoveyda pre-catalyst. The nanofiltration in a batch mode of the catalyst using a Starmem 228 membrane (Polyimide membrane, molecular weight cut-off [15] of 280 g mol−1, MET-Evonik) showed a recovery of more than 99%. Recently, Grela et al. [16] reported the synthesis of enlarged Hoveyda pre-catalysts, in which a PolyOctahedral SilSesquioxane (POSS) ligand was attached to the NHC of the unmodified Hoveyda pre-catalyst. Application of this catalyst in a continuously flow operated membrane reactor showed good performances in RCM reactions. More recently, Fischmeister et al. [17] developed a series of four new enlarged Hoveyda pre-catalysts, in which the MWE unit was attached to the NHC ligand of the Hoveyda pre-catalyst. These enlarged catalysts showed good activities in the model RCM reaction and high retention by OSN (99%) [18].
Herein we report our efforts to develop straightforward synthetic methodologies for the preparation of a series of tailor-made enlarged Hoveyda pre-catalysts designed for efficient separation by OSN considering that structural modifications of the ligands should not hamper the catalyst properties. The characterizations and catalytic activity of new enlarged Hoveyda pre-catalysts are reported.
2 Results and discussion
The modification of Hoveyda pre-catalyst I (Fig. 1) aiming at facilitating separation by OSN was envisioned in such a manner that these modifications should have a very negligible effect on the catalytic efficiency of the sterically enlarged complexes. Previous studies from different groups showed the influence on the catalyst efficiency of the aryl substituents of the chelating o-isopropoxystyrene ligand, in particular the initiation step, and the stability. For instance, Blechert et al. showed that steric hindrance at the o-substitution resulted in high initiation rates [19]. Similarly, Grela et al. demonstrated that p-substitution by an electron-withdrawing group also resulted in fast initiation of the pre-catalyst [20].
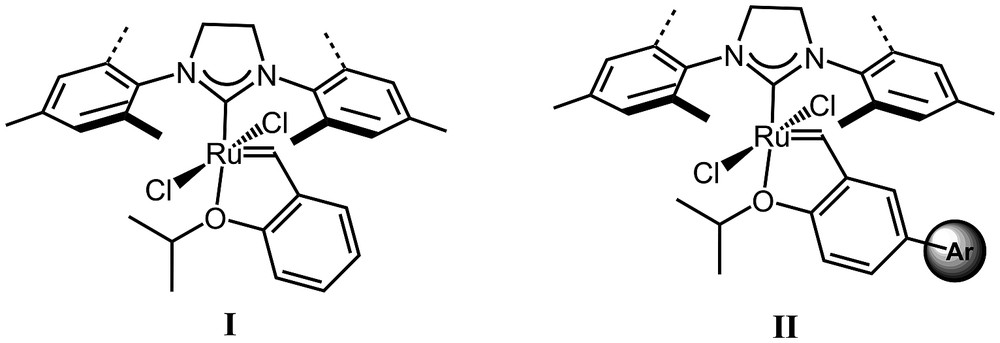
Commercial Hoveyda pre-catalyst (I) and enlarged pre-catalyst (II).
The design of tailor-made enlarged Hoveyda-type pre-catalysts for efficient separation by nanofiltration was envisaged by the enlargement of the o-isopropoxybenzylidene ligand with bulky and rigid p-aromatic substituents (Fig. 1, II). Such modification of the ligand was expected to bring only molecular weight enlargement (MWE) with little modification of the electronic and steric direct influence on the metal centre. The synthesis of the styrene derivative 5 was first performed. To ensure an efficient steric enlargement, we selected an o-methyl substituted phenyl substituent in order to decrease π-conjugation between the two phenyl rings hence preventing the planarity of these two benzene rings. The boronic ester 2 was generated in good yield by treatment of commercially available 2-bromotoluene 1 with B(OMe)3. A palladium-catalyzed Suzuki cross-coupling with 3 [21] yielded the aldehyde 4 in good yield. The final step involved a Wittig olefination that provided the desired styrenyl derivative 5 in 87% yield (Scheme 1).
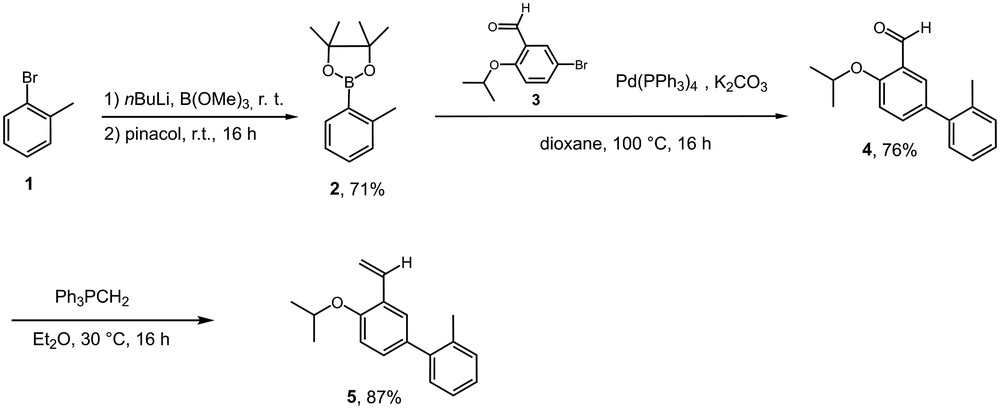
Synthesis of ligand 5.
The corresponding pre-catalyst IIa was then synthesized by exchange of a benzylidene ligand in Grubbs second generation pre-catalyst GII assisted by CuCl as a phosphine scavenger. IIa was thus isolated in 75% yield (Scheme 2) [10b].

Synthesis of catalyst IIa.
In order to further increase the steric bulk while preserving a rigid biphenyl moiety, a second styrenyl ligand was synthesized from the commercially available 4-bromo-2,6-di-tert-butylphenol 6. Etherification of 6 was first performed to deliver 7 in modest yield due to steric congestion imposed by the two tert-butyl fragments. The sequence optimized for the synthesis of 5 was then implemented to yield ligand 10 in three steps with good yields. Pre-catalyst IIb was then synthesized and isolated in 80% yield (Scheme 3).

Synthesis of pre-catalyst IIb.
Polymetallic structures present interesting characteristics with regards to their molecular size and bulkiness. For instance, a few examples of dendrimer-supported pre-catalysts have been reported [10b,12,22]. We envisioned that a rigid core made of phenyl rings decorated at the periphery with ruthenium pre-catalyst units would be an efficient way to ensure high retention by OSN. For this purpose, the synthesis of compound 15 was performed in four steps starting from the commercially available p-bromoacetophenone 11. A first condensation delivered the tris-bromo rigid core 12. This compound was further transformed using the sequence as reported for 5 and 10 (Scheme 4).

Synthesis the poly-phenyl core ligand 15.
The corresponding trimetallic pre-catalyst was prepared under the same experimental conditions as for IIa and IIb (Scheme 5).
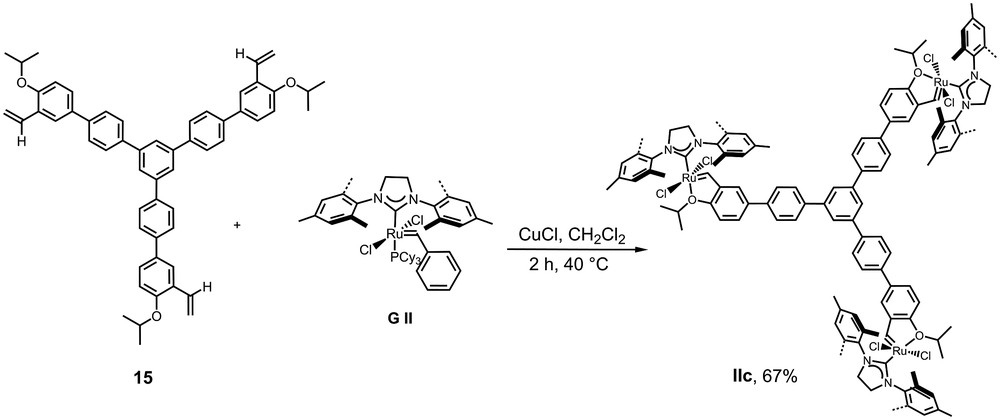
Synthesis of catalyst IIc.
The activity of complexes IIa, b, c was evaluated with the model ring closing metathesis (RCM) reaction of DiEthyl DiAllylMalonate (DEDAM) using a well-defined procedure (Scheme 6) [23].

Model RCM of DEDAM.
As summarized in Table 1, pre-catalyst IIa was as efficient as the Hoveyda catalyst I, whereas complexes IIb and IIc reached lower conversion after 30 min. However, higher conversions were obtained upon extended reaction time suggesting slower activations of these pre-catalysts. This observation for IIb is consistent with Blechert's study on the electronic effect of various substituents on the overall efficiency of ruthenium catalysts [24]. Indeed, electron-donating substituents introduced on the aryl ring of the styrenyl ligand resulted in slower activation of the pre-catalyst. The slower activation of IIc may result from conjugation along the aromatic core. Such issues have been observed by us [25] and others [26] in the case of naphthyl-based chelating ligands.
Ring closing metathesis of DEDAM with IIa, b, and ca.
Pre-catalyst | Time (min) | Conversion (%)c |
I | 30 | 95 |
IIa | 30 | 92 |
IIb | 30 | 84 |
IIcb | 30 | 74 |
a Catalyst 1 mol %, CH2Cl2, [DEDAM] = 0.1 M 30 °C, 30 min.
b 0.33 mol %.
c Determined by gas chromatography using dodecane as the internal standard.
These complexes were evaluated by OSN using a Starmem 228 membrane (cross-flow 0.80 mL min−1, transmembrane pressure 25 bar) where it was demonstrated that the steric bulk and structure rigidity brought by aryl substituents in particular in IIc was not as important as anticipated for the efficient recovery of the complexes [27]. As depicted in Fig. 2, the convection flow due to the TransMembrane Pressure (TMP) results in an increase of the solute concentration near the membrane with the formation of a concentration polarisation layer. We believe that the expected high retention of the molecular weight enlarged complex IIc was partly compensated by a decrease of its diffusion coefficient before the membrane entrance thus limiting its retro-diffusion (Fig. 2, (3)) from the concentration polarisation layer. Consequently, the accumulation of the complex at the membrane wall (Fig. 2, (4)) resulted in favourable transfer through the membrane (Fig. 2, (5)). Of course an in-depth study of the transfer mechanism of all these complexes might be achieved to definitively confirm this assumption but this is out of the scope of this article.

Solute concentration as a function of the distance from the membrane wall.
For this reason, we have prepared new pre-catalysts incorporating structural modification of the ligand by aliphatic groups. For instance, ligand 19 was easily obtained in three steps and the corresponding pre-catalyst IId was obtained in 88% yield (Scheme 7).
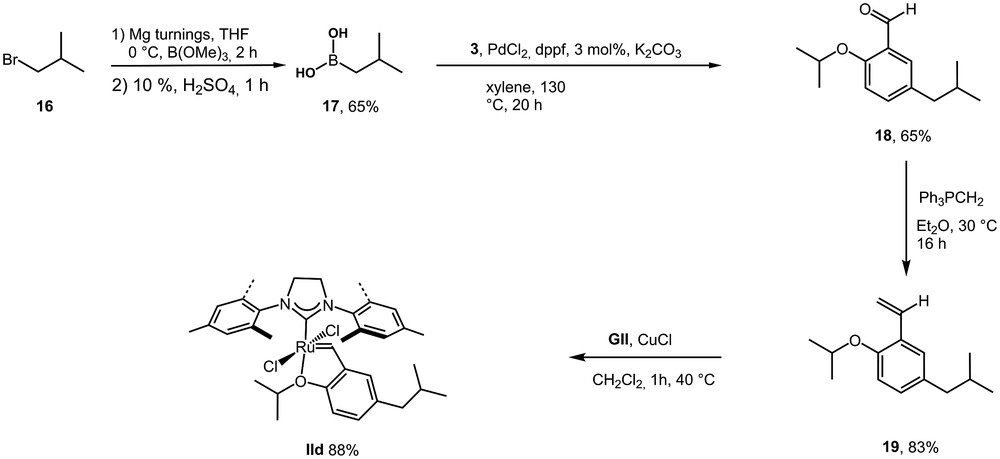
Synthesis of an iso-butyl substituted ligand 19 and the corresponding pre-catalyst IId.
A second new complex incorporating a siloxy-substituent was prepared as a model product of a family of tunable compounds. Indeed, a broad scope of chlorosilanes is commercially available from which a large number of ligands are potentially accessible. Furthermore, the same strategy employing dichlorosilanes would also be useful for catalyst heterogenization through the grafting of such ligands via siloxane bonds on the surface of inorganic oxides. Ligand 24 was easily prepared employing previously optimized synthetic methods. The corresponding pre-catalyst IIe was then prepared in very good yield (Scheme 8).
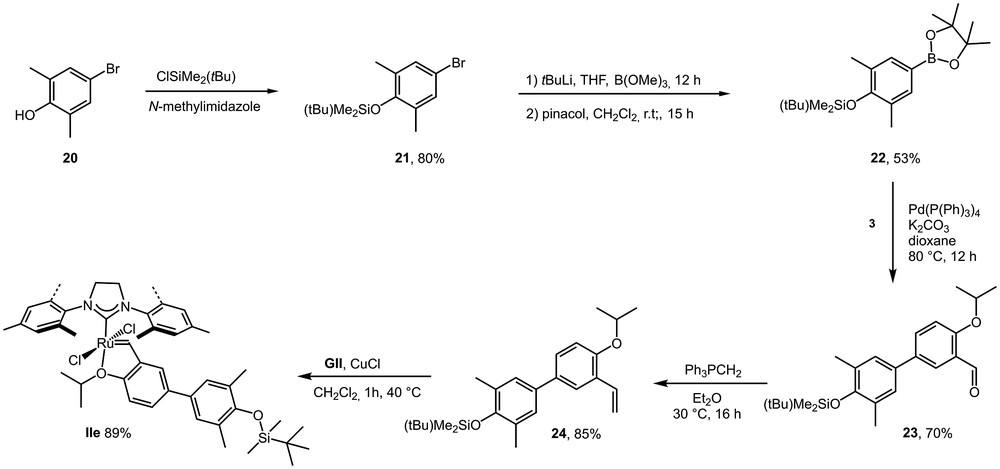
Synthesis of siloxy-substituted ligand 24 and corresponding pre-catalyst IIe.
As for the first series of complexes, these two new complexes were evaluated in the RCM of the same substrate DEDAM using slightly different experimental conditions. As depicted in Table 2, both catalysts exhibited performance as high as the Hoveyda pre-catalyst I hence demonstrating that the structural modification brought by the introduction of these aliphatic substituents did not alter the catalyst efficiency measured after 30 min at 25 °C.
Ring Closing metathesis of DEDAM with IId and ea.
Pre-catalyst | Time (min) | Conversion (%)b |
I | 30 | 85 |
IId | 30 | 86 |
IIe | 30 | 86 |
a Catalyst 1 mol %, [DEDAM] = 0.1 M, CH2Cl2, 25 °C, 30 min.
b Determined by gas chromatography using dodecane as internal standard.
The OSN of pre-catalyst IId was studied using a different NF setup than that used for IIa–c. A low molecular weight cut-off (220 g mol−1) Starmem® 122 membrane (Polyimide membrane, MET-Evonik) and a continuous cross-flow filtration unit ensuring reduced membrane fouling were used (Fig. 3).

Dead-end vs cross-flow filtration.
Under optimized conditions (transmembrane pressure TMP = 40 bar and cross-flow velocity v = 0.1 m s−1), the retention of commercially available Hoveyda pre-catalysts at 0.5 mmol L−1 reached 99.5%. Under the same conditions (optimized for Hoveyda pre-catalysts but not for prototypes that can exhibit a difference in the transfer mechanism through the membrane), the retention of IIe reached a similar and very high retention of 98.5% (Table 3). Further studies with OSN membranes with a higher MWCO or made of other polymers should demonstrate the full potential of this structural modification.
3 Conclusion
In conclusion, we have set up a synthetic protocol for the gradual increase of the steric hindrance of olefin metathesis pre-catalysts. A series of five enlarged homogeneous Hoveyda pre-catalysts of different molecular weights, ranging from 682 to 2195 g mol−1, was successfully synthesized and characterized. The structural modifications have been introduced in the benzylidene ligand in order to introduce steric size enlargement in view of further utilization in nanofiltration processes. The five enlarged Hoveyda pre-catalysts are very stable and the activity of three of these catalysts in the RCM of diethyl diallylmalonate is not altered by the structural modifications. Their retention in an OSN process is very good and offers interesting potential for further development of this environmentally friendly separation process. Further fine studies on the solute/membrane interactions will be necessary to address the full potential of the OSN process as the results obtained under one single set of conditions are not sufficient.
Acknowledgements
The authors acknowledge the Ministère de la Recherche for a PhD grant to A.K., and the Agence Nationale de la Recherche (ANR) (ANR-09-CP2D-11-01, NanoremCat2) for financial support and PhD funding to S. S. and G. N.