1 Introduction
The micellization of AB or ABA block copolymers in selective solvents, either of the A or of the B block, has been studied extensively in recent years and has become a topic of considerable academic and industrial interest. In fact, the self-aggregation aspects of block copolymers are involved in their widespread application possibilities such as polymeric surfactants for the stabilization of various types of emulsions, pigment dispersants, nanoparticles (NPs) for controlled drug delivery, and so forth. The formation of block copolymer micelles is now a well-established fact and the different theoretical aspects of such polymeric colloidal systems are described in several review articles [1–6].
Many studies have been carried out until now aiming to investigate the theoretical predictions on diblock micellar systems as a function of molecular characteristics such as composition and polymerization degree (DPn) of the blocks [7–10]. Another parameter that can strongly influence the micellization of copolymers in a selective solvent is the copolymer architecture. Linear ABC triblock copolymers containing three chemically distinct polymer blocks have recently attracted increasing attention because they display a very large variety of properties leading thus to a wide range of specific application possibilities, such as pigment dispersant, surface modifier, and drug encapsulation [1,11–19]. Therefore, the micellization of such copolymers was widely investigated predominately in aqueous medium, as reported by different authors [11–13]. It was demonstrated that ABC triblock copolymers form usually core–shell–corona micelles in aqueous medium [20–24]. On the contrary, the self-aggregation studies of ABC triblock copolymers in selective organic solvents are relatively scarce [25,26]. To the best of our knowledge, there is, with the exception of a preliminary investigation of our group [1], no example where a given ABC triblock copolymer, such as poly(butadiene)-b-poly(2-vinylpyridine)-b-poly(ethylene oxide) (PBP2VPPEO), may lead to micelle formation as well in aqueous as in nonaqueous media. Moreover, because of the presence of P2VP middle block, this copolymer has the advantage of being pH sensitive by protonation. The understanding of the influence of the ABC triblock copolymer's composition on the micellar characteristics in organic medium remains a challenge.
The aim of the present study was therefore to examine the self-aggregation behavior of a series of well-defined PBP2VPPEO triblock copolymers in heptane, which is a typical selective solvent of the PB block, taken here as a model solvent. Dynamic light scattering (DLS) and viscometry were used to determine the colloidal characteristics, such as the hydrodynamic diameter, the aggregation number, and intrinsic viscosity, which were correlated to the molecular characteristics of block copolymers.
2 Experimental part
2.1 Materials
Butadiene (Fluka) liquefied at low temperature is stored under nitrogen atmosphere and purified by addition of n-BuLi (1.6 M solution in hexane, Aldrich) until the persistence of a light yellow color corresponding to the butadienyl anion. Ethylene oxide (EO) (Fluka), 2-vinylpyridine (VP) (Fluka) 1,1-diphenylethylene (DPE, Aldrich), and tetrahydrofurane (THF, Aldrich) are purified before use. Phenylisopropyl potassium (PIK) was synthesized from methylcumylether in the presence of sodium/potassium alloy according of Hruska et al. [27].
2.2 Polymerization
PBP2VPPEO block copolymers were prepared by sequential anionic polymerization in THF under dry nitrogen using PIK as an initiator.
After cryodistillation of THF into the glass reactor, the desired amount of PIK was added. The calculated amount of butadiene is then cryodistilled into the reactor kept at −65 °C. The polymerization was allowed to proceed at that temperature for 1–4 h. A small amount of the “living” PB solution was removed and quenched in methanol for characterization. The PB anion was then end-capped with DPE (mole ratio DPE/initiator = 2) to reduce its reactivity. The color of the solution changed from yellow to red. After decreasing the temperature to −78 °C, the required quantity of 2VP was diluted with an equal amount of THF that was added dropwise to the polymerization medium and the reaction was carried out during 2 h. In the last step, EO was cryodistilled into the reactor. The temperature was slowly increased to +25 °C and the polymerization was completed at this temperature during 48 h, followed by 24 h at 50 °C. The PBP2VPPEO triblock copolymer was deactivated with a few drops of acetic acid and recovered by vacuum drying for several days.
2.3 Characterization of the copolymers
The molecular weights and the molecular weight distribution were determined by gel permeation chromatography on an ALC/GPC 150 chromatograph (Millipore-Waters), equipped with four Microstyragel columns, refractive index, and UV detectors. The eluent was THF and commercially available polystyrene standards were used for calibration. The microstructure and the composition of the copolymer samples were determined by 1H NMR using CDCl3 as a solvent. The molecular characteristics of the copolymers used in this study are indicated in Table 1. The configuration of the PB sequence corresponds to 85–90 mol % 1, 2 for all samples.
Molecular characteristics of PBP2VPPEO triblock copolymers.
Samples | PB100P2VP100PEO104 | PB185P2VP108PEO154 | PB89P2VP145PEO125 | PB113P2VP67PEO218 | PB131P2VP219PEO322 |
Mn (PB)a (g mol−1) | 5400 | 10,000 | 4800 | 6100 | 7100 |
Mn (P2VP)b (g mol−1) | 10,500 | 11,400 | 15,300 | 7000 | 23,000 |
Mn (PEO)b (g mol−1) | 4600 | 6800 | 5500 | 9600 | 14,200 |
Wt % (PB)c | 26.3 | 35.5 | 18.7 | 26.9 | 16.0 |
Mn (PBP2VPPEO)a (g mol−1) | 20,500 | 28,200 | 25,600 | 22,700 | 44,300 |
Đ (PBP2VPPEO)a | 1.21 | 1.14 | 1.23 | 1.18 | 1.18 |
a Determined by gel permeation chromatography (GPC) calibrated with polystyrene (PS) standards using the “universal calibration”.
b Determined by GPC and 1H NMR.
c Determined by 1H NMR using CDCl3 as a solvent.
In the following, the copolymer samples are designated as PBxP2VPyPEOz, where x, y, and z represent the DPns of each block.
2.4 Preparation of the colloidal systems
The colloidal solutions in heptane could not be prepared by the dialysis techniques as no suitable membranes were available. Direct solubilization of the copolymer in heptane at 70 °C led to large amounts of aggregates in the colloidal solution. Therefore, it was preferred to start with a solution of the copolymer in a common solvent, such as THF, than to add an excess of heptane and finally to strip off the THF by azeotropic distillation at 64 °C under atmospheric pressure. The absence of THF in the final colloidal solutions was checked by gas chromatography. At this point, it should be mentioned that for these copolymers and for this preparation process, the micellar core might be “frozen-in” because of the glass transition temperature (Tg) of the P2VP of 72 °C and to a partial crystallization of PEO. Equilibrium between micelles and unimers, which is required for a strict definition of micelles, might therefore not exist in the present case. In the following, these colloidal particles are designated as micelle-like NPs.
2.5 DLS measurements
The intensity fluctuations of the scattered light at 90 °C were measured at 20 °C using a Coulter apparatus with a 32-channel correlator and a HeNe laser light source. The hydrodynamic diameter (Dh) was calculated using the classical Stokes–Einstein relation with extrapolation of the Dh values to zero concentration. The μ2/Γ2 ratio or “quality factor”, which is a measure of deviation from single exponentiality of the autocorrelation function, was used to evaluate the polydispersity of the colloidal systems. According to Debuigne et al. [28], low polydispersity values are furthermore admitted as an indication that the micelles have a spherical geometry.
All the solutions were filtered through Dynagard 0.45 μm polypropylene filters, and the concentration of the colloidal solutions ranged between 1 × 10−3 and 20 × 10−3 g ml−1.
2.6 Viscometry measurements
The intrinsic viscosities of the colloidal solutions were determined in an Ubbelohde-type Schott automatic viscometer with a photoelectric detection of flow times and a thermostatically controlled bath with a precision of ±0.01 °C. The data were fitted according to both equations of Huggins–Kraemer (Eq 1) and Heller (Eq 2).
(1) |
(2) |
3 Results and discussion
The colloidal characteristics of the copolymer samples were determined by viscometry and DLS, and then correlations were established between these properties and the molecular parameters of the triblock copolymers.
3.1 Colloidal characteristics
According to Tuzar and Kratochvil [29], the intrinsic viscosity of the colloidal solutions allows the qualitative evaluation of the compactness of micelle-like NPs. Therefore, the extrapolation to zero concentration by applying both the equations of Huggins–Kraemer and Heller was used to determine the [η] values for all the copolymer samples. These results are given in Table 2:
Micellar characteristics determined by viscometry using both the Huggins–Kraemer and Heller's theories.
Sample | Huggins–Kraemer | Heller | ||
[η] (cm3 g−1) | k | [η] (cm3 g−1) | k1 | |
PB100P2VP100PEO104 | 12.6 | 1.56 | 12.8 | 1.01 |
PB185P2VP108PEO154 | 15.4 | 1.25 | 15.6 | 0.87 |
PB89P2VP145PEO125 | 10.0 | 2.42 | 10.3 | 1.45 |
PB113P2VP67PEO218 | 16.3 | 2.21 | 17.0 | 1.09 |
PB131P2VP219PEO322 | 10.6 | 2.20 | 10.8 | 1.26 |
From the results given in Table 2, it appears that a good agreement exists between the intrinsic values obtained by applying the equations of Huggins–Kraemer and Heller.
The variation in the constants k and k1 as a function of the intrinsic viscosity determined by the equations of Huggins–Kraemer and Heller, respectively, are illustrated in Fig. 1.

Variation of k and k1 vs [η] determined by the equation of Huggins–Kraemer (squares) and Heller (diamonds), respectively.
According to Tuzar and Kratochvil [29], it was shown that the lower the intrinsic viscosity and the higher the Huggins constant, the more compact are the micelle. From Fig. 1, it can be noticed that the most compact micellar structure appears for copolymer PB89P2VP145PEO125 with the shortest PB sequence length. Conversely, the lowest compactness corresponds to copolymer PB185P2VP108PEO154, with the highest PB sequence length. With the exception of one sample (PB113P2VP67PEO218) it turns out as expected that the compactness decreases with increasing the PB content of the copolymers. This influence of the PB block, expressed by the molar ratio NB/NA, is clearly outlined in Fig. 2, which illustrates the correlation of both the intrinsic viscosity and k, determined using the equation of Huggins–Kraemer, as a function of molar ratio NB/NA with NB = DPnPEO + DPnP2VP.
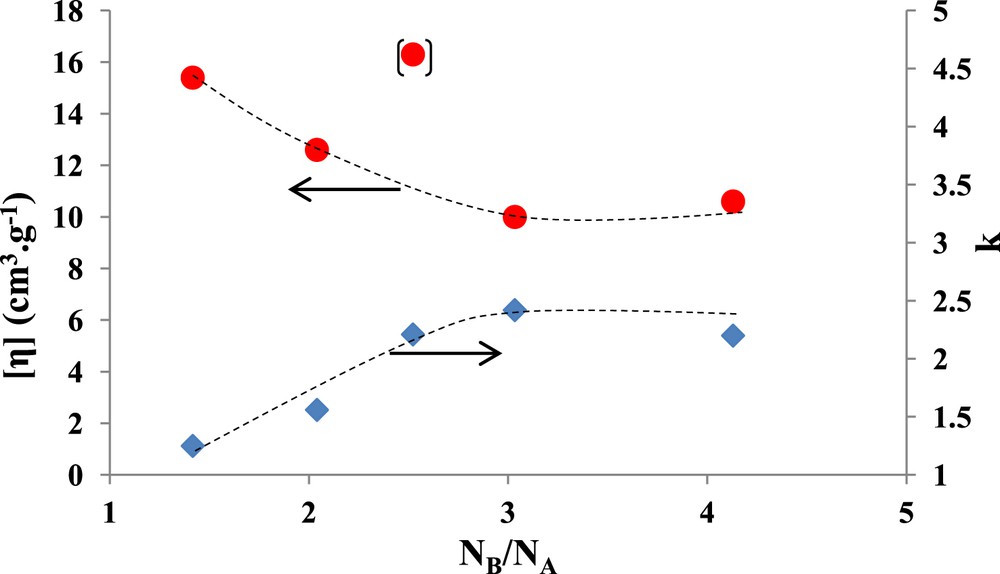
Variation of the intrinsic viscosity [η] and k, obtained by using the equation of Huggins–Kraemer, vs. NB/NA. NA = NPB and NB = NP2VP + NPEO.
Fig. 2 shows that a plateau regime is reached for high NB/NA ratio values and for intrinsic viscosity as for the k values.
Sizes of the micelle-like NPs were determined as a function of the concentration, and the extrapolation to zero concentration is provided in Fig. 3.

Extrapolation of Dh to zero concentration for samples PB100P2VP100PEO104 (cross), PB185P2VP108PEO154 (triangles), PB89P2VP145PEO125 (diamonds), PB113P2VP67PEO218 (squares), and PB131P2VP219PEO322 (circles), respectively.
Fig. 3 shows a slight decrease in the hydrodynamic diameters of the micelle-like NPs with increasing copolymer concentrations and that variation is more pronounced (the slope of the curve is more important as given in Table 3) for samples with lowest PB soluble sequence length. This behavior is in agreement with the statement of Konak et al. [30] according to which the micellar corona is compressed with increasing the copolymer concentrations. Moreover, from the data analysis provided in Fig. 3, a correlation coefficient greater than 0.99 was noticed for all curves. Colloidal characteristics, including the hydrodynamic diameters as determined from Fig. 3 by extrapolation to zero concentration, are given in Table 3.
Experimental colloidal characteristics of micelle-like NPs determined in heptane at 20 °C by extrapolation to zero concentration.
Sample | PB100P2VP100PEO104 | PB185P2VP108PEO154 | PB89P2VP145PEO125 | PB113P2VP67PEO218 | PB131P2VP219PEO322 |
Dh NPs (nm) | 45.2 ± 0.4 | 57.0 ± 0.8 | 48.4 ± 1.0 | 52.6 ± 0.8 | 75.2 ± 1.2 |
Slope | 0.590 | 0.334 | 0.648 | 0.363 | 0.455 |
Proportion of NPs (%) | 100 | 99.8 | 97.1 | 99.2 | 99.5 |
μ2/Γ2 | 0.04 | 0.04 | 0.06 | 0.05 | 0.05 |
From Table 3 it can be noticed that the Dh of NPs is in the range of 45–75 nm and that the μ2/Γ2 ratio related to the polydispersity of the micelles ranges between 0.04 and 0.06, indicating a narrow size distribution. Moreover, with the exception of sample with the lowest PB soluble sequence length PB89P2VP145PEO125, it can be observed that the aggregates content is less than 1%, and thus the NPs proportion is more than 99%.
From data given in Table 3, it was possible to correlate the Dh values with the total DPn N of the copolymers, as illustrated in Fig. 4.

Variation of the ln Dh vs. ln N. N = NPB + NP2VP + NPEO.
Fig. 4 shows that the hydrodynamic diameter scales with the total DPn of the copolymer samples, such as Dh ∼ N0.66. This exponent of 0.66 is in agreement with the scaling theory developed by Noolandi and Hong [8] for diblock copolymers.
3.2 Correlations between the colloidal and molecular characteristics of the triblock copolymers
In the following, an attempt was made to correlate the micelle-like NP characteristics, such as the aggregation number Z, the core Rc, and shell size Ls, respectively, to the molecular characteristics of the copolymers by considering that such triblock copolymers have a soluble block NA of PB and an NB insoluble moiety comprising the total number of 2VP and EO monomer units. In fact, according to Tuzar and Kratochvil [31], Einstein's viscosity laws allow an approximate evaluation of the mean molecular weight Mm of block copolymer micellar systems, such as , where N is the Avogadro's number (6.023 × 1023 mol−1). The average aggregation number Z corresponding to the average number of unimer chains in a micelle is given by the ratio Mm/Mn, Mn being the average molecular weight of the copolymer. From Mm, the molecular weight of the micelles, one could in fact also calculate the core size of the micelle (Rc), with the assumption that the core does not contain any solvent, according the following equation: , where WB is the weight fraction of the insoluble block and νB is the molar volume of the insoluble blocks in cm3 mol−1. The obtained results are given in Table 4.
Theoretical colloidal characteristics calculated from data obtained by DLS and viscometry.
Sample | N | Mm × 106 (g mol−1) | Z | Rca (nm) | Ls (nm) |
PB100P2VP100PEO104 | 304 | 5.66 | 276 | 11.3 | 11 |
PB89P2VP145PEO125 | 359 | 8.83 | 345 | 13.5 | 11 |
PB113P2VP67PEO218 | 398 | 6.67 | 294 | 11.8 | 15 |
PB185P2VP108PEO154 | 447 | 9.16 | 325 | 12.6 | 16 |
PB131P2VP219PEO322 | 672 | 31.14 | 703 | 20.7 | 17 |
A general tendency, observed in Table 4, is that the micellar characteristics, such as Mm, Z, and Rc, are related to the total DPn of the copolymer samples. The average thickness of the corona (Ls) formed by the soluble block of the copolymer has to be considered as a first approximation as it is calculated by the difference between Rh and Rc.
To have a closer insight into these correlations, an attempt was made to use existing scaling theories published for nonionic diblock copolymers. Quite a number of theories were developed in recent years for the prediction of the micellar characteristics, for example, hydrodynamic diameter (Dh), aggregation number (Z), core size, and so forth, as a function of the block copolymer molecular parameters such as their molecular weight, the relative degree of polymerization, and their architecture. However, these theories concern almost exclusively AB diblock and ABA triblock copolymers [8,10]. For ABC linear triblock copolymers mention may be made of Halperin's [9] theoretical approach concerning micelles having a C core and a shell with both A and B blocks, whereas in our case the micellar core comprises both PEO and P2VP blocks with a shell of heptane soluble poly(butadiene) block. This situation prompted us to suggest a simplified scaling outline based on the assumption that an ABC triblock copolymer might be considered as an “equivalent diblock copolymer”. In this simplified approach, the ABC triblock may be considered as AB′ diblock, having a heptane soluble A sequence, such as PB, and an insoluble B′ block comprising the P2VP and PEO sequences. To take into account the difference in molecular weights of the EO and 2VP monomer units, 44 and 105 g mol−1, respectively, the DPn of the PEO is expressed in equivalent P2VP DPns. The DPn of the insoluble B′ block is thus given by NB = NP2VP + (44/105)NPEO. The correlation of the aggregation number Z and that of the core size radius Rc as a function of the NB′ is given in Fig. 5:
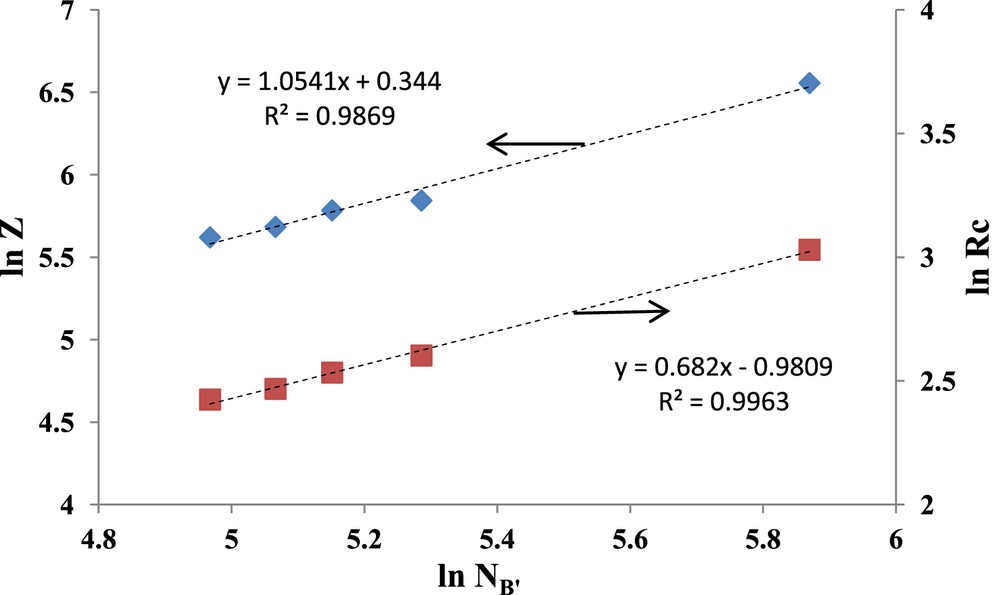
Variation of the Z (diamonds) and Rc (squares) vs. NB′, where NB′ = NP2VP + (44/105)NPEO.
In Fig. 5, a fair agreement may be noticed for the experimental Z and Rc scaling parameters with those reported by Zhulina and Birshtein [10] such as Z ∼ NB0.80 and Rc ∼ NB0.60 for diblock copolymers characterized by NA > NB0.80, a requirement that is also met for our copolymers. These results are an indication that the scaling theories developed for the self-aggregation of diblock copolymers might, in a first approximation, be extrapolated to ABC triblock copolymers.
4 Conclusions
The present study is an original contribution to the synthesis and self-aggregation behavior of ABC triblock copolymers. Well-defined poly(butadiene)-b-poly(2-vinylpyridine)-b-poly(ethylene oxide) (PBP2VPPEO) copolymers were prepared by sequential anionic polymerization. Micelle-like NPs were obtained, having a P2VPPEO core stabilized in heptane by a PB fringe. The intrinsic viscosity data and the corresponding virial coefficients revealed that the compactness of the micellar-like NPs in heptane decreases with increasing lengths of the PB sequence.
These particles are in the size range of 45–75 nm, as determined by DLS. From the intrinsic viscosity and the Dh values the aggregation number Z, as well the PEOP2VP core size as the PB fringe length, could further be evaluated. A clear correlation could be established between the hydrodynamic diameter Dh and total DPn of the copolymer samples, such as Dh ∼ N0.66. Finally, a simplified scaling approach was suggested to establish the correlations between the molar characteristics of the triblock copolymers and the aggregation number, as well as with the core size of the micelle-like NPs.
This study of nonaqueous micellar dispersions obtained with nonionic ABC triblock copolymers may open interesting perspectives for biomedical applications, such as for controlled release of hydrophilic and/or water sensitive drugs encapsulated in the dual compartment of the PEOP2VP core.
Funding
No funding was necessary for this study.