1 Introduction
Breaking and functionalization of a CH bond leading to a new CC bond formation is a challenging area in organic chemistry [1]. Traditionally, the carbon–carbon bond formation in preparation of aromatic carboxylic acids involves treatment of strongly organometallic compounds such as aryl palladium or aryl magnesium reagent [2] with CO [3] or CO2 [4]. The use of other substrates such as aryl halides has been reported in carboxylation reactions [5]. Arenes have also been used to produce aromatic carboxylic acids via direct carboxylation of the CH bond. Carboxylation of arenes with formic acid was developed [6]. It was reported that N-heterocyclic carbene metal complexes can efficiently catalyze carboxylation of heteroaromatic compounds [7]. Carboxylation of benzoxazole and benzothiazole derivatives with CO2 by heterocyclic copper complexes was reported [8]. Consequently, the introduction of a simple and efficient method without using transition metal and gaseous carbon dioxide or carbon monoxide is desirable, especially using reagents, which are more favorable to handle.
Trifluoromethanesulfonic anhydride, Tf2O, is a good reagent for the conversion of an OH group into an OTf leaving group [9]. Triphenylphosphine ditriflate (TPPD) was prepared exothermically as a white precipitate from the reaction of Tf2O with Ph3PO in CH2Cl2 [10]. This salt was presented as a powerful dehydrating agent and promoter (Scheme 1) [11].

Synthesis of TPPD.
As part of our ongoing efforts to develop electrophilic aromatic substitution [12], we have examined direct carboxylation of arenes using sodium hydrogen carbonate as a carboxylating agent, TPPD as a promoter, and ethanol as a solvent at room temperature under transition metal-free conditions (Scheme 2).

Synthesis of carboxylic acids.
2 Experimental section
2.1 General information
All the chemicals were obtained from Merck, and used as received. All products are known and characterized by a comparison of their spectra (1H NMR, 13C NMR) with those reported in the literature. All yields refer to the isolated products. NMR spectra were recorded on a Bruker Avance spectrophotometer (400 MHz) in CDCl3 and used as an internal standard.
2.2 General procedure for the carboxylation of arenes
The fresh obtained TPPD (0.672 g, 1.2 mmol) was dissolved in 3 mL of ethanol, and then sodium hydrogen carbonate (0.084 g, 1 mmol) was added into the solution. The resulting mixture was stirred for 30 min at room temperature. Then, mesitylene (0.14 mL, 1 mmol) was added into the solution and the stirring continued for 60 min at room temperature. The reaction completion was monitored by thin layer chromatography. After the completion of reaction, the solvent was removed under reduced pressure, and the saturated sodium bicarbonate solution (10 mL) was added to the medium. The product was extracted with chloroform (3 × 5 mL) and the organic layer washed with water and dried over anhydrous MgSO4. The filtrate was evaporated and the crude product was purified by silica gel column chromatography using ethyl acetate/n-hexane (3:7) as eluent to afford 2,4,6-trimethylbenzoic acid (0.131 g, 89%).
3 Results and discussion
The initial study was directed toward exploring the reaction conditions for the carboxylation of mesitylene using NaHCO3/TPPD system. We first tested the suitability of several different solvents for the carboxylation of mesitylene with NaHCO3 promoted by TPPD. It was observed that the nature of the solvent has a considerable effect on the reaction rate and the yield of product, and EtOH was found to be the most effective solvent (Table 1, entry 4). Other solvents including H2O-EtOH (1:1), H2O-EtOH (1:2), H2O-EtOH (2:1), CH2Cl2, CH3CN, and CHCl3 were substantially less effective. Water was not effective at all due to the low solubility of arenes at the reaction temperature. To study the effect of the amount of TPPD, different amounts of the promoter (0.5, 1.0, 1.2, 1.4, and 2.0 mmol) were chosen. The results show that 1.2 mmol of the promoter per millimoles of mesitylene afforded the best results. An increase in the amount of TPPD to 2 mmol shows no significant difference with respect to the yield. The reaction was not complete when less than 1.2 mmol of the promoter was used. An increase in the temperature from room temperature to reflux condition indicates that the yield was almost unaffected. As a result, the optimized reaction conditions are TPPD (1.2 equiv) in EtOH at room temperature. The presence of the promoter is essential to the reaction, because no reaction occurs in the absence of it.
Effect of the solvent on the carboxylation reaction of arenes.a
Entry | Solvent | Time (min) | Yield (%)b |
1 | H2O–ethanol (1:1) | 120 | 37 |
2 | H2O–ethanol (1:2) | 95 | 67 |
3 | H2O–ethanol (2:1) | 120 | 30 |
4 | Ethanol | 60 | 89 |
5 | H2O | 120 | 0 |
6 | CH2Cl2 | 120 | 0 |
7 | MeCN | 120 | 0 |
8 | CHCl3 | 120 | 0 |
9 | n-hexane | 120 | 0 |
a Mesitylene (1 mmol), sodium hydrogen carbonate (1 mmol), and TPPD (1.2 mmol) in EtOH at room temperature.
b Isolated yield.
To examine the generality of this procedure, we investigated the reactions using a wide range of arenes as substrates under the present reaction conditions. The results are summarized in Table 2. The carboxylation reaction can be carried out with aromatic compounds without consideration of the type of substituent. Aromatic compounds with both electron releasing and electron withdrawing groups afforded the corresponding products in moderate to good yields. Of course, the arenes with electron donating groups reacted faster than those with electron acceptor groups and afforded better yields. Naphthalene was converted to 1-naphthoic acid with high regioselectivity in 53% yield (Table 2, entry 16). The carboxylation of nitrobenzene with electron acceptor group occurred in 25% yield (Table 2, entry 8). m-Dinitrobenzene and dichlorobenzene were also reacted and produced the corresponding carboxylic acids in 20% and 57% yields, respectively (Table 2, entries 11 and 15). Notably, not only carboxylation of nitrobenzene with strong electron withdrawing group occurred, but also m-dinitrobenzene can be carboxylated.
Synthesis of aromatic carboxylic acids from sodium hydrogen carbonate using TPPD.
Entry | ArH | Product | Time (min) | Yield (%)a |
1 | 90 | 65 | ||
2 | 95 | 60 | ||
3 | 90 | 60 | ||
4 | 70 | 65 | ||
5 | 70 | 60 | ||
6 | 120 | 40 | ||
7 | 70b | 67 | ||
8 | 120 | 25 | ||
9 | 120 | 38 | ||
10 | 70 | 67 | ||
11 | 95 | 57 | ||
12 | 65 | 75 | ||
13 | 65 | 70 | ||
14 | 60 | 89 | ||
15 | 120 | 20 | ||
16 | 90 | 53 |
a Isolated yield.
b Trace amount of p-isomer.
Of course, the yields of their products were low due to their strong electron withdrawing groups and incompletion of the reaction. Carboxylation of pyridine occurred and pyridine-3-carboxylic acid was obtained in 38% yield (Table 2, entry 9).
The carboxylation reactions of toluene, chlorobenzene, bromobenzene, anisole, and fluorobenzene were carried out with high regioselectivity and afforded their corresponding products in 65%, 60%, 60%, 60%, and 40% yields, respectively (Table 2, entries 1, 2, 3, 5, and 6). The excellent regioselectivity of these reactions under the present reaction conditions is considerable. It seems that steric effect has an important role in the reactions. The high selectivity of these reactions might be because of the presence of the highly hindered carboxylating reagent, which does not permit ortho position to carboxylate.
Interestingly, thiophenol was reacted under the reaction conditions and 2-sulfanylbenzoic acid was obtained as the product with high regioselectivity, whereas phenol afforded p-hydroxybenzoic acid. The result of thiophenol carboxylation reaction is unexpected. It was reported that electrophilic reagents generally attack the sulfur atom of thiophenol and ring substitution is rarely occurred [13]. This selectivity might be due to an intramolecular rearrangement similar to the Fries rearrangement [14]. It was reported that Fries rearrangements of formyl groups can also be induced by trifluoromethanesulfonic acid [15]. In addition, triphenylphosphine oxide released during the reaction may act as a Lewis acid for the Fries rearrangement.
The intermediate 2 was isolated and identified by NMR. The peaks of protons at 1.2 and 3.9 ppm observed as a triplet and quartet in the 1H NMR spectrum ascribed to CH3 and CH2 of the ethyl group. The peaks at 7.2–8.6 ppm related to aromatic CH bonds. The 13C NMR spectrum of intermediate 2 shows the presence of an ethyl group by the peaks of carbons at 21.6 and 55.4 ppm. The carbon atoms of aromatic rings can be confirmed by the peaks at 116–147 ppm. These data indicate that the intermediate 2 was produced during the reaction.
Our attempts to isolate the intermediate 3 [(PPh3-OCO-OH)+ −OTf] from the sodium triflate salt were not successful. Hence, the FT-IR and NMR spectra of the mixture of these two salts were taken (see Supplementary Material). The presence of intermediate 3 during the process of the carboxylation reaction might be confirmed by the observation peak at 2500–3010 cm−1 related to the stretching vibrations of the hydroxy group and the broad peak at 1680 cm−1 corresponding to the carbonyl group, which are the characteristic peaks of the carboxyl group. In the 1H NMR spectrum of the mixture, the peaks of protons of CH bonds ascribed to aromatic CH bonds, and the peak related to OH bond of the carboxyl group were observed at about 7–8 and 13.2 ppm, respectively, and confirmed the presence of phenyl and carboxyl groups in the intermediate 3. Furthermore, the 13C NMR spectrum indicates the presence of carboxylating agent 3 by the peaks of carbons of aromatic rings at 118–138 ppm and a carbonyl group at 188 ppm. It seems that the intermediate 3 was formed during the process of the reaction.
The intermediates 1 and 3 as highly electrophile species reacted with highly nucleophilic ethanol. The feasibility of these reactions can be concluded when the reaction of mesitylene with sodium hydrogen carbonate under the same reaction conditions in a less polar solvent such as chloroform, acetonitrile, and dichloromethane and n-hexane as a nonpolar solvent did not proceed. These results showed that ethanol has an important role in the carboxylation reaction. TPPD (intermediate 1) reacted with ethanol and afforded ethoxytriphenylphosphonium triflate, 2. The ethoxy group in compound 2 was protonated in the presence of produced TfOH and became a good leaving group when it was attacked by sodium hydrogen carbonate as a nucleophile and the intermediate 3 was obtained. Ethanol attacked to CO group of the intermediate 3. In this case, EtO-CO-OH (4), Ph3P=O, and TfOH were obtained as products and mesitylene attacked to the carbonyl group of unstable intermediate 4 in the presence of TfOH to produce the corresponding carboxylated products. The other possibility is attack of EtOH to phosphorous of phosphonium ion in the intermediate 3 to obtain intermediate 2, carbon dioxide, and water. It seems that this reaction does not occur because no CO2 bubbling was observed during the reaction. In addition, we expected to obtain the ethylated aromatic compound as a product but this arene was not produced.
As a result of this observation, the following mechanism indicated in Scheme 3 was proposed for the synthesis of aromatic carboxylic acids promoted by TPPD. The resulting reagent was used for successful carboxylation of broad range of arenes via an ordinary electrophilic aromatic substitution reaction.
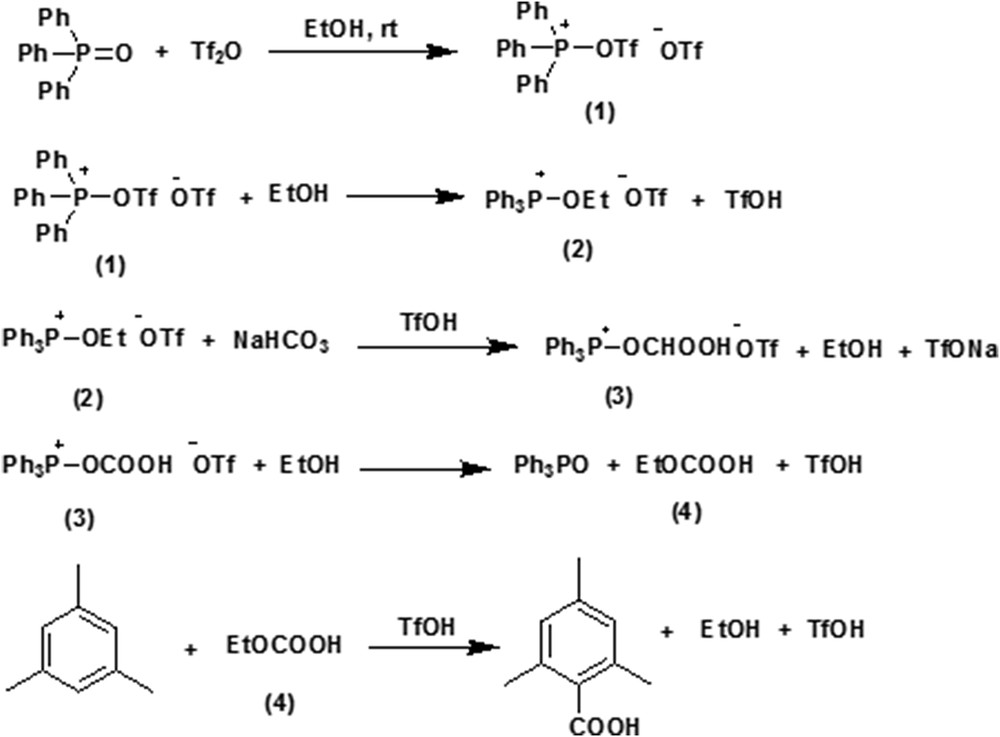
Proposed reaction mechanism for the synthesis of aromatic carboxylic acids.
4 Conclusion
We have developed a novel approach for direct carboxylation of a wide range of aromatic compounds in moderate to good yields with high regioselectivity using NaHCO3/TPPD system in EtOH. This metal-free protocol does not need to use carbon monoxide or carbon dioxide gases. The unreactive aromatic compounds such as m-dinitrobenzene can also be carboxylated under the reaction conditions. The o-carboxylation of thiophenol was unexpected due to its regioselectivity. Readily available aromatic compounds, toleration of various functional groups, short reaction times, and performance at room temperature are other advantages of this method [16].
Acknowledgments
The authors thank the Razi University for providing the generous financial support to this work.