1 Introduction
Biguanides are compounds of importance due to their biological properties, of which worth to be mentioned are the antimicrobial, antifungal, antibacterial, hypoglycemic, antimalarial, and antitumor activities [1–8]. The special interest in studying the coordination complexes in which the ligand is of biguanide type is given by the fact that many of them possess biological properties.
Thus, complexes of Fe(III), Ni(II), and Cu(II) with N,N-dimethylbiguanide ligands and their derivatives [Fe(DMBG)2]Cl·0.5H2O, [FeO(DMBG)2], [Ni(DMBG)2], [Cu(DMBG)2]·H2O, where HDMBG = N,N-dimethylbiguanide [9–11], [Cu(HTBG)2]Cl2 and [Cu(TBG)2]·3H2O, where HTBG is 2-tolylbiguanide [12], are known.
Biological, spectral, and molecular docking studies of 1-(o-tolyl)biguanide demonstrated its antimicrobial activity [13].
α-Ketoglutaric acid plays an essential role in the Krebs cycle; α-ketoacids are very important agents in the synthesis and degradation of amino acids and proteins in the metabolism of lipids and carbohydrates [14]. Studies on α-ketoacids with biological activity have shown that they can produce different effects on the biological tissue, interacting at the cellular level at different pH values, temperatures, and enzyme-controlled medium [15–17].
α-Ketoglutaric acid antagonizes toxic effects against both HCN and NaCN [18,19]. It has also a neuroprotective effect, helps in the healing and regeneration of tissues, in the development of the gastrointestinal tract, in lung disorders, and some types of cancer [14].
Complexes with lanthanides containing α-ketoglutaric acid as ligand have been synthesized [20].
Complexes with formula [M2(III)M(II)L6(NO3)6(OH2)6](NO3), where M(III) = Ce and M(II) = Cu, Co, Ni, and L is α-ketoglutaric acid, have antibacterial properties and antioxidant activity [21].
Taking into account the biological properties of α-ketoglutaric acid and 1-(o-tolyl)biguanide, we aimed to synthesize and afterward study the properties of new complex combinations of Ni(II) with these ligands.
2 Experimental section
2.1 Materials and methods
The substances used to obtain the four complexes were of high purity and came from Alfa Aesar (α-ketoglutaric acid—C5H6O5) and Sigma–Aldrich (1-(o-tolyl)biguanide—C9H13N5, NiCl2·6H2O, NiBr2, Ni(NO3)2·6H2O, Ni(CH3COO)2·4H2O, and ethanol).
For synthesis of complex combinations, the substances used (nickel salts and two ligands) were dissolved in ethanol. Reactions occurred at approximately 40 °C under agitation. The complexes obtained were vacuum filtered, washed with ethanol, and dried with ethyl ether. The molar ratio of metal salts/α-ketoglutaric acid/1-(o-tolyl) biguanide was 1:1:1 using 1 mmol of each substance (0.1461 g C5H6O5, 0.1913 g C9H13N5, 0.2377 g NiCl2·6H2O, 0.2185 g NiBr2, 0,2908 g Ni(NO3)2·6H2O, and 0.2488 g Ni(CH3COO)2·4H2O). Synthesis of complex combinations led to pure compounds, which did not require further purification.
The nitrogen, carbon, and hydrogen content of the synthesized complexes was determined by microcombustion using a Flash 2000 Organic Elemental Analyzer. The presence of chlorine in complex C1 was revealed with AgNO3, and the percentage of nickel was determined using a Perkin Elmer AAnalyst 400 atomic absorption spectrometer.
Electronic spectra were recorded using a Jasco V670 spectrometer by the diffuse reflection method at room temperature in the range 200–1500 nm, using MgO as a standard.
A Nicolet IS 50 FT-IR spectrophotometer was used for recording FT-IR spectra in the 4000–200 cm−1 range. Thermal analysis was performed with a simultaneous thermogravimetric analysis/differential scanning calorimetry (TGA/DSC) STA 449 F1 Jupiter working in a dynamic air atmosphere at a flow rate of 20 mL/min in the range 25–900 °C with a heating rate of 10 °C/min. The molar electrical conductivity was determined using a CyberScan PCD 6500 conductivity meter in 10−3 M of N,N-dimethylformamide solution at 25 °C. Magnetic susceptibility was measured with a Gouy balance using the Faraday method and Hg[Co(SCN)4] as a calibrant.
Antitumor activity was tested in HeLa tumor cells using the MTT (3-(4,5-dimethylthiazol-2-yl)-2,5-diphenyltetrazolium bromide) assay at a concentration of 500 μg/mL for 24 h incubation at 37 °C in 5% CO2 atmosphere. The method is based on the following: under certain conditions, NAD(P)H-dependent cellular oxidoreductases may reflect the number of viable cells in a culture. These enzymes may reduce the MTT tetrazolium reagent to violet insoluble formazan (Fig. 1). The color intensity of formazan was evaluated by measuring optical density at a wavelength of 570 nm using a Filter Max F5 Multi-Mode Microplate Reader spectrophotometer.
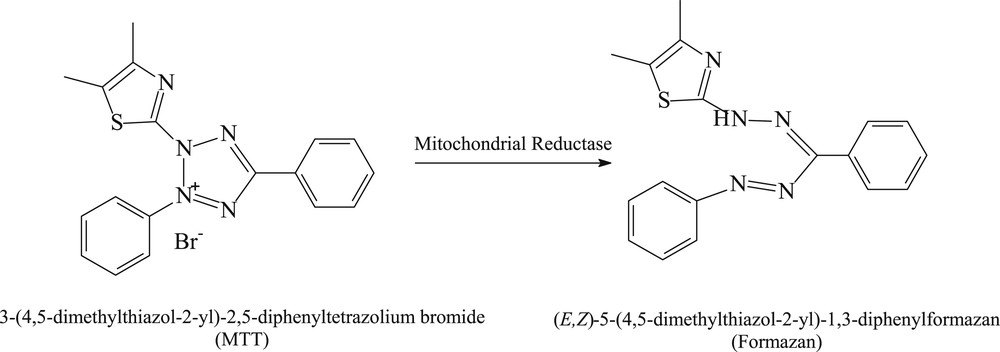
The scheme of reducing the MTT reagent and obtaining formazan.
The tetrazolium dye reduction method is often used to measure the cytotoxicity of some compounds and cell proliferation. Because MTT reagent is sensitive to light, the whole procedure is done in the dark.
The antibacterial activity of ligands and complexes synthesized was determined in vitro against Pseudomonas aeruginosa and Staphylococcus aureus bacterial strains. To determine the minimum inhibitory concentration (MIC), the broth microdilution method was used by successively diluting a 10 mg/mL complex combination solution using 96-well plates. According to the dilution scheme of complex compounds, the influence of a diluted dimethyl sulfoxide (DMSO) solvent was also quantified.
To determine the influence of the test compounds on the adhesion of a microbial biofilm to the inert substrate, the microbial cells were cultivated in the presence of compounds of interest into nutrient broth using 96-well plates. They were incubated at 37 °C for 24 h. The plates were emptied and washed twice with physiological sterile water, and the adhered cells were fixed with 0.1 mL of 80% methanol for 5 min. The methanol solution was removed by overturning, and the adhered cells were stained with 1% crystal violet alkaline solution (0.1 mL/well) for 15 min. The staining solution was removed and then the plates were washed under water stream. The microbial biofilms formed on the plastic plates were converted into suspension by bubbling in 33% acetic acid. The intensity of the suspension color was evaluated by measuring the absorbance at 492 nm using a Max F5 Multi-Mode Microplate Reader.
3 Results and discussion
3.1 Elemental analysis
To establish the formulas of coordination compounds that were synthesized, elemental analysis was performed. A good concordance between calculated and experimental percents of carbon, nitrogen, hydrogen, and nickel was found (Table 1).
The content of C, H, N, and Ni in C1, C2, C3, and C4 complexes.
Complex | Nitrogen (%) | Carbon (%) | Hydrogen (%) | Nickel (%) | ||||
Exp. | Calc. | Exp. | Calc. | Exp. | Calc. | Exp. | Calc. | |
C1 | 14.82 | 15.01 | 36.13 | 36.05 | 4.58 | 4.75 | 12.87 | 12.58 |
C2 | 13.97 | 13.71 | 33.13 | 32.91 | 4.63 | 4.34 | 11.75 | 11.49 |
C3 | 17.51 | 17.69 | 35.89 | 35.40 | 4.12 | 4.24 | 12.07 | 12.36 |
C4 | 15.77 | 15.42 | 42.44 | 42.32 | 4.98 | 4.66 | 12.74 | 12.93 |
3.2 Thermal analysis
On the basis of the interpretation of the results of the TGA, information has been obtained that has contributed to the proposed formulations for coordination complexes, namely, the presence of water molecules and the thermal effects associated with mass loss processes. Thermogravimetric (TG) and DSC curves of the four complexes are presented in Fig. 2(a–d).

TG and DSC curves of the complexes (a) [Ni(TB)(HA)(H2O)2]Cl, (b) [Ni(TB)(HA)(H2O)2]Br, (c) [Ni(TB)(HA)]NO3·H2O, and (d) [Ni(TB)(HA)]CH3COO.
From the analysis of the C1 complex TG and DSC curves, the first decomposition step (<180 °C) indicates the loss of two water molecules (experimental loss, 8.12%; calculated loss, 7.72%). In the range of 180–280 °C, chlorine anion is lost as HCl (experimental loss, 7.67%; calculated loss, 7.82%). Ligand decomposition occurs in the third and fourth steps, with strong exothermic effects in the 280–450 °C range.
In case of complex C2 up to approximately 200 °C, we have a mass loss of 7.50%, indicating the presence of two coordinating water molecules. In the range of 200–270 °C, bromine anion is lost as HBr (experimental loss, 16.25%; calculated loss, 15.85%). Above this temperature, the mass loss corresponds to the decomposition of the ligand being accompanied by exothermic effects.
Analyzing the TG and DSC curves of the C3 complex, it is observed that in the first step (<180 °C) the crystallization water molecule is lost (experimental loss, 3.74%; calculated loss, 3.79%). Subsequently, the removal of nitrate ions as nitrogen oxides and oxidative degradation of the ligand takes place. Consequently, in the temperature range 400–500 °C, a strong exothermic effect can be observed.
The thermal analysis curves of the C4 complex indicate that the acetate ion was eliminated as CO2 and H2O up to about 250 °C (experimental loss, 13.50%; calculated loss, 13.00%) and the ligand degradation takes place up to 500 °C.
For all four complexes analyzed, the residue obtained is NiO (greenish gray), from which the percentage of nickel was determined, namely, for C1 (experimental, 12.73%; calculated, 12.58%), for C2 (experimental, 11.28%; calculated 11.49%), for C3 (experimental, 11.98%; calculated, 12.36%), and for C4 (experimental, 13.42%; calculated, 13.00%). A good agreement was observed between the spectrophotometrically determined metal content and the corresponding value of the residue from thermal analysis.
3.3 Molar conductivity
All molar conductivities were determined at 25 °C using N,N-dimethylformamide as a solvent and concentrations of 10−3 M. The measured values for complexes are as follows: C1, 79.5; C2, 81.7; C3, 72.6; and C4, 77.2 S cm2 mol−1. These values indicate a 1:1 electrolyte type for all four complexes [22].
3.4 Magnetic susceptibility
Magnetic susceptibility determinations have led to the following magnetic moment values: 3.19 μΒ for C1, 3.27 μB for C2, and zero for both C3 and C4. Consequently, the first two complexes are paramagnetic, whereas C3 and C4 complexes are diamagnetic.
3.5 Ultraviolet–visible–near-infrared spectra
The stereochemistry of synthesized coordination complexes has been assessed using ultraviolet, visible, and near-infrared (UV–vis–NIR) spectrometry by comparing their spectra to those of the ligands (α-ketoglutaric acid and 1-(o-tolyl)biguanide). The electron spectra of the solid state C1C4 complexes are shown in Fig. 3. The bands and the maxima assigned to the d–d transitions for the four synthesized complexes are shown in Table 2.

The electronic spectra of the complexes (a) [Ni(TB)(HA)(H2O)2]Cl, (b) [Ni(TB)(HA)(H2O)2]Br, (c) [Ni(TB)(HA)]NO3·H2O, and (d) [Ni(TB)(HA)]CH3COO.
Maxima of d–d transition bands for C1C4 complexes.
Complex | Observed bands | Assignments | Symmetry | |
λmax (nm) | ύ (cm−1) | |||
C1 | 1200 | 8330 | 3B1 → 3A1 | Rhombic distorted octahedral |
750 | 13,330 | 3B1 → 3B2 | ||
675 | 14,810 | 3B1 → 3B2 (3T1) | ||
490 | 20,410 | 3B1 → 3A2 (3T1) | ||
415 | 24,100 | 3B1 → A2, 3B1,3B2 (3T1/3P) | ||
C2 | 1155 | 8660 | 3B1 → 3A1 | Rhombic distorted octahedral |
745 | 13,420 | 3B1 → 3B2 | ||
670 | 14,925 | 3B1 → 3B2 (3T1) | ||
485 | 20,620 | 3B1 → 3A2 (3T1) | ||
400 | 25,000 | 3B1 → A2, 3B1,3B2 (3T1/3P) | ||
C3 | 680 | 14,600 | 1A1g → 1A2g | Square-planar |
465 | 21,510 | 1A1g → 1B1g | ||
C4 | 680 | 14,710 | 1A1g → 1A2g | Square-planar |
450 | 22,220 | 1A1g → 1B1g |
All complexes have absorption bands in the range of 230–345 nm that may me attributed to the π–π* and n–π* transitions of organic ligands H2A and TB, slightly displaced by coordination of the ligand to metal ions.
The electronic spectra of the C1 and C2 complexes are very similar and correspond, by the position and intensity of the visible range bands, to a rhombic distorted octahedral symmetry [23].
The broad cleavage of the first and second octahedral bands cannot be explained on the basis of a tetragonal deformed octahedral symmetry. The existence of two different bidentate ligands in the plane and two water molecules in axial positions, that is, a chromophore NiO2N2O2/2NiO (α-ketoglutaric acid), 2NiN (biguanide), and 2NiO(H2O), leads to a rhombic deformation of the octahedron and a symmetry that corresponds, with good approximation, to C2v [23]. Under these conditions, the bands of 8330/8660 and 13,330/13,420 cm−1 correspond to the transitions from the fundamental state 3B1 to the terms 3A1 and 3B2 of the term 3T2g in the octahedral symmetry.
The other observed peaks that follow in the spectra correspond to the transitions to terms 3B2 and 3A2 from the 3T1g(F) term of the octahedral symmetry. The cleavage of the third octahedral band that involves transitions to terms derived from the 3T1g(P) level is not observed in any of the complexes. The position of all the observed bands is in agreement with the literature data regarding complexes having oxygen and nitrogen donor atoms and analogous symmetry [24–27].
C3 and C4 complexes do not have bands below 10,000 cm−1 (which are characteristic of octahedral symmetry), their first bands being at 14,600 and 14,710 cm−1, respectively.
The symmetry of these complexes is square-planar, in agreement with the observed diamagnetism and the fundamental term is 1A1g. In agreement with the literature data, the observed peaks correspond to the first and second bands in D4h symmetry (square-planar) [28–30].
The electronic spectra of the four complexes dissolved in DMSO were also recorded. It has been observed that the positions of the bands due to the metal ion do not change from those in the solid state, which means that the proposed symmetries are maintained. The electronic spectra of C1C4 complexes dissolved in DMSO are shown in Fig. 4.
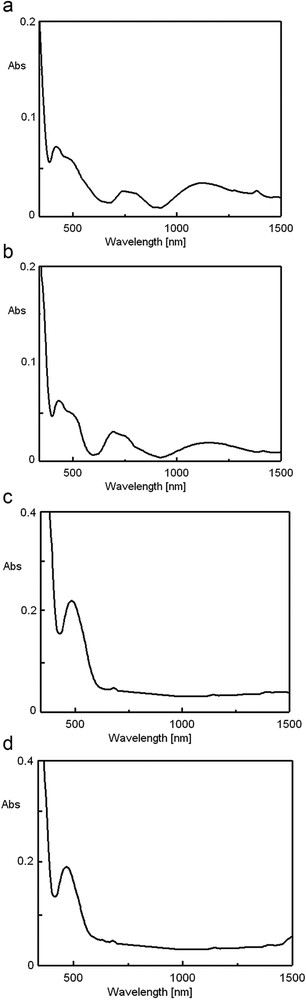
The electronic spectra in DMSO of the complexes (a) [Ni(TB)(HA)(H2O)2]Cl, (b) [Ni(TB)(HA)(H2O)2]Br, (c)[Ni(TB)(HA)]NO3·H2O, and (d) [Ni(TB)(HA)]CH3COO.
3.6 FT-IR spectra
To determine the coordination mode of the ligands to the metal ion, the FT-IR spectra of the C1C4 complexes were compared with those of the H2A and TB ligands [31]. The characteristic spectral bands and their assignments are shown in Table 3. To determine the coordination pattern of the H2A ligand, the IR spectrum of sodium α-ketoglutarate was also analyzed, the difference between the wavelengths corresponding to the bands ν(COO−)asym and ν(COO−)sym being 181 cm−1.
IR bands and their assignments for the synthesized complexes and ligands.
Assignments | 1-(o-Tolyl)biguanide | α-Ketoglutaric acid | C1 | C2 | C3 | C4 |
ν(CO)keto | 1720vs | 1690vs | 1682vs | 1695vs | 1692vs | |
ν(CN) | 1610vs | 1635vs | 1648vs | 1660vs | 1651vs | |
δ(NH) + ν(CN) | 1577m | 1587m | 1595m | 1570m | 1580m | |
1270w | 1252w | 1257w | 1255w | 1244w | ||
ν(COO−)asym | 1532vs | 1544vs | 1588vs | 1595vs | ||
ν(COO−)sym | 1387s | 1392s | 1445s | 1432s | ||
145 | 152 | 143 | 163 | |||
ν3(NO3) | 1390vs | |||||
ν1(NO3) | 1072m | |||||
ν2(NO3) | 837m | |||||
ν4(NO3) | 710s | |||||
ν(CO)acetate | 1570s | |||||
ν(CO)acetate | 1410s | |||||
ν(NiN) | 450m | 442m | 432m | 440m | ||
ν(NiO) | 268m | 270m | 264m | 260m | ||
ν(ΟΗ)water | 3390m | 3410m | 3370m | |||
ρr coord. water | 780m | 765m | ||||
ρw coord. water | 602s | 617s |
In the spectra of the four complex combinations, a band shift due to the valence vibration of the imine group, ν(CN), from 1610 cm−1 to higher values is observed. This is in agreement with the coordination of the TB ligand to the metal ions through the lone pair of imine nitrogen. The displacement of this band to larger wavelengths is explained by the destruction of the π electron delocalization [32]. Coordination of 1-(o-tolyl)biguanide by iminic nitrogen atoms is also supported by the band displacement due to δ(NH) + ν(CN) coupled vibration.
The displacement of the ν(CO) band below 1720 cm−1 confirms that the keto group in the α position of the H2A ligand is involved in the coordination for all the analyzed complexes.
From the difference between the corresponding wavenumbers of ν(COO−)asym and ν(COO−)sym bands (<181 cm−1), it was established that the H2A ligand coordinates with the metal ion in its deprotonated form HA−, through α oxygen atom of the ketone group and the oxygen from the hydroxyl of the adjacent carboxyl group.
The presence of a single absorption band for the characteristic vibration modes ν1–ν4 in the C3 complex spectrum indicates the presence of a nitrate anion in the ionization sphere.
In the C4 complex, the intense bands at 1570 and 1410 cm−1 can be attributed to the out of the coordination sphere ionic acetate.
Bands between 260 and 270 cm−1 and 432 and 450 cm−1, respectively, were assigned to the formation of NiO and NiN bonds.
The coordination water in the C1 and C2 complexes is confirmed by the presence of the three characteristic bands located at approximately 3400, 600, and 700 cm−1. In the case of C3 complex, the stretched band from 3370 cm−1 is attributed to the crystallization water.
3.7 Biological activity
Metabolism of HeLa cells varies depending on the type of the material used. Thus, ligands and C1 and C2 complexes show a very weak effect on HeLa tumor cells under the tested conditions, whereas complex C3 and C4 combinations have demonstrated a moderate cytotoxic effect on them. Fig. 5 shows the absorbance readings at 570 nm for the analyzed samples (at a concentration of 500 μg/mL for 24 h incubation at 37 °C) and untreated control sample indicating the metabolic activity of HeLa cell cultures by the MTT method.
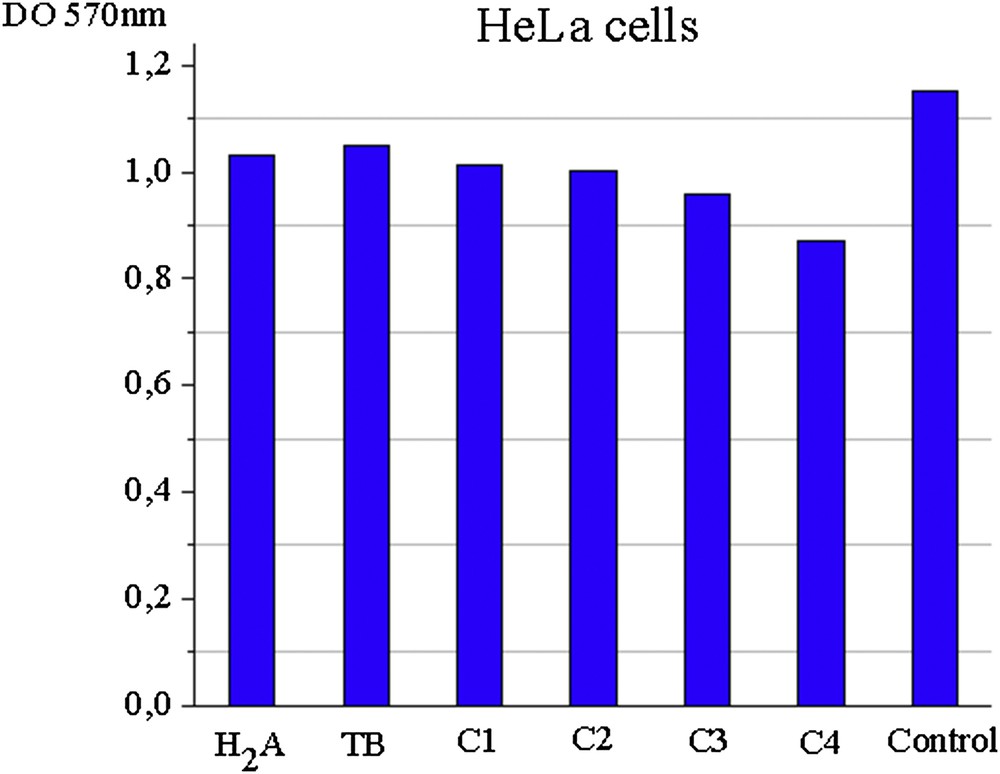
Absorbance values at 570 nm for ligands and complexes of HeLa cell cultures.
Evaluation of the antimicrobial activity of the ligands and synthesized complexes was done on S. aureus and P. aeruginosa species. It has been observed that DMSO (the solvent used in dilutions) does not influence the antimicrobial activity of the tested compounds at the chosen working concentrations.
In the case of Gram-positive S. aureus, C2 has shown identical activity to ligands, whereas C1, C3, and C4 complexes show a better activity. Thus, C4 has the best activity, whose MIC value is 0.75 mg/mL.
The activity of complexes synthesized (except C2) against Gram-negative bacteria P. aeruginosa is better for synthesized complexes than for H2A ligand but similar to TB ligand. C4 complex has the best activity among the tested substances against these bacteria (MIC is 1.00 mg/mL). MICs for the ligands and C1C4 complexes against the two bacterial strains are shown in Figs. 6 and 7.
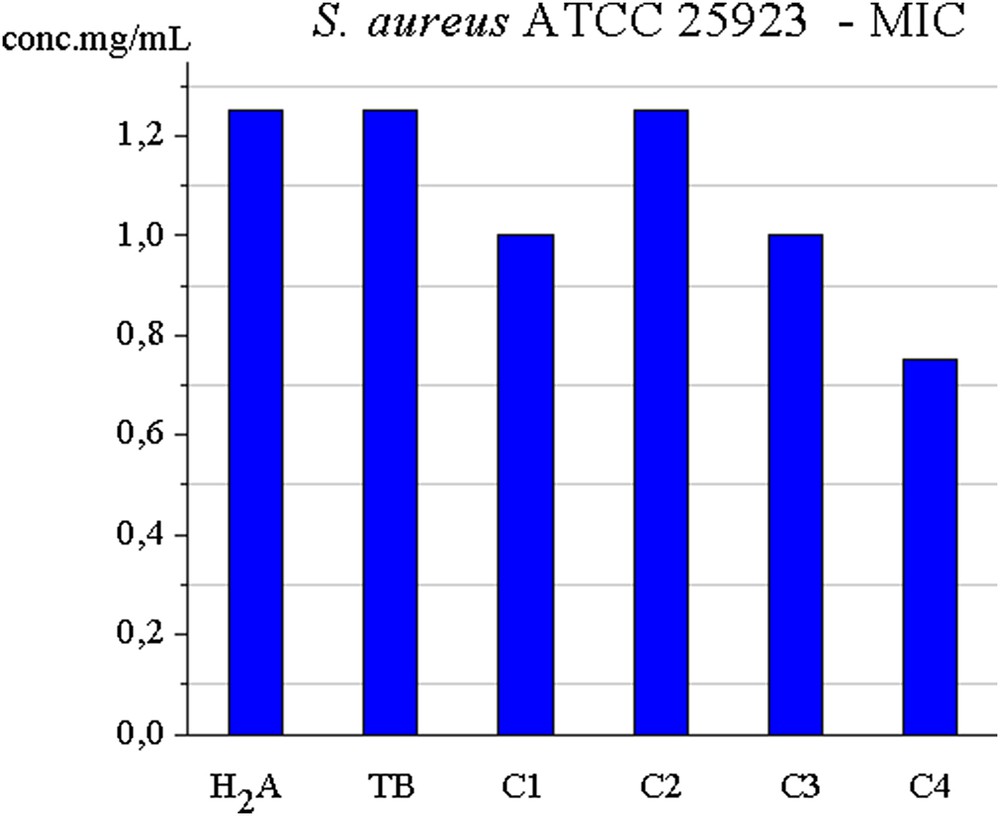
MIC for C1C4 ligands against S. aureus.
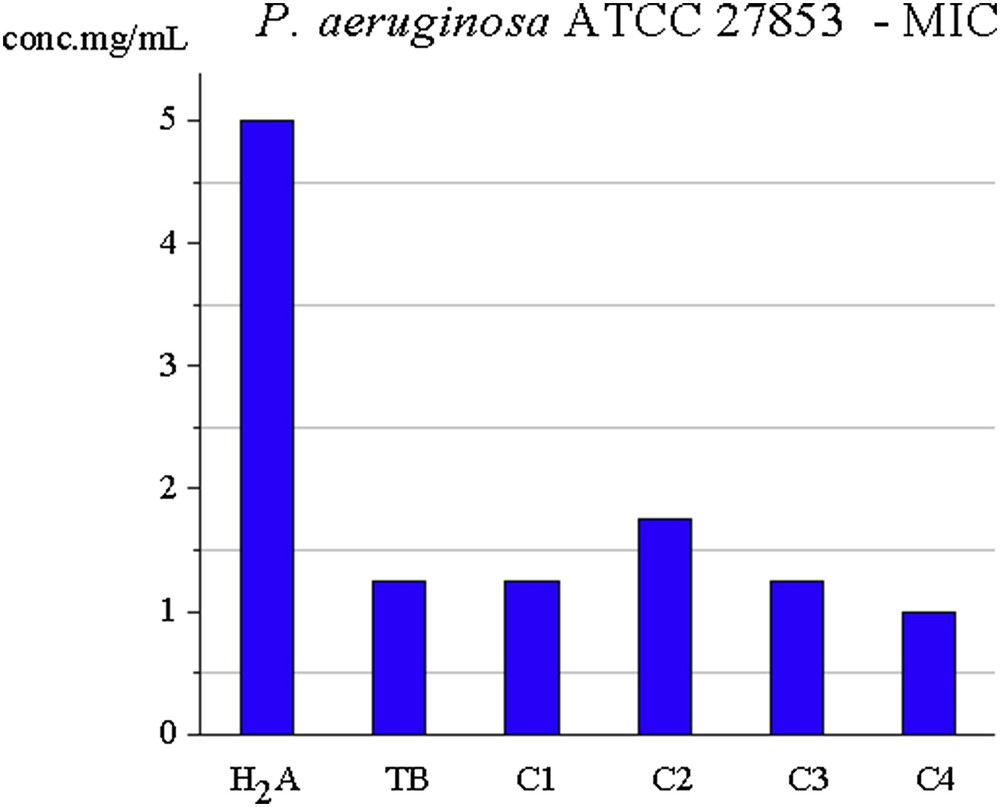
MIC for C1C4 ligands against P. aeruginosa.
Regarding the ability to inhibit the adhesion of the microbial biofilm to the inert substrate, in S. aureus, all the test compounds (ligands and complexes obtained) inhibit this process in a dose-dependent manner to a minimum biofilm eradication concentration of 0.04 mg/mL (C3, C4), 0.09 mg/mL (TB, H2A, C2), and 0.18 mg/mL (C1). Against P. aeruginosa, all compounds have inhibitory capacity up to a minimum biofilm eradication concentration of 0.04 mg/mL (TB, C1C4) and for H2A of 0.09 mg/mL.
The influence of ligands and complexes on adhesion to inert substrate capacity is shown in Figs. 8 and 9.
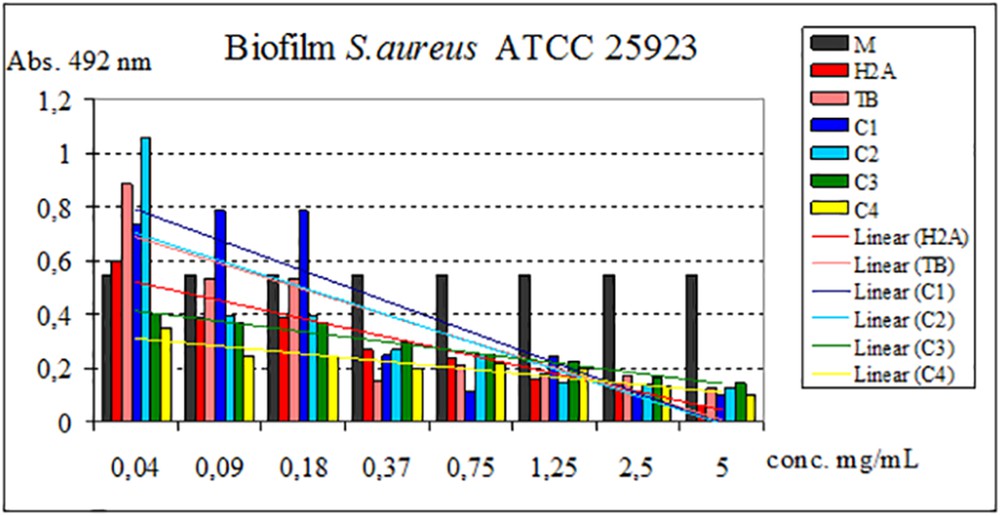
Influence of H2A, TB, and C1C4 on adhesion to the inert substrate of the strain S. aureus.
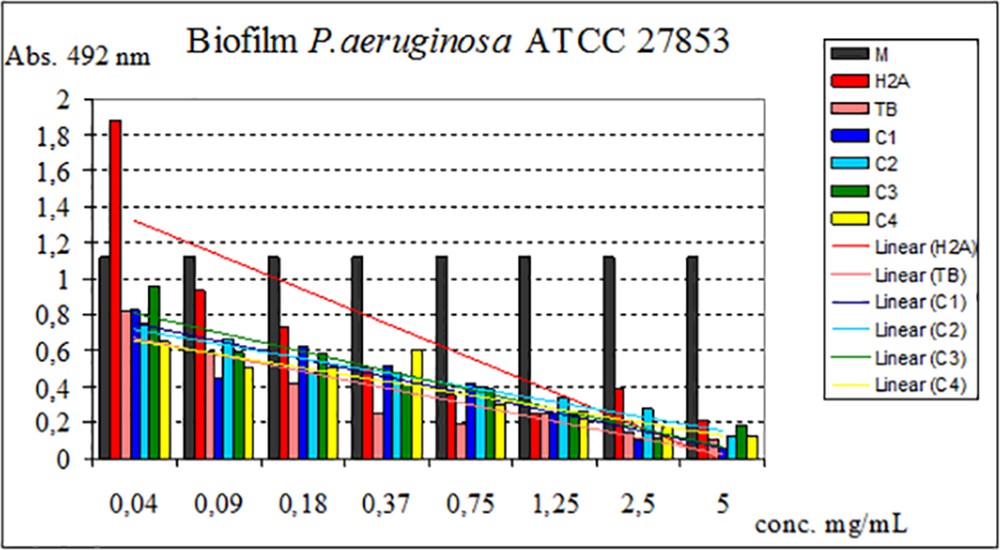
Influence of H2A, TB, and C1C4 on adhesion to the inert substrate of the strain P. aeruginosa.
4 Conclusions
Four nickel coordination complexes having α-ketoglutaric acid and 1-(o-tolyl)biguanide were synthesized and characterized.
On the basis of the analyses performed (elemental analysis, UV–vis–NIR spectra, IR, thermal analysis, molar electric conductance, and magnetic susceptibility), the formulas of C1C4 complexes were proposed.
Both α-ketoglutaric acid and 1-(o-tolyl)biguanide function as bidentate ligands in the four complexes (the structures of the two ligands) are shown in Fig. 10.
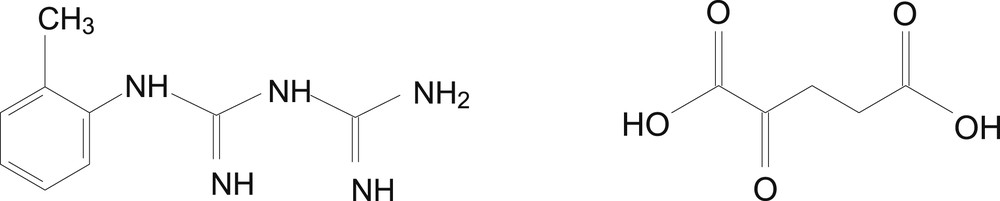
Structures of TB and H2A ligands.
For the synthesized complexes, the following formulas for complex cations were proposed (Fig. 11a and b).
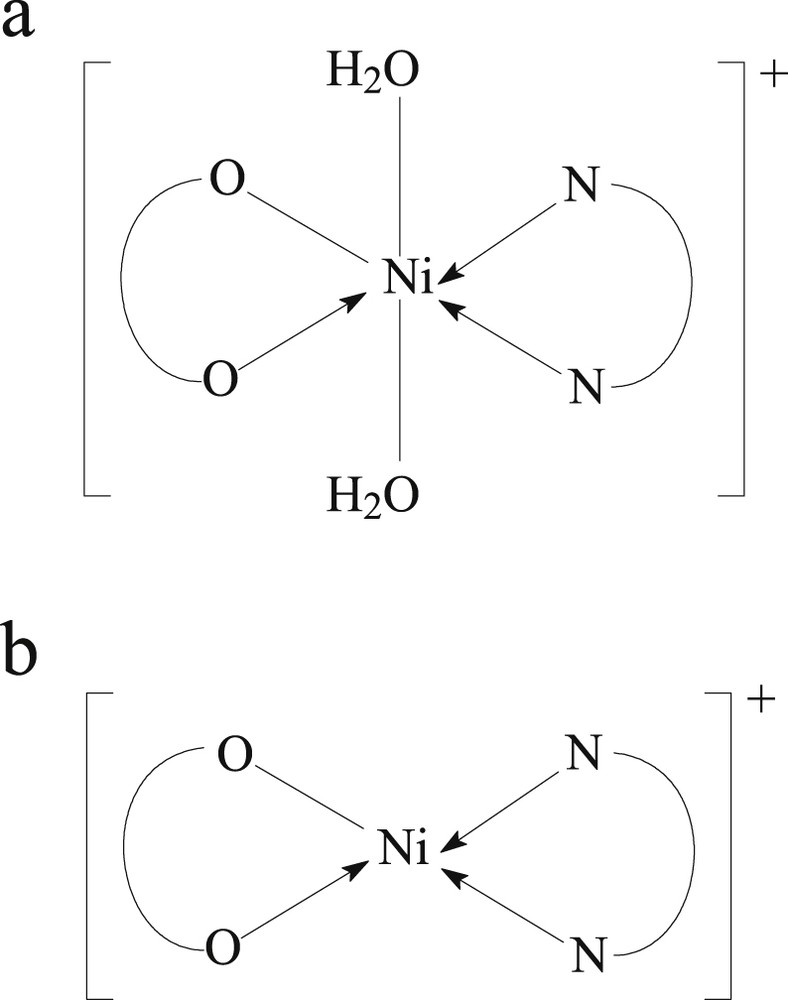
Complex cation structure for (a) C1 and C2 and (b) C3 and C4.
The antitumor activity in HeLa tumor cells of these complexes and the ligands used in the synthesis was tested. The best cytotoxic effect on this type of cell has the C4 complex. Concerning the antibacterial activity of the synthesized complexes against S. aureus and P. aeruginosa, this is comparable or better than that of the ligands (C3 and C4 showing the best activity).
Because the C1C4 complexes are of the electrolyte type, their activity may result from the electrostatic interaction of the complex cation of these species with the negatively charged components of the cell membrane, consequently leading to their inactivation. On the other hand, antimicrobial activity may be associated with stereochemistry and the combined effect of the ligand and metal ion to inactivate a particular component involved in the pathogenesis of the microorganism.
The different activity of the C1C4 complexes can be attributed to their different stereochemistry. Better biological activity was observed for square-planar symmetry complexes (C3 and C4) than octahedral (C1 and C2).
Acknowledgment
This work was supported by the UEFISCDI through PN-II-PT-PCCA-2013-4-0891 project: Innovative dental products with multiple applications no. 229/2014.