1 Introduction
One of the hottest topics in the current scientific literature is the design of sustainable procedures for the valorization of lignocellulosic biomass from agricultural, industrial, and forest residues [1,2] via extractions using bio-based solvents [3–6], and microwave (MW) and ultrasound (US) irradiations [7–10]. The major challenge in efficient biomass exploitation is still the reduction of lignin and hemicellulose within their complex lignocellulosic structure to facilitate subsequent cellulose conversion to value-added chemicals via either enzymatic hydrolysis or catalytic procedures [11]. Lignin is widely treated as a waste product in bioethanol production, it is mainly recycled as fuel for energy balance [12], but further valorization is worthy of investigation [13,14], as it is the second most abundant natural polymer after cellulose [15]. Lignin is a three-dimensional highly cross-linked macromolecule made up of three main phenyl propane units (monolignols), namely, coniferyl alcohol (G), sinapyl alcohol (S), and minor amounts of p-coumaryl alcohol (H), which are interwoven by a series of characteristic linkages, such as CeOeC and CeC bonds [16,17].
In particular, the destruction of the majority of lignin ether bonds during the biomass delignification process generally results in an increased phenolic hydroxyl group content in the lignin structure. These compounds are essential for the product's radical scavenging activity [18]; they can trap radicals and become phenoxyl radicals themselves. Furthermore, the methoxyl groups located in the ortho position play an important role in phenoxyl radical stabilization [19], which is also a step toward the capture of radicals and the subsequent antioxidant effect of lignin [20–22]. Lignin has two main advantages as an antioxidant: it is of natural origin and is a polymeric structure. Antioxidant polymers have been a topic of great interest for researchers in many industrial fields [23], meaning that lignin and lignin-related polymers have been started to be used as additives in biodegradable packaging materials [24], as food additives [25], and in cosmetics products [26]. However, the high chemical heterogeneity of lignin also makes it necessary to consider how antioxidant activity can be influenced by biomass processing conditions [27,28]. Delignification processes may affect the functional groups and molecular weight of recovered lignins, conditioning their antioxidant properties. For this reason, finding an effective and sustainable means of recovering lignin from lignocellulosic biomass may be the first step toward its valorization as an antioxidant. It has recently been demonstrated that the extraction of lignin from wheat straw (WS), using organic solvents of different polarity and alkaline solutions, results in the isolation of products with good antioxidant activity [27], whereas a plethora of biological, chemical, and physical methods have been reported for lignin extraction from a variety of biomass sources [29]. However, these methods are all arduous, time-consuming, and entail high solvent and chemical consumption. Besides the complete redesign of all existing processes, the use of alternative solvents, together with the so-called enabling technologies, may also be a means to increase the efficiency of lignin extraction protocols [30]. Focusing on green extraction and mass transport enhancing methods, such as microwave-assisted extraction (MAE) and ultrasound-assisted extraction (UAE), may provide the necessary potential to accomplish faster and more gentle processes that consume less solvent than conventional methods [31]. In fact, the last few decades have seen enabling technologies being widely exploited as efficient and sustainable alternatives to traditional lignocellulose pretreatment methods [32,33]. In particular, the sonomechanical effect of US has been shown to enhance solvent penetration into cellular materials, thus improving the mass transfer of the extractive processes behind biomass pretreatment [34]. On the other hand, the MW-assisted technique has been postulated as an alternative heating technology and one that is able to reduce reaction times and improve extract efficiency and quality, compared with conventional extraction protocols [35,36]. MWs interact with polar molecules leading to the rapid and volumetric heating of irradiated mixtures and mass transfer optimization. With this efficient and selective heating, MWs can also be considered a promising approach to the thermal treatment of biowaste, especially lignocellulose [37]. However, of these numerous studies, only a few focus on MW-assisted lignin isolation and the majority of these work under acidic conditions [38,39]. Nevertheless, they do highlight the advantages that MW-assisted lignin isolation can offer, especially in terms of lignin purity and processing time. The present study reports the MW-assisted delignification of WS (one of the most abundant agricultural residues) and demonstrates the synergism that can exist between the so-called enabling technologies and bio-based solvents.
The concept of green solvents is strongly related to the principles of green chemistry, and natural deep eutectic solvents (NaDESs) have received much more recent attention than the others available [40]. A deep eutectic solvent (DES) is a fluid generally composed of two or three inexpensive and safe components that are capable of self-association, often through hydrogen-bond interactions, to form a eutectic mixture with a melting point lower than that of each individual component. DESs, similarly to the more traditionally used ionic liquids (ILs), are generally liquid at temperatures less than 100 °C. Their synthesis is 100% atom-economic and their purity is high. However, unlike ILs, they are nontoxic and biodegradable. A wide range of NaDESs have been applied across a number of chemistry fields. Particularly, noteworthy is the choline chloride series that are coupled with monosaccharides (mainly glucose and fructose), glycerol, and organic acids, which act as hydrogen bond donors [41,42]. Moreover, NaDESs have recently been used as extraction solvents for phenolic compounds [43].
The nonvolatility of NaDESs is considered to be the most challenging issue in the design of separation processes, as solvents can no longer be separated by simple distillation. For this reason, other green solvents have been tested in lignin extraction experiments, including those that arise from the conversion of biomass itself. γ-Valerolactone (GVL) is a polar aprotic solvent conventionally produced from biomass-derived levulinic acid [44] and its esters via (catalytic) hydrogenation [45]. GVL shows a number of interesting features [46]; its polarity is similar to those of the most common polar solvents (GVL's dielectric constant is 36.47, whereas those of CH3CN, DMF, NMP, and DMA are 37.5, 36.7, 32.0, and 37.8, respectively). Furthermore, it has low melting and high boiling points (−31 and 207 °C respectively) and a flash point 96 °C, which is generally higher than that of conventional dipolar aprotic solvents. It is also quite stable under neutral conditions. GVL has recently been used as a solvent in the extraction of lignin from biomass in a cascade MW-assisted integrated process [45].
In this study, the delignification power of NaDES and GVL on WS has been maximized using US and MW irradiations. Moreover, the antioxidant property of extracts was used to assess the best extraction procedure in terms of product quality [47]. The extracts showed good antioxidant properties, thus paving the way for the application of lignin as an added value product, as an alternative to its combustion, according to the biorefinery approach. We herein demonstrate that nonconventional extraction techniques using bio-based solvents, GVL and NaDES, may be considered a valid alternative to classical methods, which use organic solvents coupled with alkali or acid treatments.
2 Materials and methods
2.1 Plant material
Milled WS homogenous powder (0.2 mm) was provided by Environmental System GmbH (Germany) and was protected from light and humidity until use.
2.2 Chemicals
All chemicals were purchased from Sigma–Aldrich (Milan, Italy) and used without further purification. NaDESs were obtained via heating: choline chloride (1 mol) was mixed with hydrogen bond donors: lactic acid (10 mol) to produce NaDES 1, and a combination of lactic acid (1 mol) and glycerol (1 mol) to produce NaDES 2. The mixtures were stirred for 2 h at 100 °C until homogeneous liquids were obtained. NaDES 1 and NaDES 2 were finally collected for biomass extraction without further purification.
2.3 Biomass delignification procedures under nonconventional techniques
2.3.1 Ultrasound-assisted extraction
UAE was performed in a high-power ultrasonic bath (Weber Ultrasonics AG, Germany) composed of an inox cell (5 L) with three probes screwed into the bottom of the bath, which was operated at a frequency of 40 kHz and at maximum input power of 200 W. The suitable weight of WS powder (0.2 mm particle size) was placed in a 250 mL round-bottomed flask and immersed in the ultrasonic bath equipped with inlet and outlet for cooling water circulation. The temperature in the ultrasonic bath was stabilized at either 35 or 50 °C during sonication (60–120 min). GVL and NaDESs were tested at a solid/liquid ratio of 1:50 (200 mL maximum liquid volume), whereas a variety of GVL/H2O ratios were investigated, as was a combination of bio-based solvents and NaOH (10% in the dry biomass). After UAE, the mixture was filtered off and washed with pure water.
WS solid fraction workup (after GVL extraction): the solid recovered after filtration was treated with US irradiation (40 kHz, 200 W for 30 min at 50 °C) in the presence of an aqueous NaOH solution (1% of NaOH in the dry biomass) to test the effectiveness of NaOH in degrafting GVL from the straw surface. This procedure was adopted for both the GVL-UAE5 and GVL-UAE6 samples. The suspension was then filtered and the treated WS was freeze-dried (−70 °C, 0.2 mbar).
WS solid fraction workup (after NaDES extraction): the solid recovered after filtration was thoroughly washed with pure water until complete eutectic solvent removal was achieved, and the treated biomass was then freeze-dried under vacuum (−70 °C, 0.2 mbar).
WS liquid fraction workup (after GVL extraction): the recovered liquid fraction was used without any further purification.
WS liquid fraction workup (after NaDES extraction): an aqueous NaOH solution (1 M) was added to the recovered liquid fraction until neutrality was achieved and then used without any further purification.
2.3.2 Microwave-assisted extraction
MAE was performed by a multimode MW reactor in a closed vessel system SynthWAVE (Milestone Srl). Reactor frequency was 2.45 GHz. The different bio-based solvents (GVL and NaDES) were tested for WS pretreatment at a solid/liquid ratio of 1:50 (20 mL maximum liquid volume). A variety of GVL/H2O ratios were tested for WS MAE at 90–120 °C under 1500 W, as was a combination of bio-based solvents and NaOH (10% in the dry biomass).
WS solid fraction workup (after GVL extraction): the solid recovered after filtration was treated under conventional heating (for 30 min at 50 °C) in the presence of an aqueous NaOH solution (1% of NaOH in the dry biomass) to test the effectiveness of NaOH in degrafting GVL from the straw surface. This procedure was adopted for both GVL-MAE2 and GVL-MAE4 samples. The suspension was then filtered, and the treated WS was freeze-dried (−70 °C, 0.2 mbar).
WS solid fraction workup (after NaDES extraction): the solid recovered after filtration was thoroughly washed with pure water until the eutectic solvent was completely removed and the treated biomass was freeze-dried (−70 °C, 0.2 mbar).
WS liquid fraction workup (after GVL extraction): the recovered liquid fraction was used without any further purification.
WS liquid fraction workup (after NaDES extraction): an aqueous NaOH solution (1 M) was added to the recovered liquid fraction until neutrality was achieved, which was then used without any further purification.
2.4 Liquid fraction antioxidant activity
2.4.1 2,2-Diphenyl-1-picrylhydrazyl radical tests
Antioxidant activity was determined using the radical scavenging activity method with the 2,2-diphenyl-1-picrylhydrazyl (DPPH) radical [48]. A lignin sample, recovered after water precipitation from each liquid extracts (NADEs and GVL), was dissolved in 0.1 mL dioxane–water (9:1, v/v), and the solution was added to a 3.9 mL DPPH solution (25 mg/L in ethanol), used as the free radical source. The blank sample consisted of 0.1 mL methanol and a 3.9 mL DPPH solution. After 30 min of incubation period at room temperature in the dark, the decrease in solution absorbance caused by proton-donating activity was measured immediately at 515 nm using a UV spectrometer (Cary 60 UV–Vis, Agilent Technologies). The tests were carried out in duplicate. Radical scavenging activity was calculated as follows: I (%) = (A0 − A1)/A0, where A0 is the absorbance of the blank and A1 is the absorbance in the presence of the test compound at the various concentrations. IC50 values (concentrations that provide 50% inhibition) were calculated graphically using a calibration curve in the linear range by plotting the extract concentration versus the corresponding scavenging effect.
2.4.2 Total phenolic contents
The total phenolic contents (TPCs) of the liquid fractions were determined according to the method described by Singleton and Orthofer [49]. Briefly, each sample of liquid extracts was diluted with distilled water 50 times and 1 mL of the diluted solution was added to 5 mL of 0.2 M Folin–Ciocalteu reagent. After 5 min of gentle shaking, 4 mL of 7.5% (w/w) sodium carbonate was added and mixed thoroughly. After 30 min of storage in dark, optical density of the resulting blue complex was measured at 765 nm against the blank on a PerkinElmer Lambda 650 UV–Vis spectrophotometer, using gallic acid as the standard. The TPC was expressed as milligrams of gallic acid equivalents per 100 mL of solution.
2.5 Py-GC/MS/FID analysis
Py-GC/MS/FID analyses were performed using a Micro Double-shot Pyrolyzer Py-3030D (pyrolysis temperature 500 °C, heating rate 600 °C s−1; Frontier Laboratories, Japan), which was directly coupled with a Shimadzu 2D FID/MS gas chromatography system MSGC/GCMS-2010 (Japan) with a capillary column RTX-1701 (Restek, USA; 60 m × 0.25 mm × 0.25 μm film, injector temperature 250 °C, ion source 250 °C with an EI of 70 eV, MS scan range m/z 15–350, the carrier gas was helium at a flow rate of 1 mL min−1, and a split ratio of 1:30). Sample masses ranged from 1.00 to 2.00 mg. The oven program was as follows: 1 min at 60 °C and the temperature was then increased at 6 °C min−1 to 270 °C before it was finally held at 270 °C for 10 min. The identification of the individual compounds was performed on the basis of GC/MS chromatograms using Library MS NIST 14, whereas the relative peak areas of the individual compounds were calculated using the Shimadzu software on the basis of GC/FID data. The summed molar areas of the relevant peaks were normalized to 100%. Relative peak areas calculated in % rel for the pyrolysis products of different origin were used to assess the composition of the biomass samples.
2.6 Solid sample surface characterization
The nitrogen adsorption–desorption isotherm method was used to characterize the microsurfaces of raw WS and biomass samples. The isotherms were measured using a Quantachrome Gas Sorption System Nova 4000 (Quantachrome Instruments, USA) at a temperature of −196 °C according to the recommendations of the International Union of Pure and Applied Chemistry [50].
3 Results and discussion
Bioderived solvents and NaDESs are currently attracting increasing amounts of interest for use in biomass delignification because they exhibit low toxicity and are fully biodegradable [51]. It has recently been proven that pretreatment with either choline chloride/glycerol or choline chloride/lactic acid boosts enzymatic digestibility and enhances the delignification of date palm residues and corncobs [52,53]. Meanwhile, the use of GVL/H2O mixtures for the fractionation of lignocellulosic biomass in an MW reactor has recently been reported [54]. Some recent articles have reported the use of US in biomass pretreatment [55], as well as that of NaDES for the UAE of catechins from green tea [56]. However, this technology has yet to be studied in combination with biomass-derived solvents for biomass delignification to the best of our knowledge.
In this study, a variety of processes have been tested for their ability to perform WS (containing 20.8% lignin) delignification in the presence of bio-based solvents and the results have been compared with those of more traditional methods.
Two different NaDESs, a lactic acid/choline chloride mixture (10:1) (NaDES 1) and a three-component (lactic acid/choline chloride/glycerol 1:1:1) mixture (NaDES 2), have been used to study the synergism between organic acids and alcohols and their effect on extraction efficacy. The two DESs exhibit similar physicochemical properties to ILs (such as [BMIM][Cl]) [57], while also being much cheaper and potentially environmentally friendly. The extraction capacity of NaDESs is also correlated with their physical properties, including polarity and viscosity. DESs display high ionic conductivity as a result of their high ion concentrations, which is a positive feature in terms of their MW responsiveness. The major disadvantage of NaDESs, when compared with conventional solvents, is their inherently high viscosity. Viscosity is known to hinder the efficiency of NaDESs as extraction solvents because it results in slow mass transfer. This disadvantage has been negated here by the efficient heat and mass transfer provided by MW and US irradiations.
Despite extensive research into NaDESs, there is still a lack of information as to the practical issues, including their efficiency, optimal water content, and the recovery of extracted compounds related to their use as extraction solvents. The inherent low vapor pressure that NaDESs display hampers evaporation, meaning that extracted compounds can be only recovered via selective adsorption onto a suitable stationary phase. However, the whole NaDES extract can be used in nonfood applications [53].
Two different GVL/H2O mixtures (1:1 and 8:2) were adopted for lignin extraction from WS under MW and US irradiations. These ratios were chosen to test the specific role that GVL plays with respect to water. The delignification and antioxidant activity of liquor recovered from these alternative lignin extraction protocols were compared with those of the extracts of the water/alkali treatment.
WS was US irradiated for 60 min at 40 kHz and 200 W power (temperature 35 or 50 °C) at a solid/liquid ratio of 1:50 using water as a reference solvent (Table 1, entries 2 and 3). The same treatment was reproduced, but 10% of NaOH was added to the dry biomass (Table 1, entries 4 and 5), whereas bioderived solvents were also used for comparison (Table 1).
Py-GC/MS/FID results: summarized relative abundance (%) of lignin-derived pyrolysis products and the ratio of relative S- and G-type phenol contents (S/G ratio) as detected for untreated and pretreated WS biomass.
Entry | Sample | Extraction method | Lignin derived in volatiles (% rel.) | S/G ratio |
1 | WS | Nontreated | 20.8 ± 0.1 | 1:1.7 |
2 | H2O-UAE1 | 35 °C, 60 min, H2O, US (40 kHz, 200 W) | 18.2 ± 0.1 | 1:1.8 |
3 | H2O-UAE2 | 50 °C, 60 min, H2O, US (40 kHz, 200 W) | 19.0 ± 0.2 | 1:1.8 |
4 | NaOH-UAE1 | 35 °C, 60 min, H2O + 10% NaOH, US (40 kHz, 200 W) | 17.0 ± 0.1 | 1:1.7 |
5 | NaOH-UAE2 | 50 °C, 60 min, H2O + 10% NaOH, US (40 kHz, 200 W) | 16.0 ± 0.1 | 1:1.6 |
6 | GVL-UAE1 | 35 °C, 60 min, 8:2 GVL/H2O, US (40 kHz, 200 W) | 16.1 ± 0.2 | 1:1.8 |
7 | GVL-UAE2 | 50 °C, 60 min, 8:2 GVL/H2O, US (40 kHz, 200 W) | 16.2 ± 0.1 | 1:1.8 |
8 | GVL-UAE3 | 35 °C, 60 min, 8:2 GVL/H2O + 10% NaOH, US (40 kHz, 200 W) | 20.1 ± 0.1 | 1:1.5 |
9 | GVL-UAE4 | 50 °C, 60 min, 8:2 GVL/H2O + 10% NaOH, US (40 kHz, 200 W) | 18.6 ± 0.1 | 1:1.6 |
10 | GVL-UAE5 | 35 °C, 60 min, 8:2 GVL/H2O, US (40 kHz, 200 W)a | – | – |
11 | GVL-UAE6 | 50 °C, 60 min, 8:2 GVL/H2O, US (40 kHz, 200 W)a | – | – |
12 | GVL-UAE7 | 35 °C, 60 min, 1:1 GVL/H2O, US (40 kHz, 200 W) | 17.9 ± 0.2 | 1:1.6 |
13 | GVL-UAE8 | 50 °C, 60 min, 1:1 GVL/H2O, US (40 kHz, 200 W) | 15.3 ± 0.1 | 1:1.9 |
14 | GVL-UAE9 | 35 °C, 60 min, 1:1 GVL/H2O + 10% NaOH, US (40 kHz, 200 W) | 19.2 ± 0.2 | 1:1.5 |
15 | GVL-UAE10 | 50 °C, 60 min, 1:1 GVL/H2O + 10% NaOH, US (40 kHz, 200 W) | 18.0 ± 0.2 | 1:1.6 |
16 | GVL-MAE1 | 90 °C, 30 min, 1:1 GVL/H2O, MW (1500 W) | 15.1 ± 0.1 | 1:1.8 |
17 | GVL-MAE2 | 90 °C, 30 min, 1:1 GVL/H2O, MW (1500 W)b | – | – |
18 | GVL-MAE3 | 90 °C, 30 min, in 8:2 GVL/H2O, MW (1500 W) | 15.2 ± 0.1 | 1:1.8 |
19 | GVL-MAE4 | 90 °C, 30 min, in 8:2 GVL/H2O, MW (1500 W)b | – | – |
20 | NaDES-UAE1 | 50 °C, 120 min, in NaDES 1 (lactic acid/choline chloride (10:1)), US (40 kHz, 200 W) | 14.6 ± 0.1 | 1:1.7 |
21 | NaDES-UAE2 | 50 °C, 120 min, in NaDES 2 (lactic acid/glycerol/choline chloride (1:1:1)), US (40 kHz, 200 W) | 15.5 ± 0.1 | 1:1.9 |
22 | NaDES-MAE1 | 120 °C, 30 min, in NaDES 1 (lactic acid/choline chloride (10:1)), MW (1500 W) | 14.5 ± 0.2 | 1:1.7 |
23 | NaDES-MAE2 | 120 °C, 30 min, in NaDES 2 (lactic acid/glycerol/choline chloride (1:1:1)), MW (1500 W) | 11.3 ± 0.1 | 1:1.2 |
a The solid recovered after filtration was post-treated with US (40 kHz, 200 W for 30 min at 50 °C) in the presence of an aqueous NaOH solution (1% of NaOH in dry biomass).
b The solid, recovered after filtration, was treated under conductive heating at 50 °C (for 30 min), in the presence of an aqueous NaOH solution (1% of NaOH in dry biomass).
Although it has been reported in the literature that maximum cavitation is achieved at 50 °C [58], lower temperatures were tested here to increase the sustainability of the process. Moreover, it is important to note that sonication time has the largest effect on biomass delignification. However, prolonged sonication beyond a certain limit has no additional effect in terms of delignification and after sugar release [59]. Besides duration, another parameter to directly determine the power of sonication is sonication frequency, and it is also important here as it affects lignocellulosic feedstock delignification. The majority of research in the field has used frequencies in the 10–100 kHz range, which is sufficient for cell rupture and polymer degradation [60]. NaDESs were exposed to US irradiation for longer time periods to overcome the problems related to their viscosity.
As regards MAE, the same GVL/H2O mixtures were tested under 30 min of irradiation at 90 °C. NaDESs were tested under MW irradiation for 30 min at 120 °C. Higher temperatures were required to reduce NaDES viscosity. In summary, longer reaction times at lower temperatures were used with US, whereas the MW-assisted treatments saw shorter reaction times and higher temperatures, owing to the specific features of the two technologies. To better understand the influence that the processes had on the chemical composition of the biomass, analytical pyrolysis (Py-GC/MS/FID) was applied to WS before and after treatment. The analytical data (Table 1) show that the lignin content in the residual solids decreased after extraction. In particular, it can be observed that the addition of 10% NaOH had a surprising negative effect on the GVL/water mixture-mediated US-assisted delignification (Table 1, entries 8, 9, 14, and 15). Meanwhile, temperature seems not to influence delignification when GVL content is higher (Table 1, entries 6 and 7). However, it does have an effect when the amounts of GVL and water are the same (Table 1, entries 12 and 13). Almost identical results were achieved by the two mixtures under MAE, indicating that the temperature may be the most important parameter to influence delignification in MW-assisted processes (Table 1, entries 16 and 18).
NaDESs are generally more promising extraction solvents than the GVL/H2O mixtures. Interestingly, NaDES 1 is more efficient under US irradiation (Table 1, entry 20), whereas NaDES 2 leads to higher delignification under MAE (Table 1, entry 23). This may be due to the synergism between two polar compounds enhancing the dielectric constant of the system and thus improving their interaction with MW.
Analytical pyrolysis data for US- and MW-pretreated WS in bio-based solvents demonstrated that the most prominent decrease in the lignin content was found in the MW method, whereas guaiacyl- and syringyl-type phenols (G units and S units, respectively) were found to be the dominant lignin-related analytic pyrolysis products in both untreated and pretreated biomass. The obtained data show the variations in relative S- and G-type phenol contents (S/G ratio) in the volatile products of analytical biomass pyrolysis (Table 1), indicating that the aromatic lignin structure was modified by the extraction process.
The clear differences in the influence exerted on the extractability of lignin from WS by the tested GVL/H2O mixtures, NaDES, and water treatments were also reflected in the color of the obtained extracts. The GVL and NaDES extracts exhibited the most intense color (Fig. 1), which relates to their better delignification activity.
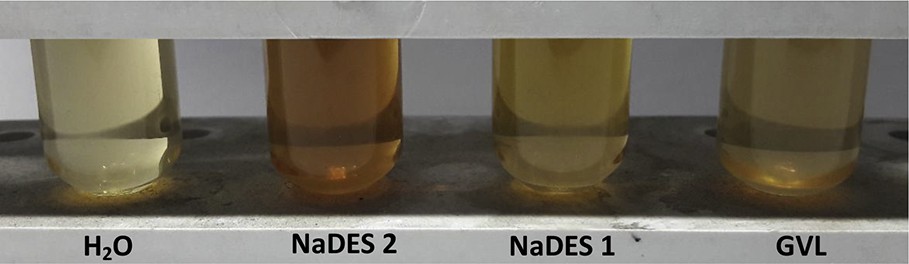
Delignification solvents.
Moreover, the content of levoglucosan (LG), in the analytical pyrolysis volatiles data, was lowered upon MW treatment in the presence of GVL. This becomes evident when compared with the data from the H2O solvent treatment (Fig. 2). This reveals that the proportion of ordered cellulose in the solid pretreated products decreased, making cellulose more prone to enzyme attack, which could be very interesting for the further valorization of WS by enzymatic digestion. On the other hand, LG content increased with NaDES 1 and NaDES 2, except for the NaDES-MAE2 sample, thus making NADES less useful for further enzymatic treatment (Fig. 3).
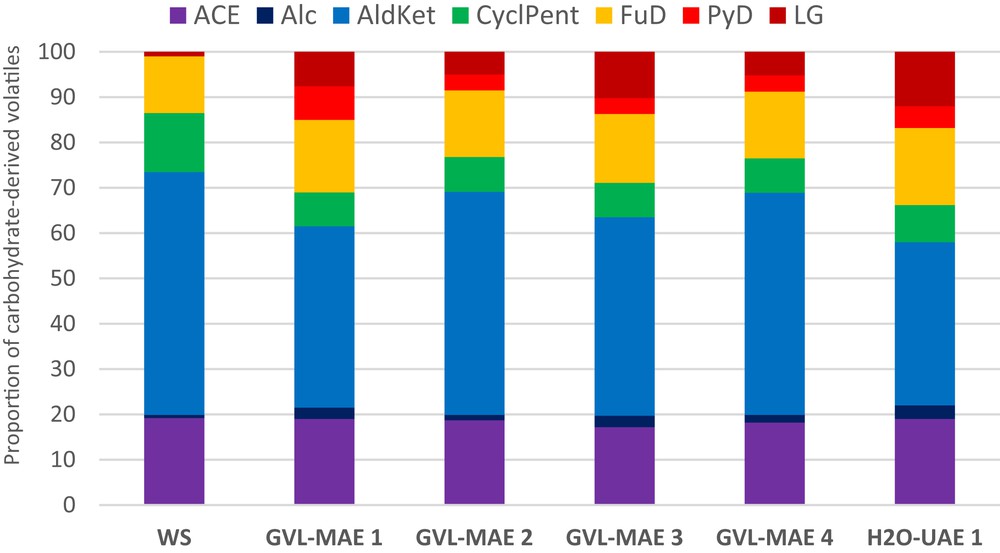
Aliphatic alcohol (Alc), aliphatic aldehydes and ketones (AldKet), cyclopentane derivatives (CyclPent), aliphatic acids and esters (ACE), furan derivatives (FuD), pyran derivatives (PyD), and LG (after normalization to 100%). Proportions of carbohydrate-derived products of the analytical pyrolysis of WS samples treated in the presence of GVL.
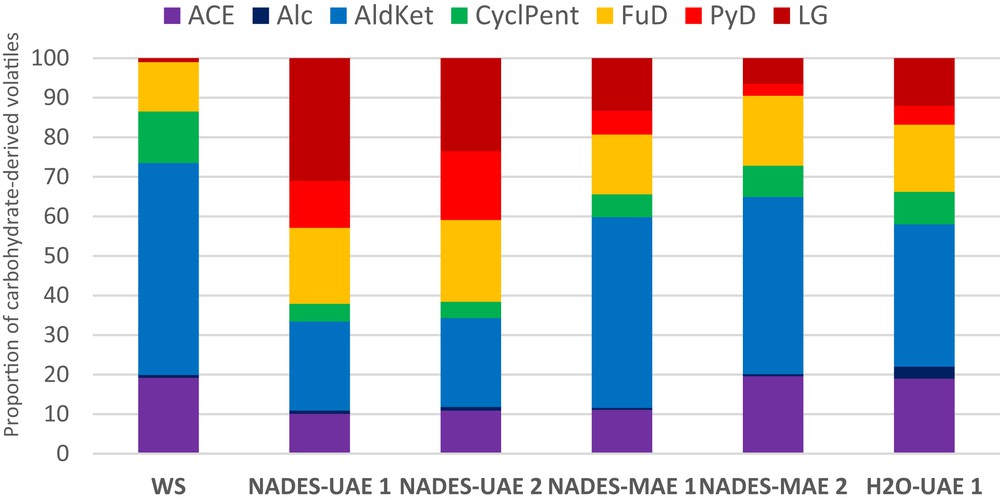
Aliphatic alcohol (Alc), aliphatic aldehydes and ketones (AldKet), cyclopentane derivatives (CyclPent), aliphatic acids and esters (ACE), furan derivatives (FuD), pyran derivatives (PyD), and LG (after normalization to 100%). Proportions of carbohydrate-derived products of the analytical pyrolysis of WS samples treated in the presence of NaDES.
The radical scavenging capacity (by DPPH test, Table 2) and TPC (by Folin–Ciocalteu colorimetric method, Table 3) of the crude US and MW extracts were also evaluated. The samples that provided the best delignification data, according to the results reported in Table 1, were analyzed.
Antioxidant activity of US and MW extracts.
Entry | Sample | Extraction method | DPPH results IC50 | |
1 | NaOH-UAE2 | 10% NaOH | US | 0.2451 (0.2131–0.2917) |
2 | NaOH-MW2 | 10% NaOH | MW | 0.2863 (0.2383–0.3514) |
3 | GVL-UAE8 | GVL/H2O | US | 0.3129 (0.2604–0.3742) |
4 | GVL-MAE1 | GVL/H2O | MW | 0.3617 (0.3117–0.4235) |
5 | NaDES-UAE1 | NaDES 1 | US | 0.2713 (0.2493–0.3063) |
6 | NaDES-MAE1 | NaDES 1 | MW | 0.3357 (0.3061–0.3717) |
7 | NaDES-UAE2 | NaDES 2 | US | 0.2619 (0.1235–0.4461) |
8 | NaDES-MAE2 | NaDES 2 | MW | 0.3652 (0.3244–0.4163) |
TPC of the liquid fractions obtained from WS delignification in the presence of green solvents.
Entry | Sample | WS pretreatment | TPC (mg of gallic acid equivalents per 100 mL) |
1 | GVL-UAE1 | 35 °C, 60 min, in 8:2 GVL/H2O, US (40 kHz, 200 W) | 10 ± 1 |
2 | GVL-UAE2 | 50 °C, 60 min, in 8:2 GVL/H2O, US (40 kHz, 200 W) | 17 ± 2 |
3 | GVL-UAE3 | 35 °C, 60 min, in 8:2 GVL/H2O + 10% NaOH, US (40 kHz, 200 W) | 14 ± 1 |
4 | GVL-UAE4 | 50 °C, 60 min, in 8:2 GVL/H2O + 10% NaOH, US (40 kHz, 200 W) | 14 ± 1 |
5 | GVL-UAE7 | 35 °C, 60 min, in 1:1 GVL/H2O, US (40 kHz, 200 W) | 21 ± 2 |
6 | GVL-UAE8 | 50 °C, 60 min, in 1:1 GVL/H2O, US (40 kHz, 200 W) | 24 ± 1 |
7 | GVL-UAE9 | 35 °C, 60 min, in 1:1 GVL/H2O + 10% NaOH, US (40 kHz, 200 W) | 21 ± 1 |
8 | GVL-UAE10 | 50 °C, 60 min, in 1:1 GVL/H2O + 10% NaOH, US (40 kHz, 200 W) | 25 ± 1 |
9 | GVL-MAE1 | 90 °C, 30 min, in 1:1 GVL/H2O, MW (1500 W) | 29 ± 1 |
10 | GVL-MAE2 | 90 °C, 30 min, in 1:1 GVL/H2O, MW (1500 W)a | 25 ± 1 |
11 | GVL-MAE3 | 90 °C, 30 min, in 8:2 GVL/H2O, MW (1500 W) | 17 ± 2 |
12 | GVL-MAE4 | 90 °C, 30 min, in 8:2 GVL/H2O, MW (1500 W)a | 24 ± 2 |
a Samples for which post-treatment was performed as reported in the Section 2.3.
Lower IC50 values indicate higher radical scavenging activity, as the IC50 indicates the concentration of the tested antioxidant samples required for a 50% inhibition of the radical species [21]. Despite MAE showing the best results in terms of delignification, antioxidant activity was higher in the extracts obtained under US treatment. This is probably due to the higher lignin depolymerization induced by cavitation. The MW-extracted lignin most likely still displayed a high polymerization degree, owing to the reduced reaction time. Once more, NaDES proved to be more efficient than GVL/H2O mixtures, with the three-component NaDES 2 showing the best results. The higher extractability that phenolic compounds show in NaDESs may be attributed to H-bonding interactions that occur between the NaDES molecules and phenolic compounds. The functional groups that are generally involved in H-bonds are hydroxyl, carboxylic, and amine groups, all of which are abundant in NaDESs, whereas hydroxyl groups are obviously available in phenolic compounds. Furthermore, it can be observed that the alkaline extracts showed the best antioxidant activity.
The data on TPC in the liquid fractions obtained after the WS US and MW extraction processes (Table 3) did not show high values.
It should be noted that it was not possible to carry out this analysis on the samples obtained using NaDES 1 and NaDES 2 because of the stratification of the end products of the Folin reaction, meaning that their phenolic content could not be detected by UV–vis spectrophotometry.
Finally, the surface characteristics of the recovered US and MW pretreated WS samples were investigated. The results of the nitrogen gas adsorption–desorption experiments are summarized in Table 4.
The surface characteristics of WS biomass before and after US and MW extraction processes, calculated from the results of a nitrogen gas adsorption–desorption experiment.
Entry | Sample | Surface characteristics | ||||
Specific surface area (SBET, m2/g) | Total pore volume (mm3/g) | Micropore volume (mm3/g) | Average pore width (nm) | |||
DR | BJH | |||||
1 | WS | 1.3 ± 0.2 | 5.1 ± 0.7 | 0.35 ± 0.05 | 3.7 ± 0.7 | 3.7 ± 0.4 |
2 | H2O-UAE1 | 2.4 ± 0.3 | 11.1 ± 2.0 | 0.80 ± 0.03 | 3.5 ± 0.1 | 3.5 ± 0.1 |
3 | H2O-UAE2 | 2.3 ± 0.1 | 9.8 ± 0.2 | 0.78 ± 0.05 | 3.6 ± 0.1 | 3.3 ± 0.2 |
4 | GVL-UAE1 | 1.3 ± 0.2 | 4.7 ± 0.1 | 0.43 ± 0.03 | 3.2 ± 0.1 | 3.4 ± 0.1 |
5 | GVL-UAE2 | 1.1 ± 0.1 | 4.3 ± 0.1 | 0.31 ± 0.02 | 3.2 ± 0.1 | 3.3 ± 0.2 |
6 | GVL-UAE3 | 1.6 ± 0.1 | 5.1 ± 0.1 | 0.61 ± 0.04 | 3.2 ± 0.1 | 3.5 ± 0.5 |
7 | GVL-UAE4 | 2.0 ± 0.1 | 7.0 ± 0.4 | 0.74 ± 0.02 | 3.1 ± 0.1 | 3.3 ± 0.2 |
8 | GVL-UAE5 | 2.7 ± 0.1 | 8.2 ± 0.1 | 0.84 ± 0.01 | 3.2 ± 0.1 | 4.1 ± 0.4 |
9 | GVL-UAE6 | 2.7 ± 0.3 | 9.2 ± 2.0 | 0.88 ± 0.08 | 3.2 ± 0.1 | 3.7 ± 0.3 |
10 | GVL-UAE7 | 3.1 ± 0.1 | 9.6 ± 0.6 | 1.22 ± 0.01 | 2.7 ± 0.4 | 3.9 ± 0.2 |
11 | GVL-UAE8 | 2.0 ± 0.1 | 9.0 ± 0.6 | 0.82 ± 0.01 | 2.9 ± 0.1 | 3.1 ± 0.2 |
12 | GVL-UAE9 | 3.3 ± 0.1 | 10.2 ± 1.4 | 1.14 ± 0.01 | 2.9 ± 0.1 | 3.9 ± 0.1 |
13 | GVL-UAE10 | 2.0 ± 0.1 | 7.5 ± 0.1 | 0.86 ± 0.03 | 3.0 ± 0.1 | 3.9 ± 0.1 |
14 | GVL-MAE1 | 1.6 ± 0.2 | 5.8 ± 0.4 | 0.45 ± 0.04 | 3.5 ± 0.2 | 3.1 ± 0.1 |
15 | GVL-MAE2 | 1.2 ± 0.2 | 7.0 ± 0.4 | 0.38 ± 0.04 | 3.4 ± 0.2 | 3.9 ± 0.2 |
16 | GVL-MAE3 | 1.8 ± 0.2 | 6.3 ± 0.4 | 0.52 ± 0.04 | 3.7 ± 0.3 | 3.4 ± 0.1 |
17 | GVL-MAE4 | 1.6 ± 0.2 | 6.2 ± 0.6 | 0.49 ± 0.03 | 3.2 ± 0.2 | 3.5 ± 0.3 |
18 | NaDES-UAE1 | 2.0 ± 0.2 | 10.0 ± 1.6 | 0.54 ± 0.04 | – | 4.3 ± 0.4 |
19 | NaDES-UAE2 | 1.1 ± 0.2 | 8.4 ± 0.8 | 0.32 ± 0.04 | 3.7 ± 0.1 | 3.1 ± 0.2 |
20 | NaDES-MAE1 | – | – | – | – | – |
21 | NaDES-MAE2 | – | – | – | – | – |
US lignin extraction in the presence of bio-based solvents generally increased WS porosity both in the GVL (entries 6–9 and 10–13) and NaDES samples (entry 18). Moreover, the addition of NaOH (10% in dry biomass) positively influenced the surface parameters of recovered WS (entries 5 and 7, Table 4). A further improvement in the surface characteristics of the GVL/H2O–treated WS (more than obtained by adding NaOH to bio-based solvents) was recorded when the solid was further sonicated in the presence of NaOH (1% on dry material) as can be seen by the results for entry 5 versus 7 and 9 (Table 4). This result was probably due to the degrafting of GVL from the WS surface, as mediated by NaOH. NaDES 1 was more effective than NaDES 2 in improving the surface characteristics of US-pretreated biomass (Table 4, entry 18 vs 19).
MW pretreatment of WS biomass in green solvents (Table 4, entries 14–17) did not significantly influence WS porosity.
4 Conclusions
In comparison with conventional extraction, the proposed MAE and UAE with green solvents present significant advantages in terms of sustainability. These findings suggest that US and MW are ecofriendly techniques suitable for WS delignification.
A mixture of natural compounds, NaDES and bioderived GVL, has been tested as solvents for the extraction of lignin-derived compounds as an alternative to alkali treatment.
The highest delignification (45%) for unconventionally pretreated WS in the presence of bioderived solvents was reached under MW irradiation at 120 °C in only 30 min in the presence of three-component NaDES. A GVL/water mixture also gave efficient delignification (27%) under US mild pretreatment (50 °C for 60 min). Antioxidant activity, measured using the DPPH test, was higher in the extracts obtained under US irradiation, and in particular, using NaDES. This holds promise for further applications of NaDES in the valorization of lignin as an antioxidant additive in pharmaceuticals, cosmetics, and the food industries. Furthermore, GVL/water mixtures proved efficient in decreasing the portion of ordered cellulose in WS. According to these findings, NADESs could be used for liquid fraction valorization, whereas GVL could be the solvent of choice for further solid applications. These nonconventional treatments can lead to the valorization of both the lignin and the cellulose in WS and, as such, fulfill the requirements the biorefinery concept.
Acknowledgments
The H2020 Project US4GREENCHEM (Grant Agreement no. 669055) “Combined ultrasonic and enzyme treatment of lignocellulosic feedstock as substrate for sugar based biotechnological applications” is warmly acknowledged for the funds provided by the BBI-JU.