1 Introduction
In their 12 Principles of Green Chemistry, Anastas and Warner [1] explicitly claim the use of “safer solvents and auxiliaries”. And who would contradict this claim? Consequently, there are an uncountable number of scientific articles dealing with “green solvents”. The question is, how much will remain from all these academic efforts? Will there be a chance for all these new solvents to come into the market, if only for some speciality applications? In this context, we would like to make some comments on the ongoing research and future directions of green solvents.
2 No-solvent systems
The best solvent is sometimes no solvent. In full agreement with the fifth Principle of Green Chemistry [1], it has been shown that there are relevant chemical and industrial processes, in which solvents can be completely avoided. Of course, this is not always possible. If the lack of the solvent causes dangerous overheating or leads to a higher energy demand, these disadvantages would foil the advantage of a solvent-free process.
For many years, reasonable effort has been made on the preparation of polymers in the absence of conventional reaction solvents. Indeed, there are industrial polymerization processes avoiding the use of solvents, like melt polymerization or solid state polymerization. For example, PET is commercially produced by melt polymerization followed by a solid state polymerization process [2–4]. It is even possible to prepare polymers (e.g., poly(phenylene vinylene)) by ensuring close contact of the reacting molecules in a ball milling process within minutes [5]. The ball milling method is not only known in polymer science but also in organic synthesis. Besides avoiding the use of organic solvents, it exhibits further advantages like high energy efficiency and reduced reaction times [6]. Solvent-free microwave extraction is another elegant technique worth to mention. Developed in 2004 by Lucchesi et al. [7,8], it is used for the extraction of essential oils from fresh plant materials in a microwave-assisted dry distillation process. It is a rapid method that delivers a powerful alternative to the conventional water-consuming and long-lasting hydrodistillation.
3 Water as a solvent
Some scientists try to replace organic solvents by water, as it bears several advantages, like a low hazard potential, high availability and low cost. It also exhibits interesting aspects concerning reactivity: uncommon selectivities, influences of hydrogen-bond network on reaction behaviour, adjustable pH values, use of salts for the salting-in or salting-out effect and the application of biphasic reaction systems. Hence, it is certainly a valuable approach, but it should be kept in mind that water has also some disadvantages: its temperature-restricted range of liquid state and especially its significant heat capacity, which makes distillation processes extremely energy consuming. The same is true for pervaporation and reverse osmosis. All these techniques may be necessary when water must be separated from a non-volatile reaction product or impurities. In these cases, the high energy consumption may lead to the choice of another solvent.
Nevertheless, many promising results have been published over the years following this approach [9–11]. For example, the Diels–Alder reaction in water has been widely studied since 1980, when Rideout and Breslow [12] reported a certain rate acceleration using water as reaction medium. In all those studies on organic reactions in water, water is used both under atmospheric pressure and under higher pressures. In the latter case, reaction conditions with elevated temperatures are often adopted to reach the so-called subcritical water region, which is in general the liquid water phase above the boiling point at ambient pressure (100 °C) and below the critical point (374 °C, 22.1 MPa). Physical and chemical properties of subcritical water show a significant dependence on temperature and pressure, so that solvent properties are strongly influenced and adjustable. By keeping the pressure rather low, glass containers with decently thick walls could even still be used. Subcritical water can be considered as a promising solvent for biomass processing, for example, the conversion of lignin-derived compounds into value-added fuel products with and without addition of industrial gases [13–15]. In addition, various organic reactions could be successfully performed under “hydrothermal” conditions, that is, in water at temperatures between 150 and 250 °C [16,17].
4 Classical green solvents
Replacing conventionally used solvents by green solvents is a very wide field of research and development. A first general requirement is to define what a green solvent is. Gu and Jérôme [18] proposed 12 criteria that green solvents should fulfil related to availability, price, recyclability, grade, synthesis, toxicity, biodegradability, performance, stability, flammability, storage and renewability. Doubtlessly, this is a reasonable collection of conditions. However, it also demonstrates the dilemma of classifying a solvent as green, as in most of the cases not all requested parameters can be fulfilled by one solvent. What if the solvent in hand only complies with 11, 10 or 9 out of the required 12 characteristics of green solvents? Can it still be considered as green?
As a result, we should be precise about the meaning of a green solvent. It is clear that it is a relative term. Even Anastas and Warner [1] defined green chemistry as a reduction of harm and not as processes that do not cause any harm. Consequently, a new and apparently benign solvent can be termed green when it is “greener” as compared to the conventionally used solvent that should be replaced and when clear evidence can be given for this assumption.
In nearly all cases, the ultimate choice will be a compromise between several requirements, as it is schematically resumed in Fig. 1:
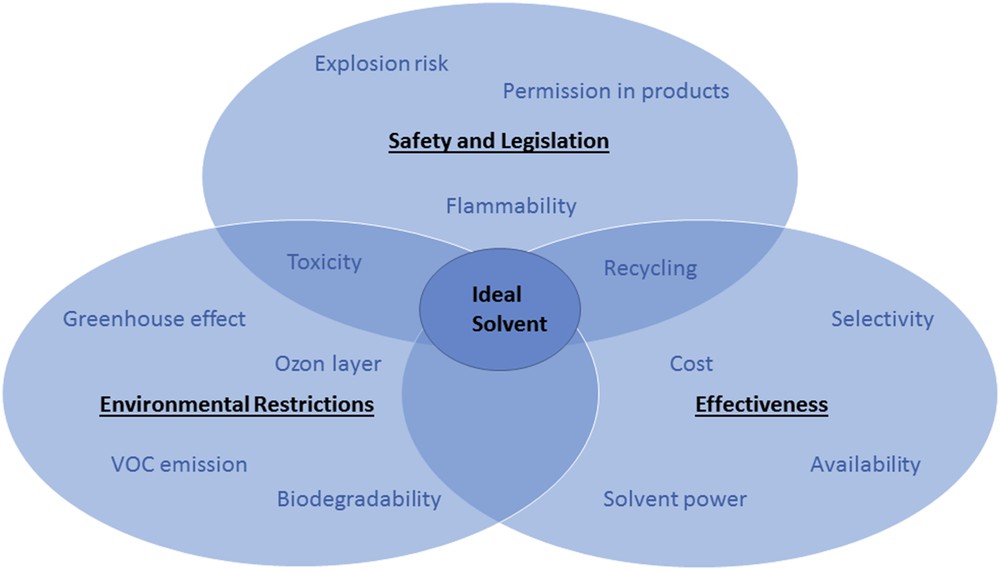
The ideal solvent is always a compromise between a multitude of different requirements (adopted with permission from Chemat [19]).
As recently proposed by Jessop [20], the best way to estimate the environmental impact of a solvent is a life cycle assessment (LCA), as it is done for many other chemical substances. It considers the whole fate of a solvent from the manufacturing until its disposal or recycling. Such an LCA must be done for each specific application of the solvent. It may turn out that a solvent is green (meaning less harmful than another one) for one process, but not for another process. For example, and as it was discussed in Section 3, water may be a green solvent, but whenever its distillation or other ways of purification are required, this can be very energy-consuming and changes the greenness of water for a given process.
During the last years, an almost uncountable number of articles and several excellent reviews and monographs have been published. They provide a very useful overview of a large variety of solvents and their classification as more or less green solvents [18,20–30]. It would be beyond the scope of the present article to go into details; the interested reader is referred to the literature. Still, we would like to emphasize on some particular points. Although we will not present LCAs, some interesting aspects will be mentioned. As a consequence, the picture will necessarily not be complete.
Talking about classical green solvents, we are thinking of already existing solvents that are Registration, Evaluation, Authorisation and Restriction of Chemicals (REACH) registered and may already find some applications in chemical industry and formulation. There are several issues we would like to mention regarding this kind of solvents:
- (1) The biogenic origin of a solvent is neither a sufficient nor even a good criterion of the greenness of a solvent. For example, the bio-based furfural is considered as a renewable and sustainable platform chemical for the production of numerous value-added chemical compounds [31,32]. However, it is classified as toxic and carcinogenic. On the other hand, there is a class of petrol-based solvents that are eco-friendly showing a good human safety environment profile, for example, 3-methoxy-3-methyl-butane-1-ol [28,33].
- (2) It is worth defining the sectors of application, in which green solvents are most urgently needed. According to Jessop [24], these are “solvents of high basicity/low polarity, high basicity/high polarity and low basicity/medium-to-high polarity. In other words, we lack replacements for amines, HMPA, halogenated solvents and aromatic solvents”. Especially for these classes of solvents, it is difficult to find greener substitutes (please note that this requirement is not only valid for the classical green solvents).
A particular problem is the use of hexane, which currently is the solvent of choice for the extraction of plant, fruit and vegetable oils. This solvent presents serious health concerns and is threatened to be banned in Europe for this application in the next years. There is still a controversy on if this will indeed happen, as long as no adequate substitute is found. Certainly, some alternatives exist such as extraction with supercritical CO2 [34] or with 2-methyltetrahydrofuran (MeTHF) [35], but of course, these solvents have other shortcomings (e.g., an ether-like MeTHF may be explosive and requires special safety measures) and can never replace hexane 1:1.
- (3) There are some solvents that are known for a long time and that may have a promising future in the context of green solvents. For example, γ-valerolactone has a low melting and high boiling and flash point, it has a pleasant herbal odour and as such is used in flavour and perfume industries and even as food additive. It is found in fruits, it is of very low toxicity and miscible with water, and it is stable under neutral conditions. Also most important is that it has excellent solvent properties and may, for example, be used in cleaning products [36,37]. Of course, γ-valerolactone is also energy demanding when it must be distilled, but for many applications, for example, in cleaning agent formulations, this is irrelevant.
Other promising candidates of green solvents are not only glycerol derivatives, such as glycerol carbonate, solketal or triacetin [38–41], but also lactic acid and its derivatives (e.g., ethyl lactate) [42,43]. The dimethyl amide of lactic acid is sold by BASF as a green and safe alternative to N-methyl pyrrolidone.
5 Novel green solvents
5.1 Ionic liquids
In the context of green solvents, we would like to comment on ionic liquids (ILs) that, at least for a certain time, have been considered as not only designer solvents but also as green solvents, mainly because they have a negligible vapour pressure and as such do not contribute to the volatile organic compounds' problematic. But of course, often their synthesis is not green at all, many of them are badly biodegradable and show a significant toxicity [44–46]. The dilemma is that the term ionic liquids comprises a whole bunch of chemically very different solvent and liquid classes with extremely different characteristics, except that they are all salts and liquid at low to moderate temperature. Similar to the term green solvents, ILs are so “species-rich” that the term becomes almost meaningless.
Let us consider some examples. The most widespread class of ILs is probably the one containing N,N′-dialkyl-substituted imidazolium cations combined with a convenient counterion. As Jessop [24] pointed out, the synthesis of these cations requires a significant amount of chemical reaction steps, also involving non-green reactions. Although at least a few of them have an acceptable toxicity, there is no enzyme known that could cleave the NC-bonds. Consequently, these cations are not biodegradable [45] and therefore not in agreement with one of the major requirements of a green solvent.
In fact, there are two classes of ILs that can be considered to be at least somewhat greener—depending, of course, on their application—than the usual ones considered in most publications.
The first one comprises ILs consisting of cations that are found in the metabolism of living things or at least derived from them. Such cations can be based on choline, betaine, carnitine, or amino acids [47–50]. Especially choline seems to be promising, as it is cheap and available in large quantities (although it has to be admitted that usually it is not extracted from biomass, but rather chemically synthesized). Choline hexanoate, for example, can be obtained by simply reacting choline hydroxide with hexanoic acid in a one-step synthesis. It was shown that it has remarkable features, such as the ability to dissolve suberin, a natural polyester and the main component of cork [51]. As a consequence, ILs like choline hexanoate can be regarded as potentially green ILs.
The second class includes ILs, which contain ethylene oxide (EO) groups. In contrast to other ILs, where crystallization is energetically hindered by the bulky cations, their low melting temperature is the consequence of high entropy contributions caused by introducing several EO groups (usually in the anion). Due to these structural features, these ions show high flexibility and thus favour the liquid state. A typical IL representative of this class is the sodium 2,5,8,11-tetraoxatridecan-13-oate ([Na][TOTO]) [52], which is shown in Fig. 2. These TOTO compounds are already commercialized by Kao Chemicals under the trade name Akypo. They are cheap, available in large quantities, of low toxicity and, in contrast to choline ILs, even admitted in cosmetic products. In addition, they convince with high thermal and electrochemical stability. However, it has to be noted that for their synthesis, although it is cheap and simple, the use of “non-green” reactants cannot be avoided.
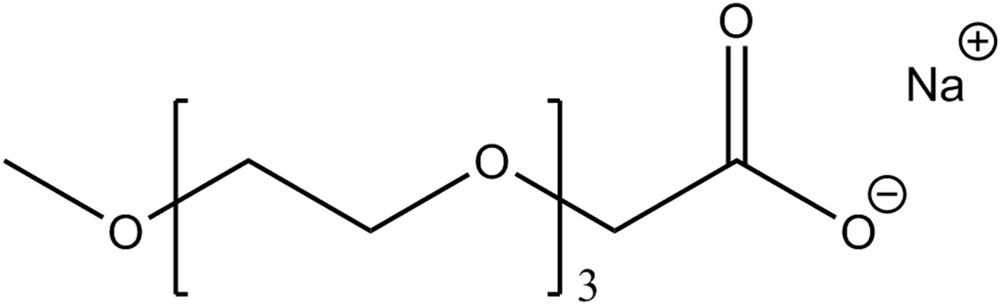
[Na][TOTO] as the example of an easy-to-make low toxic IL.
In the context of TOTO ILs, it is interesting that this chain flexibility caused by EO groups is a well-known feature in surfactant science. In fact, there are many non-ionic surfactants and so-called extended surfactants (surfactants comprising a long alkyl chain, several EO or propylene oxide (PO) groups and a charged head group) that are liquid at room temperature and, in the case of extended surfactants, could be also considered as ILs. However, the focus of studying these substances is usually set on classical surfactant behaviour in aqueous solutions rather than on their IL properties.
It is a pity that in nearly all publications about the alleged greenness of ILs, there is hardly any comparison to the (more or less green) properties of classical solvents—a gratifying exception is Ref. [53], in which indeed such comparisons are reported. We would like to see tables of the 12 criteria of green solvents clearly presenting point by point such comparison between the newly presented solvent and the conventional one, which it should replace. Nevertheless, in rather rare cases, there are also other advantages, like solvent performance, as compared to an existing solvent. In this case, of course, it will be essential to explain in detail the performance enhancement that is achieved by application of an IL, as compared to the usual solvent. Comparing all properties of a novel and a conventional solvent is in fact highly required as a standard procedure in the whole field of green solvent research. In other words, a complete LCA is mandatory.
One should also be aware of several other disadvantages of ILs, such as relatively high price, especially for reasonably pure ILs, their often high to very high viscosity and the high effort required for their synthesis.
For a more detailed discussion of ILs, see our recent mini-review “The hype with ionic liquids” [54].
5.2 Ephemeral solvents
If a solvent cannot be avoided or if the price to pay would be too high, there is a way to create an “ephemeral” solvent only during the reaction. One strategy is to take profit of the current knowledge on deep eutectic solvents (DESs). The corresponding solvents are gained by combining an organic salt with a hydrogen bond donor and simply mixing them at elevated temperature until a homogeneous liquid forms through extreme melting point depression. Although such mixtures have been described already in the 1990s, today's most well-known solvent of this kind was reported by Abbott et al. in 2003 [55], when he studied mixtures of choline chloride and urea (for a review see Ref. [30]). DESs exhibit properties similar to ILs. However, where ILs often require a complicated synthesis, the formation of DESs is very simple and inexpensive. As the toxicity and biocompatibility of these solvents are thought to be dependent on the individual components, it is relatively simple to obtain eco-friendly solvents [56–58].
If one of the reagents of an organic reaction is, for example, an acid, it can occur that it is liquefied by adding a salt such as choline chloride. The second reactant might then be well soluble in this liquid mixture and the reaction can start. At the end, by simply adding water, the salt can be separated from the hydrophobic water-insoluble product. This approach has been applied to synthesize caffeic acid phenethyl ester (CAPE), which is a molecule of pharmaceutical relevance [59]. Fig. 3 illustrates the approach: as caffeic acid is almost insoluble in the second reactant phenethyl alcohol, a DES with choline chloride and caffeic acid (molar ratio 2:1) is built first, in which phenethyl alcohol is completely soluble. The reaction is started by the addition of an acid catalyst. When the reaction is complete, the reaction mixture is poured into hot water, where choline chloride and residual phenethyl alcohol solubilizes. The CAPE product precipitates upon cooling and can be collected by filtration.

Acid-catalyzed esterification of caffeic acid with phenethyl alcohol and subsequent collection of CAPE by the addition of water.
5.3 Switchable solvents
In 2010, Jessop et al. [60] introduced the concept of “switchable solvents”. They could show that depending on the presence or absence of CO2, certain species can either be water soluble or play the role of a hydrophobic solvent, respectively. This phenomenon can be observed in water–amidine systems.
Because the mixing–demixing process (see Fig. 4) is reversible and can be tuned simply by controlling air and CO2 pressure, a hydrophobic water-immiscible phase can be temporarily created and removed, when the desired chemical reaction in the organic phase is complete. The non-polar organic product can be collected by separating it from the hydrophilic mixed state of the solvent system. Tremendous advantages of these switchable solvents are the uncomplicated isolation of the product and the simple recovery of the organic solvent from water by removing carbonate from the mixture. In this way, an energy-consuming distillation can be completely avoided.

Change in the phase behaviour of amidines in the presence of CO2 (adopted from Jessop et al. [60]).
On the other hand, the used chemicals such as amines, amides and amidines are not always green. Many of them fail to fulfil the desired properties of green solvents, whereas others are acceptable. For a critical discussion of this point see Ref. [21]. Nevertheless, this is a clever system and might be helpful in further development of green solvents and solvent systems.
Instead of using CO2 as a trigger, temperature can be used for this purpose, as shown by Khono et al. [61] in water/IL mixtures. Another example is given in Fig. 5.
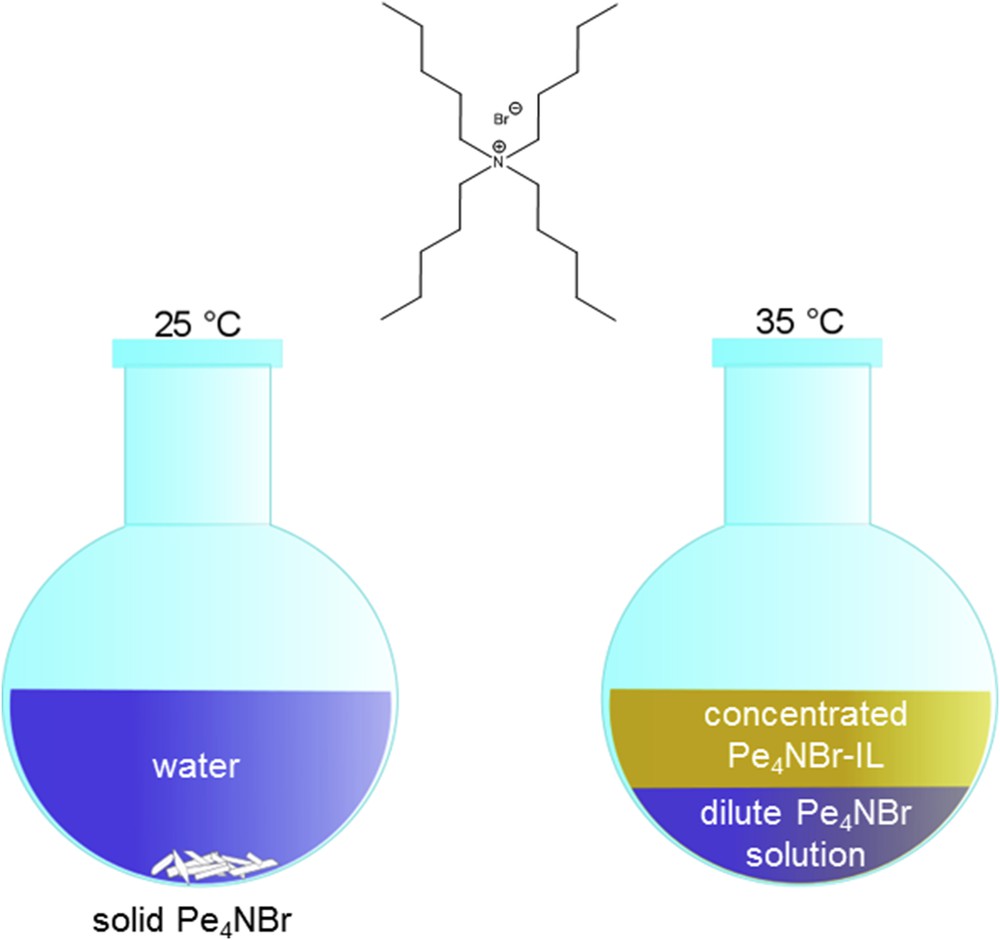
Change in the phase behaviour of Pe4NBr in water upon changing the temperature.
Tetrapentylammonium bromide (Pe4NBr) is nearly insoluble in water at room temperature. With a melting point close to 100 °C, it is at the limit of what is usually called IL. But what matters here is its remarkable phase behaviour when mixed with water. Above approximately 30 °C, it becomes highly water soluble, but at the same time, and as otherwise only known from polymer solutions, it separates into two phases, building a water-rich and a second water-poor phase in equilibrium. The latter behaves like an organic phase. As a result, organic molecules can be dissolved in this phase during a chemical reaction. At the end, the hydrophobic phase can easily be recycled simply by decreasing the temperature, because then the phase vanishes and the salt precipitates. As such, it even does not pollute the water phase. A liquid organic product phase separates from the aqueous phase and can easily be isolated. As in the case of Jessop's switchable solvents, a distillation process can be avoided. However, one disadvantage should be mentioned: the salt contains bromide, which is not always desirable in industrial processes.
This approach of a triggered pathway from a one-phase to a two-phase system is only one example of a whole family of similar processes. For example, Bergbreiter et al. [63] took profit of a monophasic solvent–polymer mixture close to its immiscibility so that the phase separation after the reaction could be easily induced by adding a small amount of additional solvent. Ludmer et al. [66] developed what they called “sediments remediation phase transition extraction” coupled with a phase transition cycle induced by temperature variation. This process involves a ternary mixture of the environmentally safe solvents, water, ethanol and ethyl acetate, and has been successfully applied to depollute heavily contaminated sludge from a wastewater treatment plan.
5.4 Natural DESs
In the case of the ephemeral solvent, as discussed above, the concept of DESs has been applied. Solvents are called natural DESs (NADESs) when both components (or even more in the case of multicomponent low melting mixtures (LMMs)) are “natural” or of “biological origin”. However, these terms are somewhat misleading. It is true that the components of NADESs occur in living things, but in many cases they are chemically synthesized, for example, choline, see Section 5.1. It should be kept in mind that petrol and hence its components are also of natural origin, but they are not considered to be sustainable.
Anyway, it was discovered in the last years that such NADESs or natural LMMs may play a significant role as solubilizers of hydrophobic molecules in biological systems and especially in plants [30,62]. Because in the present special volume, there will be other articles especially devoted to this type of solvents, we will not consider them in detail here. Instead, we would like to focus on one aspect: although there is no doubt that DESs and LMMs are liquid at low temperature—sometimes even significantly below room temperature—they are often mixed with a significant amount of water [64,65]. In that case, they should better be called hydrotropes as they facilitate the solubility of organic compounds in these rather aqueous solutions.
A typical mixture is malic acid–choline chloride–water in a molar ratio of 1:1:2. The binary mixture of the first components shows already a significant depression in melting point as compared to the individual components. The third component, water, can rather be seen as a cosolvent that reduces viscosity and the consolidation of the three compounds might rather resemble a solubilization of choline chloride and malic acid in water. So it is questionable, if the term “natural deep eutectic solvent” is appropriate for a “real” deep eutectic solvent that is mixed with/solubilized in water. For further information, an article of Hammond et al. [67] studying the nanostructure of the ternary malic acid–choline chloride–water system can be recommended.
Of course, what is stated here is only a linguistic issue and in fact such ternary mixtures of natural products with water are most valuable and useful, for example, for plant extraction processes. We believe that we are only at the beginning of an application era of these systems and suggest the term “natural hydrotropic mixtures” (NAHYMs) for future discussions.
Finally, another aspect should be mentioned. It would be challenging to directly extract true NAHYMs from plants and to see their solubilization power. For example, the essential oil of Nanah mint is very hydrophobic and insoluble in (cold and warm) water. In contrast, putting the oil containing fresh leaves in hot water gives a wonderful aromatic tea. Obviously, other components (NAHYMs) in the leaves ensure a sufficient solubility of the Nanah essential oil in aqueous solution.
5.5 Hydrotropes
In the previous section, we have already mentioned hydrotropes. This term was coined by Neuberg [68] and denotes amphiphilic compounds that increase the solubility of sparingly soluble organic substances in water. For more detailed information the reader is referred to a review article [69].
In our opinion, there is an increasing demand of natural or at least non-toxic biodegradable hydrotropes. As an alternative to conventional solvents, it can be useful to consider a hydrotropic system as an at least ternary macroscopically homogeneous mixture of water, the hydrotrope (or a mixture of them as in the case of NADESs) and a hydrophobic compound, and this at any compositions.
From Fig. 6 [69], it can be observed that the solubility of the organic compound S can be most relevant in several compositions of the system: region 1 features a rather dilute aqueous solution with classical hydrotrope action; region 4 would resemble a system like the above-mentioned NADES malic acid–choline chloride–water (1:1:2) with a comparably low water content; region 2 shows a mainly reverse system, where the organic solute might be liquefied by the presence of water and the hydrotrope; region 3—often referred to as pre-Ouzo region—is a special case, where the significant mesoscopic structuring of the macroscopically homogeneous mixture can be most relevant for solubilization and reaction processes [70,71].
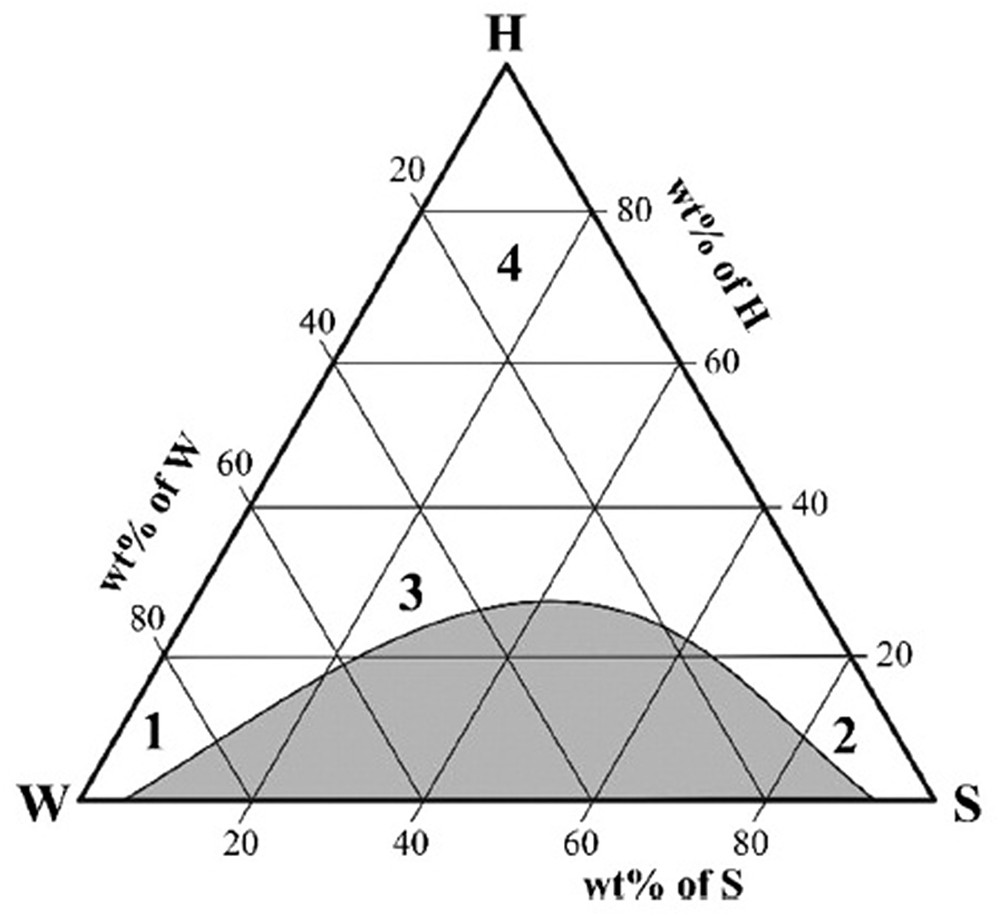
Typical ternary phase diagram of water (W)–organic solvent (S)–hydrotrope (H) (taken from Kunz et al. [69]).
As a concrete example of such a hydrotropic bio-based ternary system, we cite the mixture of water with ethanol and ethyl acetate [72]. Its phase diagram is shown in Fig. 7. It is a green alternative to the classical system water–methanol–chloroform, which is widely used, for example, to extract and then purify lipids from microorganisms. At the composition indicated with the blue dot, the microorganisms (or plant materials) are subjected to an extraction process. By adding a water–ethyl acetate mixture (following the blue arrow) after the extraction is complete, the solution phase separates and the lipids can be selectively recovered in the ethyl acetate–rich phase at the end of the tie line (indicated in black). Both extraction and subsequent separation only imply environmentally acceptable solvents.
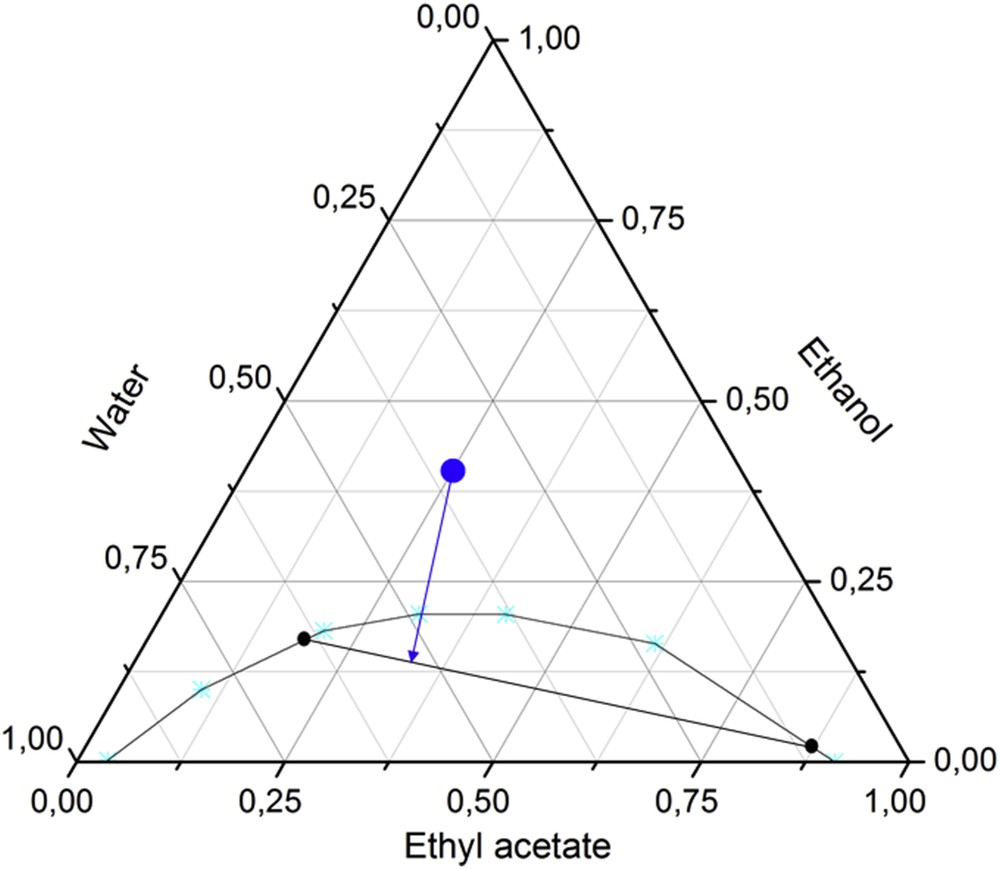
Ternary phase diagram of a water–ethyl acetate–ethanol system (adopted from Breil et al. [72] and complemented for the purpose of this review).
6 Conclusion and outlook
Considering today's incredibly huge amount of information on solvents in general and “green” solvents in particular, it is not easy to draw a general conclusion. Too many parameters and perspectives have to be considered. However, we would like to focus on some crucial points:
- (1) A solvent is only green, when it is better suited than the present one for a particular application. Better means, for example, better fulfilling the criteria proposed by Gu and Jérôme [18]. And of course, it is necessary to also consider its competitiveness in price and performance. There is no absolutely green solvent. It always depends on the context of its application and it is only a relative term. Although this has been known for a long time, it is often forgotten today. The best way to study the greenness of a solvent is through an LCA, as it is done for other chemicals and processes.
- (2) It is good to develop green alternatives for currently applied solvents. But it is even better to think about new concepts. One of them could be the development of ephemeral [59] and switchable [60] solvents that are created only during a desired process. When used as reaction medium, these are smart systems for an easy separation of the reaction product.
- (3) Ternary mixtures of environmentally benign solvents such as water–ethanol–ethyl acetate have a significant potential for extraction and purification processes. Two examples are shown in this article. The key advantage is that a slight change in temperature can induce a reversible phase separation.
- (4) We need not only green solvents, but also green cosolvents or solubilizers to make hydrophobic molecules water soluble. There remains a considerable work to be done in this field, as most of the known hydrotropes do not fulfil the criteria of a green chemical substance.
- (5) ILs are widely overestimated as green solvents. NADESs and NAHYMs can be interesting alternatives. However, they still have to prove their real usefulness. Too often, high viscosity and high hydrophilicity are significant disadvantages, although there are examples where these issues are overcome [64,65]. Really true NAHYMs, meaning directly extracted from plants, are not yet used. This will be a challenging topic for the future.
Most urgent for the research in the growing field of green solvents is, in our opinion, to focus on the relevant questions in this context, that is, not to waste time and money with apparently green solvents of no further use, but instead to concentrate on an application-oriented development of green solvents for distinct processes.
Acknowledgements
We thank the reviewers for their very valuable comments that allowed us to significantly improve the manuscript. W.K. is grateful to the French Government for the Gay-Lussac-Humboldt-Prize allowing him to pursue his research in the field of green processes together with his French colleagues, in particular Professor Farid Chemat and Professor Thomas Zemb.