1 Introduction
Vegetable oil is a natural source of sterols, which are called phytosterols when extracted from plant matter. Phytosterols are bioactive compounds composed of four rings with a long hydrocarbon chain extending from the carbon labelled 17 in Fig. 1, as well as a hydroxyl group on the 3-carbon [1–3]. Phytosterols are classified based on the length, branching and olefin content of their principal aliphatic hydrocarbon chain, as well as the substituents of the 3-carbon. More than a hundred different phytosterols have been identified. The most abundant are β-sitosterol, stigmasterol and campesterol (Fig. 1).
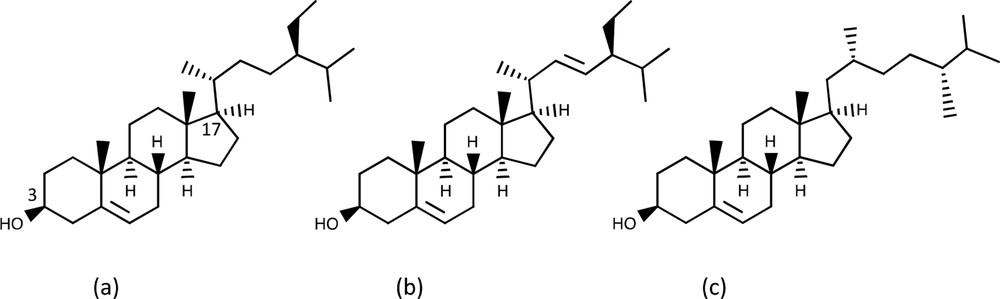
Chemical structure of b-sitosterol, stigmasterol and campesterol.
Phytosterols occur naturally without conjugation or as an ester resulting from an esterification at the 3-carbon with fatty acids or hydroxycinnamate. The relative proportion of these esterified forms depends on plant species and its location in plant [2–6].
Sterols have a number of pharmaceutical, food and cosmetic applications, and have been demonstrated to have some health benefits. When used as supplements to a normal diet, sterols can reduce cholesterol accumulation, which is suggested to decrease the risk of coronary heart disease [2,4–6]. Cholesterol reduction is also observed in conjunction with the use of steryl esters, phytostanols or stanyl esters as well as sterols [2,7]. This is a critical point, because these sterol derivatives have better solubility in water than sterols and are preferred for the enrichment of food [2]. Plant sterols have also been demonstrated to have anti-inflammatory and immunomodulatory properties and to reduce lipid accumulations in arterial walls [6,8–14]. Plant sterols are also thought to have antioxidant and anticarcinogenic properties [2,5,6,15–21].
In addition to their nutritional and health benefits, sterols are also precursors in the synthesis of steroid hormones [5,22].
In industry, phytosterols can be recovered from vegetable oil through a refining process called deodistillation, which removes the flavour and odour of the vegetable oil [23]. During this step, the major part of sterols is expected to remain in the final refined oil to guarantee its nutritional and health benefits. However, the difference in sterol concentrations between the crude oil and the refined oil may vary between 14% for corn or palm oil and 7–9% for rapeseed or soybeans [2]. Corn and rapeseed are the richest sources of sterols with average total sterol contents per 100 g of refined oil of, respectively, 700–750 and 750–800 mg. For the sake of comparison, soybean and palm refined oil contains about 300 and 60 mg, respectively, of sterols per 100 g of oil. In addition, the deodistillate stream contains a variety of sterols as well a wide variety of lipids, glycerides and hydrocarbons, most notably including tocopherol [1,24]. Also known as vitamin E, tocopherols are one of the most valuable components in deodistillate and are frequently extracted along with sterols [25,26]. Typical phytosterol content for several oleaginous plant species is presented in Table 1 [22,27–29]. Genetic factors, cultivation and agronomic parameters all influence the total phytosterol content and the phytosterol profile [4].
Total sterol content in different crude vegetable oils (in g/kg of extracted oil).
Crude vegetable oils | Brassicasterol | Campesterol | Stigmasterol | β-Sitosterol |
Corn oil | 0.00–0.01 | 1.01–1.29 | 0.43–0.98 | 4.26–9.89 |
Rapeseed oil | 0.55–0.85 | 1.54–3.10 | 0.02–0.04 | 2.28–4.02 |
Sunflower oil | Not detected | 0.28–0.69 | 0.24–0.75 | 1.72–4.65 |
Soybean oil | 0.00–0.01 | 0.52–0.76 | 0.44–0.76 | 1.08–2.31 |
Olive oil | Not detected | 0,05–0,06 | 0.01–0.02 | 0.90–1.25 |
Canola oil | 0.71–1.30 | 2.17–2.78 | 0.02–0.04 | 3.61–4.19 |
Isolation of sterols and other high-value components of vegetable oil deodistillate has been a topic of study since at least the 1940s, when Hickman and Rochester [30] recovered steryl esters from oil refinery waste. They did this by first saponifying the oil, thus separating any glycerides as a solid fraction. The diethyl ether extract was then concentrated by evaporation, and finally recrystallized with the addition of methanol. This protocol has served as a benchmark for sterol extraction, although improved methods have since been demonstrated to have higher yields and lower energy costs.
Recently, lipase enzymes have been used to efficiently separate sterols from fatty acids by selectively esterifying either fatty acids or sterols, thereby permitting separation of the two components based on whether they are esterified [25]. For instance, Ramamurthi and co-workers used a lipase to convert all of the fatty acids in soybean and canola deodistillates to methyl esters, which were then distilled to produce a residue rich in both sterols and tocopherol [31,32]. The use of these enzymes precludes the use of harsh chemicals, which would otherwise be used, and improves the purity and yield of the extract. Recent advances in the search for new lipases and the development of Candida rugosa lipases may allow us to further develop this type of process [33,34].
The next part of this work reviews these new lipase-assisted sterol extractions and their main advantages and drawbacks in economic and environmental terms. Optimization of sterol recovery by enzymatic and non-enzymatic ways is discussed to provide key principles for sterol production.
2 Several ways to recover sterol
Literature shows that phytosterols can be extracted from two major raw materials: oleaginous plants and tall oil [2].
At first, oil is extracted from oleaginous plants by a combination of pressing and solvent extraction. Unwanted impurities and residues are then removed from the oil to refine it to food grade. The steps of this refining process (Fig. 2) typically include degumming (the removal of insoluble components), winterization (the removal of high melting point waxes), bleaching and finally deodorization. There are in fact a variety of vegetable oil refining processes that can be classified into two categories: physical and chemical refining [25,35–37]. Essentially, the two categories are distinguished by the way in which free fatty acids are removed from the oil: chemical processes neutralize the acids, causing them to separate from the oil, whereas physical processes separate the acids by distillation.

Crude oil refining process.
In terms of sterol recovery, the last step of the refining process—deodorization—is the most important. This process is required for the removal of flavour and odour compounds, which would cause the oil to have undesirable sensory characteristics. Deodorization is a type of distillation process, in which a small quantity of water vapour is injected in oil heated to between 180 and 220 °C under reduced pressure (typically around 2 mbar) for approximately 2 h. In physical refinement, deodorization may occur at a higher temperature (up to 260 °C) to remove free fatty acids. Chemically refined oil is free of free fatty acids during deodorization because of the aforementioned neutralization step, so a lower distillation temperature is permissible. The distillate, referred to as deodistillate (a contraction of “deodorization distillate”), contains an assortment of organic compounds including pesticides, polyaromatic hydrocarbons, oxidation products, tocopherol, sterol and (in the case of physical refining processes) free fatty acids [23]. The deodistillate composition depends on oil refining type (chemical or physical), vegetable oil origin, and deodistillation operating conditions [1,24].
Deodistillate is a valuable source of fatty acids for biodiesel production, as well as a source for tocopherols, sterols and squalene. However, the extraction of these components at high yield and high purity remains a technical challenge [25,26].
2.1 Conventional (non-enzymatic) separation of sterol from deodistillate
As mentioned in Section 1, the classic method for isolating sterols from deodistillate is by first saponifying the fatty acids (saponification refers to base-mediated conversion of glycerides to glycerol and fatty acid salts, which precipitate as soap), then washing the remaining liquid with diethyl ether and recrystallizing sterols in the diethyl ether extract by slow addition of methanol.
Improvement in this older experimental approach is more recent and includes mostly further purification or energy reduction. Martins et al. [38] saponify thus soybean deodistillate followed by an acidification. A molecular distillation process in five steps is carried out to separate free fatty acids (in distillate) from tocopherols and sitosterol (in residues). An enriched fraction of sterols and tocopherols is recovered (35% of tocopherol and 25% of sitosterol). Kasim et al. [39] developed a process in two steps with an extraction solvent (modified soxhlet) on soybean deodistillate before a cold saponification (<60 °C) followed by a liquid–liquid extraction. They obtained an enriched fraction of tocopherols and sterols (38% and 55%, respectively) with good recoveries (94%). In Charlemagne et al. [40], a saponification of a rapeseed and sunflower deodistillate mix is performed with potassium hydroxide and ethanol at 80 °C. After cooling, phytosterol crystals are recovered by centrifugation. Recovery of sterols is announced at more than 97% with a purity of 96%.
In Dobbins et al. [41], deodistillates from soybean and canola oils are also saponified with methanol and potassium hydroxide. With addition of water, a cooling is applied and sterols are precipitated. Filtration or centrifugation combination to a washing provides a recovery of 85% for sterol with a purity of 95%. Acidification and distillation allow one to recover a rich fraction of tocopherols. Saponification could also be executed to separate free fatty acid on a mix of rapeseed and sunflower chemical and physical distillates. Next, esterification of residue is followed by a short-path distillation process. Sodium methoxide with methanol or benzoic acid treatment breaks steryl ester bonds. Free sterols are further recovered by crystallization with 95% purity [42]. Yan et al. performed a similar extraction on soybean deodistillate but separated sterol with a solvent crystallization (water/petroleum ether approximately in a volume ratio of 1:4). The yield of sterol extraction is 70% with 96% of purity [43].
Despite all these improvements, this classic process is environmentally costly in terms of the quantity of soap produced, and the consequent downstream refinement processes that are required. This process also tends to degrade tocopherols, which could otherwise have been recovered as an additional profit stream [1]. Consequently, it is preferable to isolate sterols without saponification.
To avoid saponification, the fatty acids in the deodistillate can be esterified and sterols subsequently crystallized out of the mixture. This was demonstrated in an experiment in 1964 in which hydrochloric acid was used to catalyze the esterification of fatty acids. The oil was then rinsed with a mixture of methanol/water and then with hexane. The residual methanol and water were then distilled from the hexane fraction, and acetone added to precipitate sterols, which were recovered with a purity between 95% and 97% [44].
A variety of esterification catalysts have since been screened for their effectiveness in this process (i.e, butylstannoic acid, manganese diacetate dihydrate, lanthanum triacetate, zinc acetate dihydrate, dibutyltin oxide or lanthanum triacetate hydrate), leading to sterol extracts with purities of up to 98% [45]. Finally, an 85% yield of sterols with 98% purity was reported with the use of sulfuric acid in methanol. After transesterification, the methanol is vaporized and the mixture is washed with hot water and then cooled to precipitate sterols [46].
Another process design, more recent, consists to esterify fatty acids and perform distillation to separate methyl ester. Next, sterols are separated by crystallization. In Fizet [47], esterification is performed on soybean deodistillate, followed by a stepwise distillation process. Residue is treated to break sterol ester bond (with sulfuric acid and methanol use) and sterols are crystallized by cooling with purity and yield in sterol of 90%. Jeromin et al. [48] carries out an esterification of fatty acids on soybean deodistillate followed by a distillation process to recover methyl ester and rich fraction in steryl ester. Steryl ester bonds are broken with sodium methylate and methanol. Wollmann et al. [49] optimized this methodology under milder conditions with a crystallization followed by an acidification. These sterols extracted present a purity of 94%.
A multistep process involving esterification, distillation, transesterification and further distillation for recovery of tocotrienols/tocopherols from vegetable oil distillates from refineries was also proposed by San [50]. Esterifying step used alcohol (methanol, ethanol, propanol and butanol, alone or combined) in the presence of an acid catalyst (hydrochloric, acetic, sulfuric or citric acids) to convert the fatty acids contained therein to produce an esterified mixture comprising fatty acid esters.
More advanced process is also described by Yaohui et al. [51]. They performed a methyl esterification on corn germ oil deodistillate using a cation-exchange resin. Sterols are recovered by crystallization after cooling. Purification with solvent and/or cyclodextrin microencapsulation is described as a convenient purification strategy.
Literature describes also extraction protocols based on physicochemical mechanism, namely selective crystallization of sterols by modification of deodistillation polarity with addition of suitable solvents. In this methodology, extraction yields are quite good but the purity is lower than the pure “chemical” extractions described previously. In Lin and Koseoglu [5], soybean oil deodistillate is treated in a 1:3 deodistillate/solvent ratio with a mixture of two solvents, i.e., methanol and acetone (1:4 volume ratio). Temperature is maintained at a value of −20 °C for 24 h to recover sterols by crystallization with 80% yield. In Chen et al. [52], Canola deodistillate samples were extracted with a combination of absolute methanol (3 min) and methanol/2-propanol (1:1, v/v) three times. Supernatants were filtered using a Whatman No. 1 filter paper and evaporated to dryness under N2.
Furthermore, Aachary et al. [53] used a set of different solvents such as hexane, chloroform, petroleum ether, cyclohexane and toluene. Deodistillate was first solubilized with the various solvents (1:20, w/v), followed by extraction with 3 mL of 50% methanol three times. Methanolic extracts were evaporated to dryness in a rotary evaporator.
Temperature rate is a crucial parameter for this approach. Shao [54] demonstrates that a slow cooling at 5 °C/min after a preliminary heating at 45–50 °C can improve both extraction yield and purity.
The main advantage of solvent crystallization process is facility to handle, but solvent cost and environment concern can decrease its interest even if solvent is recycled through distillation or the use of “greener” solvents could increase the global performances of this simple methodology [5].
Dumont and Narine [25] also showed that supercritical carbon dioxide extraction gains in interest. However, this methodology is more efficient for tocopherol isolation than for sterol recovery [55,56]. Chang et al. optimized the supercritical CO2 operating parameters on soybean oil deodistillate and concentrated sterols in raffinates with purity lower than co-extracted squalene and fatty acid process [57]. The best results were, however, obtained by Yaping and Dapu [58]. They isolated sterols with a quite good purity (90%) but low yield (55%).
Literature also reports very specific and multistep chemical processes to recover sterols with the highest purity. For instance, Gunawan and co-workers performed a process that includes molecular distillation, Soxhlet extraction, silica gel column chromatography and water/acetone extraction [1,26].
A wide diversity of chemical processes is thus reported to extract sterol with good yield and purity. Technical and environmental concerns are usually suitably reported in the literature. However, the economical viability of these approaches at an industrial scale remains associated with “external” parameters, such as deodistillate prices (fluctuating mostly since 2010) and the ability to valorize further other deodistillate molecules (mostly tocopherols for the cosmetic industry).
2.2 Enzymatic separations
A convenient way to preserve tocopherols from degradation (observed sometimes in chemical processes such as esterification) is the use of enzymes instead of chemical reagents. Even if attractive, enzymatic processes tend to be more expensive than processes based on the use of chemical reagents. This cost is justified in circumstances where a high degree of selectivity is necessary, or where the substrate requires benign reaction conditions. In the context of deodistillate mixtures, enzymatic processes are attractive for two reasons: first, they can be performed without excessive heating, thereby reducing degradation of valuable tocopherol molecules; second, they are capable of selectively transesterifying specific molecules (fatty acids or steryl esters), which permit these molecules to be separated based on the character of their substituent esters [25].
Ramamurthi and co-workers were the first to explore the enzymatic esterification of fatty acids to concentrate sterol and tocopherol. An immobilized lipase (Randozyme SP) was used to convert glycerides to methyl esters, which could then be distilled to yield a residue rich in sterols and tocopherol [31,32]. Processes using immobilized enzymes have the advantage that the enzymes are not entrained with the reaction medium, so the enzymes are more easily recovered after the process, which permits them to be used for a greater number of treatment cycles.
This basic process has been improved upon through the use of elaborate separation processes, including ultrasound-induced crystallization with methanol followed by filtration. Furthermore, molecular distillation is performed on filtrate to separate tocopherols and fatty acid methyl esters, which is valorized as biodiesel. A treatment under ultrasound assistance with acetone/ethanol is also performed to increase sterols purity [59]. An alternative option was also reported by Peilong et al. [60]. They used first solvent crystallization to separate sterols from deodistillate before applying lipase. A mixture of methanol/acetone is added to the deodistillate with a progressive cooling to crystallize selectively sterols. In this process, steryl esters are separated by distillation and tocopherols are recovered with free fatty acids and triglycerides.
Usually, enzymatic processes include an esterification step of sterols in steryl esters to modify their corresponding ebullition points and facilitate their separation by distillation. This method leads to a supplementary step if the goal is to obtain free sterols. The hydrolysis of the ester bond in those separated steryl esters and a subsequent separation of free fatty acids must be yet done, increasing the global cost of the process. Nagao et al. [61] propose a process to esterify free sterols from a soybean oil deodistillate, allowing the extraction of tocopherols through distillation and ethanol fractionation. Residue obtained has a concentration in sterols of 75%, with small amounts of free fatty acids as minor impurities. Lipase from C. rugosa was identified as candidate with the best esterification degree (82%). Furthermore, steryl esters could be purified with n-hexane extraction followed by silica gel column chromatography.
Nagao et al. [62] also worked on soybean deodistillate that high boiling point substances (steryl ester, di- and triacylglycerol) were removed by distillation (240 °C at 0.02 mm Hg). Esterification of sterols and free fatty acids was carried out in two steps with C. rugosa lipase. Although water content of about 3% was required for the enzyme activity, a continuous water removal under reduced pressure (20 mm Hg) was found to increase esterification yield (up to 96%) of sterols. Different lipases were screened to convert free fatty acids in fatty acid methyl esters. Lipases of Alcaligenes were used to convert free fatty acids in fatty acid methyl esters with a yield of 95%. The product was then fractioned with short-path distillation and steryl esters were recovered in the final residue while tocopherols and fatty acid methyl esters were separated in different fractions.
Shimada et al. [63] worked on the same pretreated soybean deodistillate by distillation to remove high boiling point substances. Lipase from C. rugosa was used in two steps to convert free sterols in steryl esters. After the first esterification, a distillation process was performed to remove steryl esters. A second esterification with lipase from C. rugosa was performed to convert residual free sterols in steryl esters. A distillation process was then performed to separate free fatty acids and tocopherols. To purify steryl esters, Na-methylate is used to promote methanolysis of steryl esters in free sterols and free fatty acids. Watanabe et al. [64] used the same process and studied methanol influence during esterification with lipase from C. rugosa. A two-step in situ enzymatic reaction was executed. In the first step, lipase from C. rugosa esterified sterols in steryl esters. Methanol was then added to convert fatty acids in fatty acid methyl esters with the same enzyme. A distillation process was performed before the second step to remove fatty acid methyl esters. A last step of lipase esterification was performed under similar conditions with the objective to increase yield (86%) and purity (97%).
Shimada et al. [65] studied also the possibility to hydrolyze steryl ester bonds with lipase and make esterification of free fatty acids. Different lipases were screened for the hydrolysis, i.e., lipases from C. rugosa, Pseudomonas aeruginosa, Pseudomonas stutzeri, Burkholderia glumae and Burkholderia cepacia. All of them were able to hydrolyze 55% of steryl ester bonds. When methanol was added to esterify fatty acids, lipase from Pseudomonas aeruginosa (LPL from Toyobo Co. Ltd., Osaka Japan) and P. stutzeri (Meito Sangyo Co. Ltd., Aichi, Japan) was found efficient. The reaction needed a minimum of 10% of water and a 2:1 molar ratio of methanol/steryl esters. Yield of transformation was superior to 98% and free sterols were separated using a n-hexane fractionation. These reactions were performed with oleic acid ester with 92.6% of purity.
In Norinobu et al. [66], a saponification was performed on soybean deodistillate to transform acyl glycerides in free fatty acids and consequently steryl esters in free sterols. A specific sterol esterification was done with a lipase from the genus candida (Lipase OF). A distillation process was performed to separate steryl esters. Purity of steryl esters was up to 96% with a recovery of 85–90% of sterols. In Torres et al. [67], soybean deodistillate was esterified in two steps, one enabling esterification of sterols using a lipase from C. rugosa, and the second one to esterify fatty acids with ethanol in the presence of a lipase from Novozyme 435. Oleic acid was added to reduce melting point of soybean deodistillate. Recycling of these enzymes was studied and showed a good efficiency for eight consecutive runs with a lipase activity maintained at 80%.
In Weber et al. [68], chemical and physical rapeseed oil deodistillates, added or not with soybean deodistillate, were studied for the enzymatic production of steryl esters and their purification. Sterols were converted with C. rugosa lipase in vacuum at 40 °C. Purification with diethyl ether and deacidification was performed followed by column chromatography purification and solvent fractionation using an acetone/water mixture. Purity was more than 90% whereas conversion of sterols was calculated between 87% and 97% as a function of the raw material investigated.
Involvement of enzymes for sterol extraction from deodistillate is less developed (at a laboratory scale or an industrial scale) than pure chemical extractions mostly because of the cost associated with the enzymes. To reduce the operational costs, the concentration of enzyme should be kept as low as possible [69]. Teixeira et al. used low concentrations of enzyme (0.25–0.5% w/w) for obtaining high steryl ester yield (70.5–87.7%) from sunflower deodistillate. The optimal conditions were found to be 0.5% of concentration, 24 h of reaction time, water activity between 0.45 and 0.60 and substrate molar ratio (free fatty acid/S) of 6:1[69,70].
In this sense, immobilized enzymes and their recycling for subsequent runs is a huge field of research, mostly under consideration in biodiesel production technology. Even if a lot of fundamental and applied studies are thus performed on vegetable oils to produce biodiesel, the transposition of the results of deodistillate remains challenge because of inherent physicochemical (melting point, etc.) and compositional differences (presence of water, variability between the chemical and the physical deodistillate, presence of impurities, etc.) between vegetable oils and deodistillates.
The orientation toward “full enzymatic process” remains currently difficult as a “classical” chemical reaction is often still required to hydrolyze steryl ester bonds. Even if literature describes nowadays several options to ensure this hydrolysis under enzymatic conditions, the research is not yet advanced for an industrial transfer.
3 Conclusion
The use of lipases presents a more efficient and benign process to separate sterols from vegetable oils through selective functional group interconversion of specific molecules to facilitate separation. The optimization and fundamental comprehension of the mechanism have been ensured on soybean oil deodistillates and the transposition to another deodistillate needs still some adjustments. Using lipase to hydrolyze steryl ester needs more intensive research to develop a full enzyme design process.
Acknowledgments
This work was supported by “Region Wallonne”.