1 Introduction
Corrosion has a serious adverse effect on modern industry and life, especially metal corrosion. It causes destruction or deterioration of the metal under the action of external environmental factors. Corrosion of stainless steel has been investigated by many researchers [1–5]. Corrosion not only changes the original gloss of the metal, but also causes great economic loss and serious social harm to the society. As a result, corrosion inhibitor has been widely used in many fields because of its strong applicability, which can effectively reduce the degree of metal corrosion, reduce unnecessary economic losses, and contribute to environmental protection and the rational use of resources. The use of corrosion inhibitors is good for human society. The investigation of corrosion inhibition in steel is a matter of high theoretical and practical importance [7]. The mechanism of anticorrosion or corrosion inhibition in the process of metal protection has been the concern of many scholars, but there is still no unified opinion concerning this at the moment. At present, the action of a corrosion inhibitor can be summarized as follows: first, electrochemical mechanism, which is based on the electrochemical process on the metal surface that explains the anticorrosion effect of an inhibitor; and second, physicochemical mechanism, which is based on the physicochemical changes in the metal surface that explain the corrosion inhibition action of the inhibitor. Simply put, the corrosion inhibitor protects metal mainly by forming a protective film on its surface, which hinders corrosion reaction, thereby resulting in corrosion prevention. In this study, the effect of the alkyl chain of quaternary ammonium cationic surfactants on corrosion inhibition in HCl solution was investigated by using dodecyl trimethyl ammonium chloride (DTAC), tetradecyl trimethyl ammonium chloride (TTAC), cetyl trimethyl ammonium chloride (CTAC), and octadecyl trimethyl ammonium chloride (OTAC) as corrosion inhibitors to uncover their structure–efficiency relationships.
2 Experimental design
2.1 Corrosion inhibition evaluation
A3 steel strips, containing 0.02% C, 0.0017% Si, 0.237% Mn, 0.015% P, 0.0016% S, 0.008% Cu, 0.014% Cr, 0.013% Ni, 0.001% Mo, 0.01% Al, and the remaining proportion as iron, were used for gravitational measurement. The corrosion performance was carried out on the steel strips using the gravitational method. The strips were mechanically polished on wet SiC paper (grade 360-600-1200), rinsed with distilled water, transferred to petroleum ether, and immersed in absolute ethyl alcohol [8]. Two pieces of the A3 steel in 5% HCl solution (120 mL) were then suspended [6] in the absence and in the presence of inhibitors of cationic surfactants at different concentrations (the concentrations were 70.0, 50.0, 20.0, 10, 5.0, and 2.5 mg/L and blank solution, respectively) for 3 h. The corrosion temperature ranged from 303 to 343 K [9]. The A3 steel sheets were brought out, rinsed with distilled water, soaked and cleaned in acetone solution, then washed in anhydrous ethanol solution. They were then placed on a quantitative filter paper and were dried with a hair dryer. Their weights were measured after blow-drying was completed on each one of them.
2.2 Computational methods of inhibition efficiency
The inhibition efficiency was calculated as follows:
(1) |
The corrosion rate was calculated by the following relation:
(2) |
2.3 Adsorption
Assuming that the adsorption law of the corrosion inhibitor used in this experiment conforms to the Langmuir adsorption model, then
(3) |
2.4 Adsorption thermodynamic parameters
Adsorption thermodynamic parameters play an important role in the study of the adsorption mechanism of corrosion inhibitors. The heat of adsorption can be calculated by the Van't Hoff equation:
(4) |
(5) |
(6) |
The apparent activation energy (Ea) during dissolution of the steel sheets could be evaluated from the Arrhenius equation [11]:
(7) |
3 Results and discussion
3.1 Effect of inhibitor concentration
Fig. 1 shows that at 303 K, with increase in the concentrations of inhibitors, the rate of corrosion decreased and the corrosion inhibition efficiency increased continuously. It can also be seen from the result that all of the four surfactants (DTAC, TTAC, CTAC, and OTAC) acted as inhibitors to steel through adsorption on the A3 steel surface and formation of a barrier layer between the metal and the corrosive media. However, the four corrosion inhibitors had almost the same efficiency, and there was no obvious difference. This shows that the alkyl chain has little effect on quaternary ammonium cationic surfactants at low temperature.
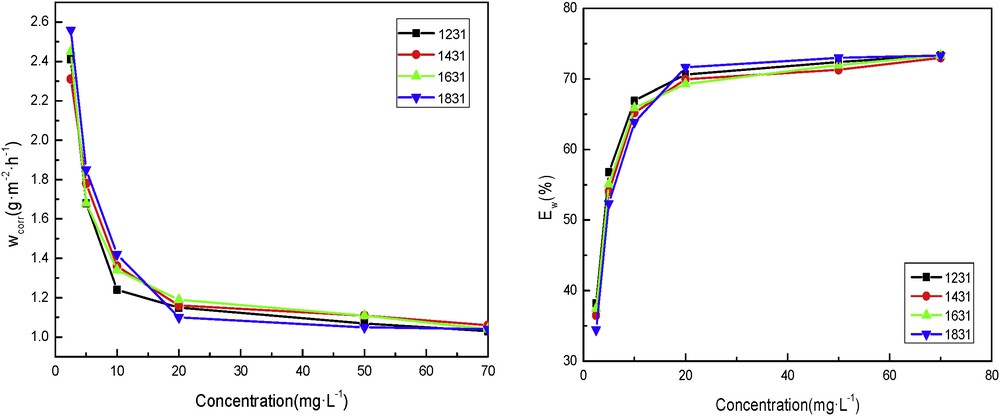
Inhibitor rate (left) and efficiencies (right) of corrosion for the different concentrations of the cationic surfactants in 5% HCl at 303 K.
Fig. 2 shows that at a certain temperature, with increase in the concentrations of inhibitors, the corrosion inhibition efficiency increased continuously and the rate of corrosion decreased, which shows that the inhibition actions are because of its adsorption on the mild steel surface as suggested in previous research. It can be seen from Fig. 2 that the corrosion rate and the corrosion inhibition rate of different cationic surfactants are obviously different when the temperature increases. The corrosion inhibition efficiency of DTAC is the best, which shows the influence of the alkyl chain on the cationic surfactant. The longer the alkyl chain is, the larger the molecular weight is, the lesser the quantity of the cationic surfactant is at the same concentration, and the lesser the molecules are adsorbed on the steel surface. This condition worsened slow release effect. At the same concentration, the longer the alkyl chain, the lower the corrosion inhibition efficiency.
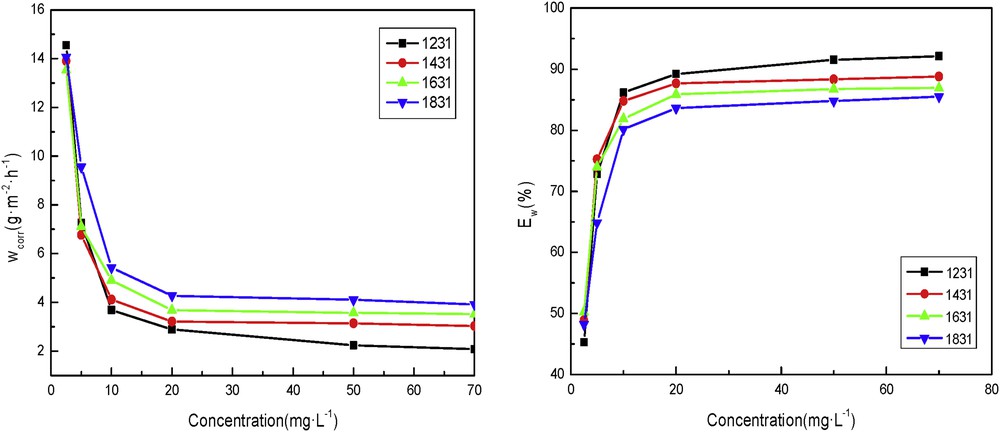
Inhibitor rate (left) and efficiencies (right) of corrosion for the different concentrations of the cationic surfactants in 5% HCl at 323 K.
Fig. 3 shows that at 343 K, with increase in the concentrations of the inhibitors, the corrosion inhibition efficiency increased continuously and the rate of corrosion decreased. It can be seen from Fig. 3 that the inhibition effect of DTAC is the best and that of OTAC is the worst. This is because the increase in temperature promotes the adsorption of cationic surfactants on the steel surface. Corrosion inhibitor molecules covered most part of the surface of the stainless steel, which hindered the collision of active atoms in stainless steel with H+ in HCl, thus reducing the corrosion rate. The molecules of DTAC were more than the other three surfactants. Therefore, DTAC had the highest inhibition efficiency.
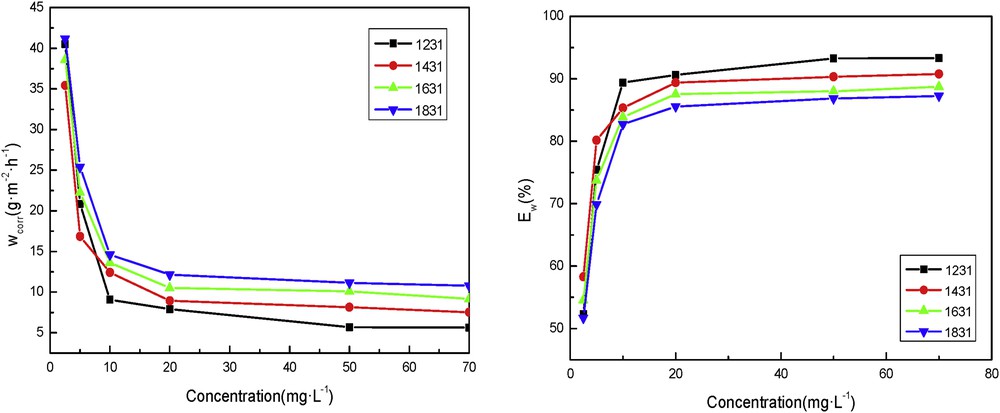
Inhibitor rate (left) and efficiencies (right) of corrosion for the different concentrations of the cationic surfactants in 5% HCl at 343 K.
In conclusion, at low temperature, the corrosion inhibition effects of the four surfactants at the same concentration are almost the same. However, when the temperature rises more than 323 K, it becomes obvious that the four inhibitors have different efficiencies. When the temperature was 343 K and the concentration of inhibitor used was 70 mg/L, the corrosion inhibition efficiencies of DTAC, TTAC, CTAC, and OTAC were 93.31%, 90.76%, 88.75%, and 87.25%, respectively. It can be seen that DTAC had the best corrosion inhibition effect, and the order of the rest is TTAC > CTAC > OTAC. This is because at the same mass concentration, the alkyl chain of DTAC is the shortest, the molecules of DTAC are more than the rest three surfactants and thus, the adsorption on the steel is more; therefore, DTAC had the highest corrosion inhibition efficiency. The number of molecules available as well as the adsorption on steel surface decreases as the alkyl chain increases, which leads to the reduction of corrosion inhibition effect.
3.2 Effect of temperature
To investigate the effect of temperature on the anticorrosion of the four surfactants, the inhibition of 20 mg/L of each of the four surfactants in 5% HCl solution was evaluated as shown in Fig. 4. The effect of temperature in the range of 303–343 K was evaluated in the absence and in the presence of the same concentration of surfactant for 2 h of immersion. From the result obtained, it can be observed that the rate of corrosion increased sharply and the inhibition efficiency increased slightly with increase in temperature. This may be because higher temperature accelerates hot movement of the organic molecules and strengthens the adsorption capacity of inhibitor on the metal surface. Fig. 4 also shows that the corrosion inhibition efficiency of each surfactant at 343 K is the best temperature, and the effect of each surfactant at 303 K is relatively poor. Because of the general solubility, the adsorption of each of the four corrosion inhibitors on the steel surface was weakened, resulting in a poor corrosion inhibition at 303 K. At the same time, increasing the temperature of the inhibitors resulted in increased solubility of the inhibitors, so that the slow-release effect at 343 K became relatively good. However, the corrosion of steel by acid is accelerated if the temperature is very high, because a large number of bubbles will be produced on the surface of the steel, which will either make the adsorption of corrosion inhibitor molecules difficult or cause the desorption of inhibitor molecules from the steel surface.

Inhibitor rate (left) and efficiencies (right) of corrosion in 5.0% HCl with 20 mg/L cationic surfactants at different temperatures in the range of 303–343 K.
3.3 Effect of acidity
As a kind of corrosion medium, the amount of HCl directly affects the pH value of the solution and the corrosion rate of the suspended sheet. Fig. 5 shows the inhibitor efficiency and the rate of corrosion at 323 K with 20 mg/L cationic surfactant at different concentrations. It can be seen from Fig. 5 that with the increase in the HCl concentration, the corrosion rate increased and the inhibitor efficiencies decreased. This is because with the increase in HCl concentration, the pH of solution decreased, the concentration of the hydrogen ion in corrosion medium increased, and a large number of hydrogen ions and inhibitor molecules compete for adsorption on the surface of the corrosion matrix, which reduced the adsorption rate of inhibitor molecules on the suspended steel sheets. The film-forming inhibition performance of the corrosion inhibitor was inhibited, thus increasing the corrosion rate.
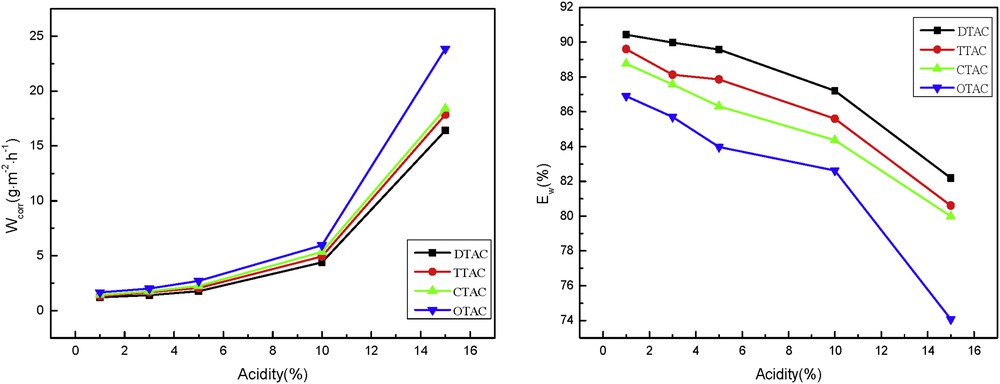
Inhibitor rate (left) and efficiency (right) of corrosion at 323 K with 20 mg/L cationic surfactants at different concentrations of HCl.
3.4 Adsorption
Adsorption isotherms can provide basic information on the interaction of inhibitor and metal surface. The degree of surface coverage as a function of the concentration of the inhibitor (c) was tested graphically by fitting it to various isotherms to determine the best fit, which describes this study. Langmuir adsorption isotherm can give the best description for the cationic surfactants on mild steel. The plots of c/θ versus c for the cationic surfactants are represented in Fig. 6, which yielded a straight line. Thus, the adsorption processes of inhibitors (the four surfactants) on the steel sheet surface in 5% HCl are consistent with the Langmuir adsorption isotherm model.

Langmuir's adsorption plots for steel sheet in 5% HCl at different concentrations of the cationic surfactants at different temperature.
Adsorption equilibrium constant (K) of the cationic surfactants of the steel surface immersed in 5% HCl at different temperatures is summarized in Table 1. The high correlation (R2) of the Langmuir adsorption isotherm for the cationic surfactants is presented for each case. The Langmuir adsorption isotherm assumes that the adsorption of organic molecule on the adsorbent is monolayered. The values of K of the inhibitors on the steel sheet immersed in HCl at different temperatures were calculated using Eq. 3. The relatively high value of the adsorption equilibrium constant reflects the high adsorption ability of the molecules on the steel sheet surface. In Table 1, the values of K for each inhibitor increased as the temperature increased, which shows that the additives capacity of adsorption increases with the increase in temperature and K is a function of temperature.
Adsorption equilibrium constant (K) of the cationic surfactants on the steel sheet surface immersed in 5% HCl at different temperatures.
Inhibitor | T (K) | R2 | Slope | K (L mol−1) |
DTAC | 303 | 0.9997 | 1.33 | 1.168 × 105 |
323 | 0.9994 | 1.06 | 1.520 × 105 | |
343 | 0.9996 | 1.05 | 1.938 × 105 | |
TTAC | 303 | 0.9997 | 1.33 | 1.316 × 105 |
323 | 0.9996 | 1.13 | 2.632 × 105 | |
343 | 0.9998 | 1.11 | 2.857 × 105 | |
CTAC | 303 | 0.9997 | 1.33 | 1.307 × 105 |
323 | 0.9997 | 1.13 | 1.632 × 105 | |
343 | 0.9998 | 1.11 | 2.801 × 105 | |
OTAC | 303 | 0.9993 | 1.32 | 1.239 × 105 |
323 | 0.9997 | 1.14 | 2.110 × 105 | |
343 | 0.9997 | 1.12 | 2.545 × 105 |
3.5 Thermodynamic study
Thermodynamic parameters are important in studying the adsorption of inhibitors. The value of K is related to the Gibbs free energy of adsorption (ΔG), which can be calculated using Eq. 5. The heat of adsorption (ΔH) is calculated using the Van't Hoff equation (Eq. 4). Fig. 7 shows the straight lines between 1/T versus ln K of the cationic surfactants. The slopes of the straight lines are equal to −ΔH/R. The heat of adsorption (ΔH) could be approximately regarded as the standard adsorption heat (ΔH) under experimental conditions. Furthermore, the standard entropy of adsorption (ΔS) is obtained by the thermodynamic basic equation (Eq. 6). The variation in the logarithm of the corrosion rate of the steel sheet in 5% HCl in the presence of 20 mg/L of the cationic surfactants as a function of temperature reciprocate is illustrated in Fig. 7. It shows that the corrosion reaction can be regarded as an Arrhenius-type process (Eq. 7).
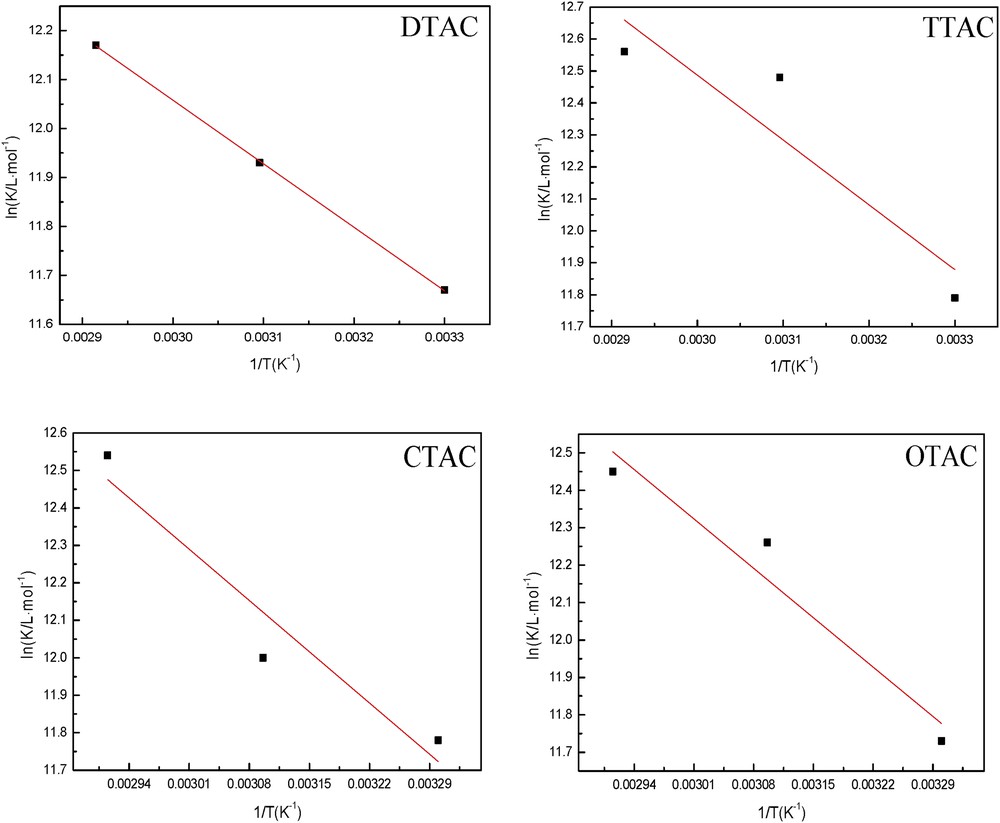
Plots of 1/T versus ln K for adsorption of the cationic surfactants on the steel sheet.
The ln Wcorr versus 1/T is represented as a straight-line function in Fig. 8. All of the linear regression coefficients (R2) were almost equal to 1, which shows that the adsorption process of the additives of the cationic surfactants on the steel samples in acid solution complied with the Arrhenius adsorption [12]. The apparent activation energy (Ea) during dissolution of the steel sheets could be evaluated from the Arrhenius equation. It can be seen from Table 2 that the activation energy of the cationic surfactants increases with the increase in length of the alkyl chain. The higher the activation energy, the more difficult it is to adsorb on the steel surface. The apparent activation energy of DTAC was smaller than that of others, indicating that desorption of other surfactants on the surface of the steel samples was more difficult than that of DTAC. Therefore, under the same conditions, the corrosion rate of DTAC solution is lower than that of the others. As the desorption of the inhibitor molecules increased at higher temperatures, the rate of corrosion increased [13].

Arrhenius plots of the steel sheet in 5% HCl with 20 mg/L of the cationic surfactants at different temperatures.
Activation parameters ΔG, ΔH, ΔS, and Ea of 20 mg/L of the cationic surfactants during the absorption on the steel sheet in 5% HCl at different temperatures.
Inhibitor | T (K) | ΔG (kJ mol−1) | ΔH (kJ mol−1) | ΔS (J mol−1 K−1) | Ea (kJ mol−1) |
DTAC | 303 | −39.512 | 10.793 | 166.023 | 41.516 |
323 | −42.827 | 10.793 | 166.007 | 41.516 | |
343 | −46.172 | 10.793 | 166.079 | 41.516 | |
TTAC | 303 | −39.812 | 16.870 | 187.071 | 43.997 |
323 | −44.302 | 16.870 | 189.386 | 43.997 | |
343 | −47.279 | 16.870 | 187.023 | 43.997 | |
CTAC | 303 | −39.795 | 16.255 | 184.984 | 47.036 |
323 | −43.018 | 16.255 | 183.508 | 47.036 | |
343 | −47.222 | 16.255 | 185.065 | 47.036 | |
OTAC | 303 | −39.660 | 15.672 | 182.615 | 50.019 |
323 | −43.708 | 15.672 | 183.838 | 50.019 | |
343 | −46.949 | 15.672 | 182.568 | 50.019 |
Physical adsorption is a kind of adsorption by means of intermolecular force. The adsorption force is weak, the adsorption heat is small (8–20 kJ/mol), and it is close to the liquefaction heat of gas. It is reversible, nonselective, and the larger the molecular weight is, the easier it is for adsorption to occur, and the stability of adsorption is not very high. Chemical adsorption is similar to general chemical reactions. It follows the traditional laws of chemical thermodynamics and chemical kinetics by means of chemical bond force. It has the characteristics of selectivity, large adsorption heat (40–800 kJ mol−1), stable adsorption, and generally irreversible adsorption. In Table 2, the positive values of heat of adsorption (ΔH) of the cationic surfactants reflect the heat-absorbing nature of the steel sheet dissolution process [14]. It also shows that the adsorption process belongs to physical adsorption. The positive values of entropy of activation (ΔS) in the present inhibitors of the cationic surfactants indicate that the activated complex in the rate determining step represents an association rather than a dissociation step, meaning that an increase in disordering takes place, going from reactants to the activated complex [15]. The values of Gibbs free energy (ΔG) were found to be highly negative values, which indicates chemisorption of the cationic surfactants on the steel surface, and at the same time, it reveals the spontaneity of the adsorption process and the stability of the adsorbed layer. Also the negative values of the Gibbs free energy (ΔG) imply that these reactions are spontaneous reactions [16]. ΔG order of the cationic surfactants is DTAC < TTAC < CTAC < OTAC, which indicates that the spontaneity of DTAC is the best. It can be seen that with the increase in the length of the alkyl chain, the value of ΔG increased gradually, and the higher the ΔG, the more difficult the reaction became. From the obtained values of Ea, it is clear that the activation energy value of the inhibitor of DTAC is 41.516 kJ mol−1, which is the smallest in this experiment and with the increase in the alkyl chain, the value of Ea increased gradually. But the corrosion inhibition efficiency of DTAC for the steel in 5% HCl is the best. The increase in activation energy is generally interpreted by an electrostatic adsorption process of the inhibitor on the steel surface [17,18].
4 Conclusion
Four surfactants, DTAC, TTAC, CTAC, and OTAC, were evaluated as corrosion inhibitors for A3 steel sheet suspended in 5% HCl solutions to study the effect of the alkyl chain of quaternary ammonium cationic surfactants on corrosion inhibition. The results obtained are as follows: at a certain temperature and the same mass concentration, the molecules decreased with the increase in the length of alkyl chain, and the adsorption on the steel sheet surface decreased, which reduced the corrosion inhibition efficiency. At the same concentration, ΔH, ΔS, and Ea increased with the increase in the length of alkyl chain. The shorter the length of alkyl chain, the easier the adsorption of cationic surfactants on the steel sheet surface, such that the corrosion inhibition capability of DTAC, whose inhibition efficiency can exceed 90% in such condition, is the best.
Acknowledgments
This work was financially supported by the grants from the National Science Foundation of China (21808182), Scientific Research Program of Shaanxi Provincial Education Department (18JC025), and Xi'an Science and Technology Planning Project (201805038YD16CG22(3)).