1 Introduction
Madder (Rubia tinctorum) is a tinctorial plant belonging to the Rubiaceae family and was cultivated in an area stretching from the Mediterranean European region to Asia. Madder dyes are present in the roots of the plant. These anthraquinone compounds are composed of two chemical populations: heterosidic and aglycone molecules (Table 1). The most important identified anthraquinone heterosides are lucidin primeveroside, ruberythric acid (alizarin primeveroside), lucidin glucoside, galiosin (pseudopurpurin-1-O-β-D-primeveroside), and rubiadin-3-O-primeveroside. The main aglycone compounds are lucidin (1,3-dihydroxy-2-hydroxymethylanthraquinone), alizarin (1,2-dihydroxyanthraquinone), the biomarker of R. tinctorum species, purpurin (1,2,4-trihydroxyanthraquinone), rubiadin (1,3-dihydroxy-2-methylanthraquinone), and nordamnacanthal (1,3-dihydroxy-9,10-dioxoanthracene-2-carbaldehyde) [1,2,3,4,5].
Chemical structures of anthraquinonic compounds of madder.
Compound name | R1 | R2 | R3 | R4 |
Lucidin primeveroside | OH | CH2OH | Xyl (1 → 6)GlcO | H |
Ruberythric acid | OH | Xyl (1 → 6)GlcO | H | H |
Rubiadin-3-O-primeveroside | OH | CH3 | Xyl (1 → 6)GlcO | H |
Anthragallol | OH | OH | OH | H |
Lucidin | OH | CH2OH | OH | H |
Alizarin | OH | OH | H | H |
Purpurin | OH | OH | H | OH |
Rubiadin | OH | CH3 | OH | H |
Nordamnacanthal | OH | CHO | OH | H |
In the pictorial sphere madder is used as a metal-complex dye named “madder lake”, and is not directly used from root powder or root extract. The use of madder lake raises the resistance of the dyes to degradation. Madder lake is a chemical coordination complex between anthraquinone dyes and a metal cation. The nature of ion differs depending on the metal salt used. Aluminum is commonly used because of the occurrence of alum (KAl(SO4)2·12H2O), but other inorganic substances have been used corresponding to different cations such as iron (as sulfate or acetate), copper (as CuSO4·5H2O), tin (as SnCl2), or chrome (as Na2Cr2O7 or K2Cr2O7) [6]. The metal-complex dye thus formed is totally insoluble in all solvents. To analyze dye molecules from lakes using liquid chromatography (LC) techniques it is necessary to release dyes by breaking the coordination of the chemical bonds of the lake. The dyes are then removable and can be identified by applying different separation methods such as LC or gas chromatography. In order not to distort the original chemical composition of the lake it is important to apply mild methods and thus avoid the hydrolysis of glycosidic compounds. The native chemical composition of the madder used to prepare the lake can provide important information concerning its formulation and the maturity state of the plant.
During the extraction process heterosidic compounds can be degraded into aglycone compounds in the presence of a strong acid such as HCl or H2SO4 and with or without heating. Pseudopurpurin or munjinstin can be, respectively, decarboxylyzed into purpurin and xanthopurpurin [7]. Lucidin primeveroside can be degraded to lucidin glucoside or lucidin, and ruberythric acid may also be degraded to alizarin glucoside or alizarin. Oxalic acid, a natural organic acid, hydrolyzes anthraquinone glycosides at high temperature but an extraction at a lower temperature (60 °C) allows heterosidic compounds to be preserved.
Several mild methods of anthraquinone extraction were recently developed to analyze madder complexes in lakes or on dyed textiles. Sanyova and Reisse [8] developed a technique using hydrofluoric acid and chelating compound (EDTA) to extract madder dyes from lakes. Aluminum-fluoride complexes compete with Al3+ ions of anthraquinone phenolate groups in metal-complex dyes. This process preserves glycosidic compounds like lucidin primeveroside, ruberythric acid, pseudopurpurin, rubiadin precursor, anthragallol, and munjistin and conserves a better extraction of alizarin, purpurin, rubiadin, and lucidin aglycones. Lombardi et al. [9] proposed a dye extraction in alkaline conditions using ammonia in association with disodium EDTA to preserve glycosidic compounds present in lakes and textiles. Valianou et al. [10] compared different methods using HCl, formic acid, citric acid, oxalic acid, or trifluoroacetic acid (TFA) in heating conditions. HCl, TFA, and oxalic acid are powerful solvents to extract anthraquinone dyes. Bourhis et al.[11] applied an acetic acid buffer solution (1 M) to successfully extract flavonoids from Buckthorn, thus preserving the native chemical composition of the plant. The latter method was based on aluminum-acetate ion (Al-(AcO)2+) complexes in competition with aluminum–dye complexes promoted at pH 4.3. Formic acid in methanol/water heated at 100 °C in the presence of EDTA can be used to extract madder dyes from textiles [12].
Recent studies describe the use of oxalic acid in different activity areas in association with other techniques. These studies demonstrated oxalic acid's potential for metal cation extractions present in different matrices. It is therefore a natural and universal chelating agent for extraction of metal [10,13,14,15,16,17,18,19].
Ultrasound has already been applied to optimize the extraction of flavonoids from lakes by Bourhis et al. [11], and in this study, the use of an acetic acid buffer solution with sonication resulted in an increase in the recovery of yellow dyes without any chemical degradation as opposed to strong acid decomplexation. The application of microwave by thermal and nonthermal effects could increase yields, selectivity, and the rapidity of certain organic reactions [20]. This technique was used in an attempt to increase decomplexation and reduce the analysis time.
The aim of the present study was to test different acidic solutions associated with two extraction processes, ultrasound and microwave, to propose a universal method of decomplexation without degradation of heterosides and which would be appropriate for studies of colored lake samples or dyed textiles, irrespective of the nature of the metal cation in the formulation of the lake. In this context, several madder lakes were prepared by varying the nature of the mineral substance (KAl(SO4)3 12H2O; CuSO4; SnCl2; ZnCl2; K2Cr2O7; FeCl3; and FeSO4). At first, the objective was to evaluate the decomplexation yield of these different methods as compared with the reference strong acidic (HCl) method on an alizarin experimental lake. Two different solutions of decomplexation were tested, the first being the acetic acid buffer solution developed by Bourhis et al. [11], and the second, a solution of oxalic acid (0.5 M) in methanol/water solvent (1/1, v/v). For both solutions, ultrasound and microwave were tested to determine the most appropriate extraction process. The second step was to compare the efficiency of the four nondenaturing methods to decomplex dyes in different madder lakes to establish the most efficient and universal technique. The chelating potential of oxalic acid related to the ultrasonic or microwave process was tested particularly to improve the extraction of metal from the lake and thereby release the dyes. Analyses in LC coupled with a photodiode array detector (LC–PDA) were performed to (1) characterize the dyes extracted and particularly to evaluate the possible alteration reactions because of the process, and (2) to determine the yield and thus the efficiency of the process.
2 Experimental section
2.1 Materials
Solvents and reagents were all of analytical grade from Merck (Darmstadt, Germany). Alizarin and purpurin were purchased from Acros Organics (Geel, Belgium). Lucidin primeveroside, ruberythric acid, rubiadin primeveroside, and rubiadin were kindly supplied by Pr. V. Golicov (Russian Research Institute for Cultural and Natural Heritage, Moscow, Russia). Lucidin primeveroside was hydrolyzed (HCl) to obtain lucidin whose structure was characterized on the basis of chemical and spectral evidence including two-dimensional NMR experiments (COSY and NOESY 1H–1H, HMQC, and HMBC) and mass spectrometric techniques (EI, HR-MS). R. tinctorum roots were purchased from Okhra (Roussillon, France).
2.2 Preparation of lakes
2.2.1 Preparation of alizarin lake (molar ratio: alizarin/Al = 0.1)
The alizarin lake formulation was adapted from Sanyova and Reisse [8]. One hundred milliliters of an alizarin solution at 200 mg L−1 was stirred at room temperature and a hot alum solution (KAl(SO4)3·12H2O, 150 g L−1, pH = 2.9, 90 °C) was added. The solution was then stirred for 10 min at room temperature. A flocculation was observed, indicating the complexation between alizarin and Al3+. The mixture was neutralized to pH = 7 with a solution of Na2CO3 (0.1 M). The obtained lake was washed with deionized water, dried for 48 h at 50 °C, and ground. Before and after alizarin complexation, quantification by LC–PDA enabled the concentration of alizarin in the experimental lake to be determined.
2.2.2 Preparation of different madder lakes
Several madder lakes were prepared using different metal salts corresponding, respectively, to KAl(SO4)3 12H2O; CuSO4; SnCl2; ZnCl2; K2Cr2O7; FeCl3; and FeSO4. The same protocol was used for each of the lakes. Two grams of dry powder of madder roots was dispersed in 150 mL of deionized water and heated at 60 °C for 1 h under stirring. After filtration under vacuum, the solution of each salt at 90 °C was added. The solution was stirred for 10 min at 60 °C and neutralized to pH = 7 by adding a solution of Na2CO3 (0.1 M). The solution was filtered under vacuum by using a cellulose acetate filter (0.45 μm). The obtained metal-complex dyes were then washed with deionized water, dried, and ground.
2.3 Extraction procedures for alizarin lake analysis
Several protocols were used corresponding to HCl extraction and to ultrasonic or microwave methods.
- - For the HCl method, 2 mg of alizarin lake was suspended in 2 mL of a mixture solution containing HCl (37%)/methanol/water (2/1/1 v/v/v). This solution was heated to 100 °C for 10 min and cooled to ambient temperature.
- - For the ultrasonic method, ultrasound was applied using a PEX 3 Sonifer (R.E.U.S., Contes, France) (25 kHz, 150 W). The extraction time and temperature were, respectively, fixed at 10 min and 25 °C for all extractions.
- - For the method concerning microwave, a CEM Discover (C.E.M. Corporation, USA) reactor (45 MHz, 300 W) was used. It consisted of a pressurized Pyrex tube, which allows microwave to be applied at temperatures greater than the solvent boiling point.
For the ultrasonic and microwave methods, 2 mg of pulverized lake powder was extracted with 2 mL of extraction solvent and two acids were used: an acetic acid buffer solution (1 M) and a solution of oxalic acid (0.5 M) in methanol/water (1/1, v/v).
For all three methods, the pH was adjusted to 4 using KOH (4 M), filtered with a PE (polytetrafluoroethylene) cartridge filter (0.45 μm) in a 5 mL volumetric flask. The volume was adjusted to 5 mL using a solution of methanol/water (1/1, v/v). The obtained solution was filtered with a 0.45 μm PTFE cartridge filter and then directly injected in LC.
2.4 Liquid chromatographic analysis
The LC–PDA analyses were carried out using LC consisting of a quaternary pump, an in-line vacuum degasser, an autosampler, and a photodiode array detection system. The system was equipped with a biphenyl-column (Kinetex biphenyl, Phenomenex 2.6 μm, 4.6 × 150 mm), and controlled by Empower 2 software. The LC separation was performed at 35 °C with a binary elution mixture composed of high purity water (18.3 MΩ cm) containing 0.01% TFA (A) and acetonitrile (B). The chromatographic analysis was carried out for 8 min with a continuous flow rate of 1.4 mL min−1. The gradient program was as follows: 0–0.6 min, 25% B and 75% A; 0.6–1.50 min, 25–80% B and 75–20% A; 1.50–2.00 min, 80% B and 20% A; 2.00–2.50 min, 80–100% B and 20–0% A; 2.50–7.00 min, 100% B. All chromatograms were acquired at 450 nm and each sample was injected in triplicate.
3 Results and discussion
3.1 Analysis of alizarin lake
In the alizarin experimental lake prepared from alizarin standard compound, the amount of alizarin was optimized during the different lake-manufacturing steps to determine the amount of alizarin contained in the lakes. This lake was made with an alizarin/aluminum molar ratio of 0.1 and enabled an amount of 0.51 g of dry lake to be obtained. The amount of alizarin was quantified before and after the complexation to determine the alizarin content in the lake. The alizarin lake contained 172.24 mg of alizarin per gram of lake corresponding to around 17% of alizarin in the experimental lake in accordance with Sanyova [21]. A total of five different methods were tested to compare their effectiveness in extracting coloring molecules from the coordination complex. The first protocol was the reference method with HCl (37%)/MeOH/H2O (2/1/1, v/v/v) [7] to extract madder dyes from lake pigments, in ancient textiles or archaeological objects [8,9,22–25]. This process was frequently used but it was accepted that this particular extraction induces the hydrolysis of glycosidic precursors into their respective aglycones [7,26]. To preserve the glycosidic chemical population, a mild method using acetic acid was developed. After decomplexation, nondenaturing methods (without degradation of heterosides) using oxalic acid solution (pH around 1) needed alkalization to preserve the chromatographic column. The pH was adjusted to 4 so as to maintain the acidic condition. In fact, in its basic condition, Al(OH)3 is the most predominant species and a coprecipitate of dye–metal complexes with Al(OH)3 may be formed [11,27,28].
Chromatographic analyses were performed using a Kinetex biphenyl column with homogeneous core–shell particles enabling an increase in the flow to occur with an acceptable level of back pressure while maintaining high resolution and sensitivity. This method reduced the analysis time to 8 min and the amount of solvent used.
Table 2 shows that strong acidic extraction by HCl allowed 31.23% of alizarin contained in the experimental lake to be extracted. The use of acetic acid buffer, a nondestructive method, enabled 19.21% under ultrasound to be extracted [11] but the use of microwave increased the decomplexation yield to 44.83%. Microwave improved molecular stirring and solvent temperature, which, in turn, promoted decomplexation. Oxalic acid solution (0.5 M) in MeOH/H2O (1/1, v/v) increased the decomplexation yield to 53.4% of recovered alizarin using ultrasound. For both acetic acid buffer and oxalic acid solution the microwave method improved the decomplexation yield. The most efficient method was to use oxalic acid solution with microwave giving a yield of 71.94% of recovered alizarin from alizarin lake. Furthermore, at 60 °C the use of oxalic acid did not hydrolyze glycosidic precursors.
Decomplexation yield of alizarin by five different methods.
Process | Solvent | Decomplexation yield (%) |
Heating 10 min at 100 °C | HCl (37%)/H2O/MeOH (2/1/1) | 31.23 ± 2.23 |
Ultrasound | Acetic acid buffer solution (1 M) | 19.21 ± 0.27 |
Ultrasound | Oxalic acid solution | 53.4 ± 1.78 |
Microwave | Acetic acid buffer solution (1 M) | 44.83 ± 3.5 |
Microwave | Oxalic acid solution | 71.94 ± 1.86 |
3.2 Comparison of mild extraction methods
All of the experimental lakes were realized from the same homogeneous sample of madder root powder.
To compare the effect of each extraction method, the madder roots that were used to prepare the various lakes were analyzed beforehand. The roots were macerated in water at 60 °C. The obtained chromatogram is presented in Fig. 1. The analyzed extract showed the presence of heterosidic and aglycone compounds corresponding, respectively, by elution time to lucidin primeveroside (1), ruberythric acid (2), unk 3.8 (3), rubiadin-3-O-primeverose (4), anthragallol (5), lucidin (6), alizarin (7), purpurin (8), rubiadin (9), and nordamnacanthal (10). The large unknown peak eluted at 3.8 min (unk 3.8) can be attributed to a coeluted mixture of pseudopurpurin and munjistin, two carboxylic acids, which are difficult to separate using this type of chromatographic column. The efficiency of the four nondenaturing protocols for the extraction of dyes from different madder lakes was compared. Six organic salts were used to make madder lakes (KAl(SO4)3·12H2O; CuSO4; SnCl2; ZnCl2; K2Cr2O7; FeCl3; and FeSO4). They were decomplexed by using two processes (ultrasound and microwave) and two solvents (acetic acid buffer solution and oxalic acid solution in methanol). After the different extraction processes, a relative quantification was carried out by measuring the peak areas of each LC–PDA chromatogram (Fig. 2).
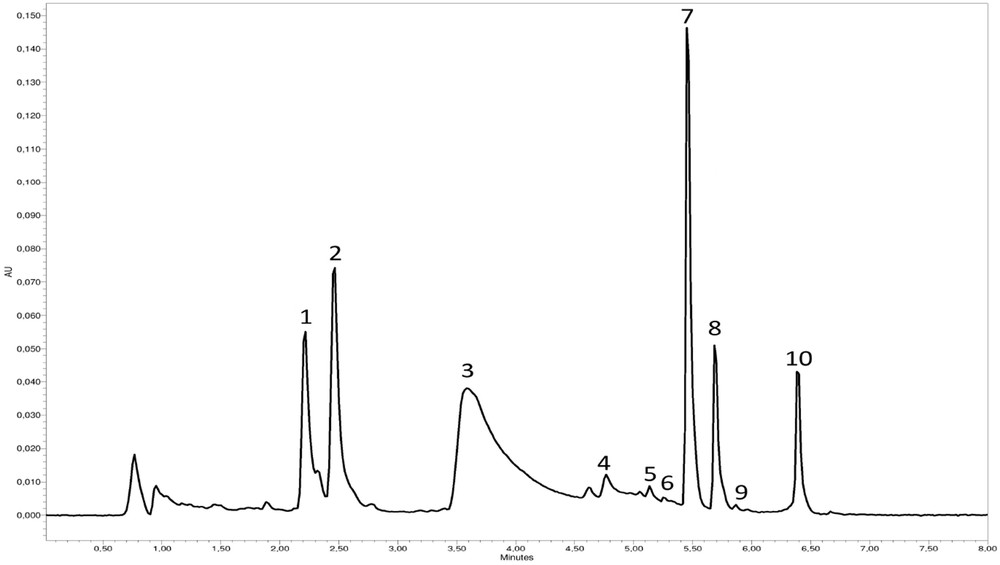
Chromatogram at 450 nm of madder extract before complexation. 1, lucidin primeveroside; 2, ruberythric acid; 3, unk 3.8; 4, rubiadin-3-O-primeverose; 5, anthragallol; 6, lucidin; 7, alizarin; 8, purpurin; 9, rubiadin; 10, nordamnacanthal.
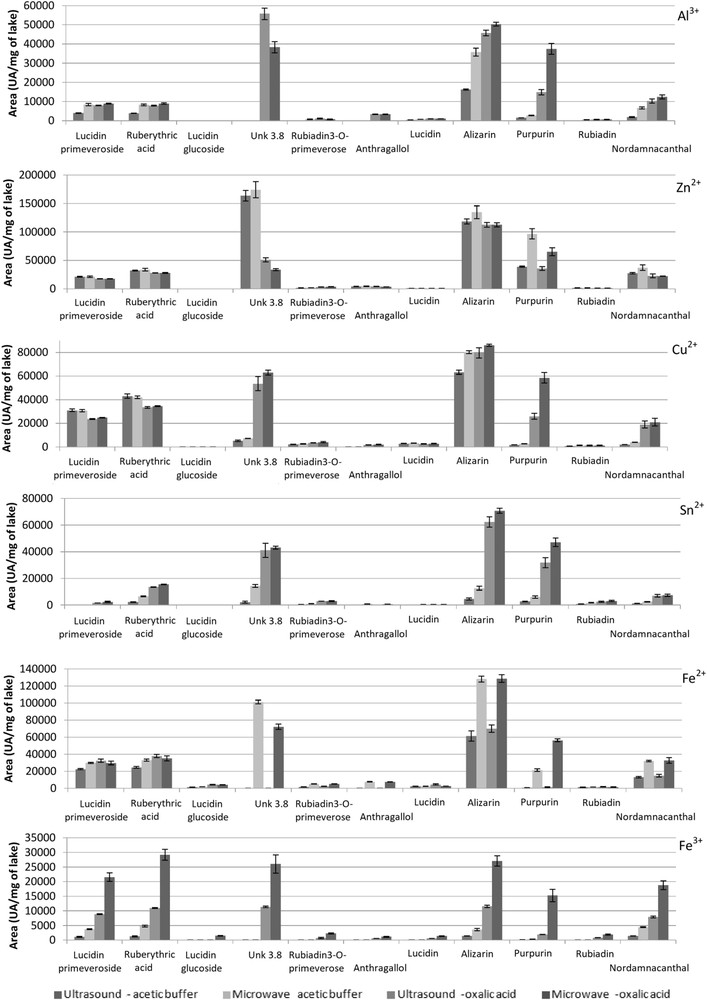
LC peak areas measured for each compound per milligram of lake for the four methods: ultrasound using acetic acid or oxalic acid and microwave using acetic acid or oxalic acid.
Aluminum lake extraction with acetic acid and ultrasound preserved and extracted lucidin primeveroside and ruberythric acid, and extracted lucidin, alizarin, purpurin, and nordamnacanthal. Microwave enabled two other compounds, rubiadin-3-O-primeveroside and rubiadin, to be recovered. Oxalic acid increased the decomplexation of all dyes and of two other compounds: unk 3.8 and anthragallol. For alizarin and purpurin, extractions increased from 27% to 3%, respectively, between acetic acid extractions under ultrasound to the maximal recovery areas for oxalic acid under microwave (Fig. 2). The microwave process increased extractions of all compounds, unlike ultrasound, except for the unknown compound with oxalic acid. Decomplexation of dyes from zinc lake was quite similar to lucidin primeveroside and ruberythric acid when applying the four methods. Alizarin extraction was better with acetic acid buffer solution and the microwave process compared with oxalic acid solution decomplexations. More purpurin was extracted with the microwave process than with ultrasound and especially with oxalic acid solution (Fig. 2). Acetic acid with the microwave process appeared to be the most suitable solution to decomplex zinc lake. For copper lake, the extraction of dyes was characterized by a better decomplexation with oxalic acid for alizarin, purpurin, rubiadin nordamnacanthal, and other compounds, except for lucidin primeveroside and ruberythric acid whose decomplexation was slightly better in comparison with processes using acetic buffer solution. The oxalic solution enabled molecules of anthragallol, which was not recovered with acetic acid buffer solution to be detected (Fig. 2). The latter solution was not efficient in decomplexing tin lake, as opposed to oxalic acid solution, a solution which presented better recovery of different heterosidic and aglycone dyes. The microwave process provided a slight advantage for oxalic acid decomplexation including alizarin and purpurin (Fig. 2).
Two iron lakes were made, respectively, with iron(II) (iron sulfate) and iron(III) (iron chloride). It was easier to decomplex dyes from iron(II) lake than from iron(III) lake. In fact, iron(II) lake extraction of dyes provided a better decomplexation yield in comparison with iron(III) lake. Concerning the Fe(II) experimental lake, acetic acid solution had similar results on the extractions of alizarin, purpurin, and nordamnacanthal, and it was likewise with both the ultrasound and microwave processes. Microwave extraction was better for heterosidic compounds. Oxalic acid solution allowed unk 3.8 and anthragallol compounds to be extracted. It also increased the recovery of all dyes.
Iron(III) lake was poorly extracted using acetic acid buffer solution. Only lucidin primeveroside, ruberythric acid, alizarin purpurin, and nordamnacanthal were detected. Oxalic acid enabled rubiadin-3-O-primeveroside, anthragallol, lucidin, rubiadin, and unk 3.8 to be obtained under ultrasound and a better yield to be extracted with microwave. More alizarin was extracted with microwave and oxalic acid solution than with acetic acid buffer solution associated with ultrasound.
Oxalic acid (0.5 M) in methanol/water solution (1/1, v/v) resulted in an increase in the decomplexation of metal-complex dyes. Acetic acid buffer presented a lower extraction of purpurin in all lakes except in zinc madder lake. Oxalic acid enabled a significant number of anthraquinones, which had not been detected to be identified using the acetic acid buffer solution. Microwave increased decomplexation of dyes in most cases. The decomplexation process using microwave and oxalic acid solution could be a universal and nondenaturing method. No chemical degradation of the native population of anthraquinones was observed in the previously mentioned conditions.
4 Conclusions
Alizarin lake was used as a model to compare the efficacy of hydrochloric acid, acetic acid, and oxalic acid solutions to extract alizarin from the metal-complex dye. The use of ultrasound and microwave increased the extraction yield of alizarin when compared with the reference method of decomplexation using a mixture of HCl (37%)/methanol/water (2/1/1, v/v/v). Among the different protocols applied in this research study oxalic acid solution (0.5 M in methanol/water (1/1 v/v)) using microwave was the most efficient and universal technique to extract glycone and aglycone anthraquinones from several madder lakes. The universal technique in this case signifies the capacity to extract dyes irrespective of the nature of the mineral salts used in the lake formulation. Further analyses are required to test this method for decomplexing dyes in other matrix such as dyed textiles.
Acknowledgments
This research was financially supported by PACA Regional Council and the Erubescence Company (Avignon, France).