1 Introduction
The progressive and devastating cognitive decline of Alzheimer's disease (AD) described by Alzheimer a century ago [1] is related to the abnormal deposition of two proteins, namely amyloid protein (Aβ) and hyperphosphorylated Tau protein. Current AD therapy is based on four acetylcholine esterase inhibitors and memantine, a weak antagonist of the N-methyl-d-aspartate receptor, that are not really effective, and the pipeline of new drugs is rather unproductive [2]. Therefore, it is essential to explore new efficient drugs for AD, which can slow down or stop the AD loss of memory at the early phase. There is extensive evidence linking metal disregulation to Tau protein pathology and Aβ-aggregation in AD brain [3–5]. Copper ions are concentrated in AD amyloid plaques, as high as 400 μM, close to six times higher than those in normal brain [6]. The activation of dioxygen by copper–amyloid complexes, in the presence of endogenous reductants, induces the catalytic generation of reactive oxygen species [7–10]. The bioinorganic pharmacology of AD should therefore be studied as a potential drug target. Few specific ligands able to restore the homeostasis of copper have been considered as potential drugs for the treatment of AD [11–15]. These copper chelators are expected to extract copper ions from copper–amyloid complexes and translate them to copper-carrier proteins or copper enzymes inside the brain. Barnham and Bush [12] developed two hydroxyquinoline derivatives, namely clioquinol (CQ) and PBT2. Unfortunately, CQ and PBT2 are unable to efficiently extract copper from Cu–Aβ, and they generate ternary Cu–Aβ–CQ or Cu–Aβ–PBT2 complexes that retain a damaging redox activity [16]. On the basis of our previous work on bis-8-aminoquinolines [17,18], we have designed a series of mono-8-aminoquinoline derivatives with a bidentate amine side chain, named TDMQs. When the length of the side chain is suitable, TDMQs offer a N4-tetradentate square planar coordination sphere that is able to specifically chelate copper (II) in complexes having a TDMQ/Cu = 1/1 stoichiometry and, therefore, to extract this metal ion from the copper–amyloid [19]complex. Copper is then released from the Cu–TDMQ complex in the presence of glutathione, which is a physiological provider of copper for copper-carrier proteins [19], without interacting with zinc, which is abundant in the brain [20], but prefers a tetrahedral coordination. To document the structure–selectivity relationship in the series of TDMQ ligands retaining the already characterized N4-tetradentate square planar coordination sphere and to enlarge the scope of these putative drugs, we report here the synthesis and characterization of new derivatives bearing electron donating groups [O–CH3 or N(CH3)2] at several positions of the quinoline nucleus. The chelation of copper (II) by these ligands and their selectivity with respect to zinc are also reported and compared with those of the previously reported TDMQ chelators.
2 Materials and methods
2.1 General methods
Chemical reagents were purchased from common suppliers and used as received. Reactions were monitored by thin-layer chromatography performed with 0.2 mm silica gel 60F plates and visualized by ultraviolet (UV) light. Purification of products by column chromatography was carried out on silica gel (300–400 mesh). 1H NMR and 13C NMR spectra were recorded using a Bruker AVANCE III 400 MHz spectrometer (400.0 MHz for 1H NMR and 100.6 MHz for 13C NMR) and a Bruker AVANCE III 500 MHz spectrometer (500.0 MHz for 1H NMR and 125.7 MHz for 13C NMR). Tetramethylsilane was used as an external standard.
2.2 Syntheses
2.2.1 Synthesis of TDMQ31
The structures of TDMQ ligands are presented in Scheme 1 R5 = R7 = H, R6 = OCH3).

Structures of TDMQ ligands having different electron withdrawing substituents on the quinoline ring. The previously reported TDMQ20 [11] is given for comparison.
2.2.1.1 6-Methoxy-2-methyl-8-nitroquinoline (D-TDMQ31)
To a solution of 4-methoxy-2-nitroaniline (C-TDMQ31, Scheme 2, R6 = OCH3) in HCl (12 M, 10 mL) at 0 °C, acetaldehyde was added dropwise under stirring. The reaction medium was kept at 0 °C for 15 min, gradually increased to 80 °C, and then stirred at 80 °C for 1 h. The resulting mixture was poured into ice-cold water and neutralized with aqueous ammonium hydroxide. After extraction with CH2Cl2, the organic phase was dried over MgSO4, filtered, and concentrated under reduced pressure. The crude mixture was purified by silica gel flash column chromatography (ethyl acetate/hexane, 1/20, v/v). After evaporation of the eluent, D-TDMQ31 was obtained as a yellow solid (41%). 1H NMR (CDCl3): δ = 7.99 (d, J = 8.6 Hz, 1H), 7.62 (d, J = 2.8 Hz, 1H), 7.35 (d, J = 8.6 Hz, 1H), 7.24 (d, J = 2.8 Hz, 1H), 3.96 (s, 3H), 2.72 ppm (s, 3H).
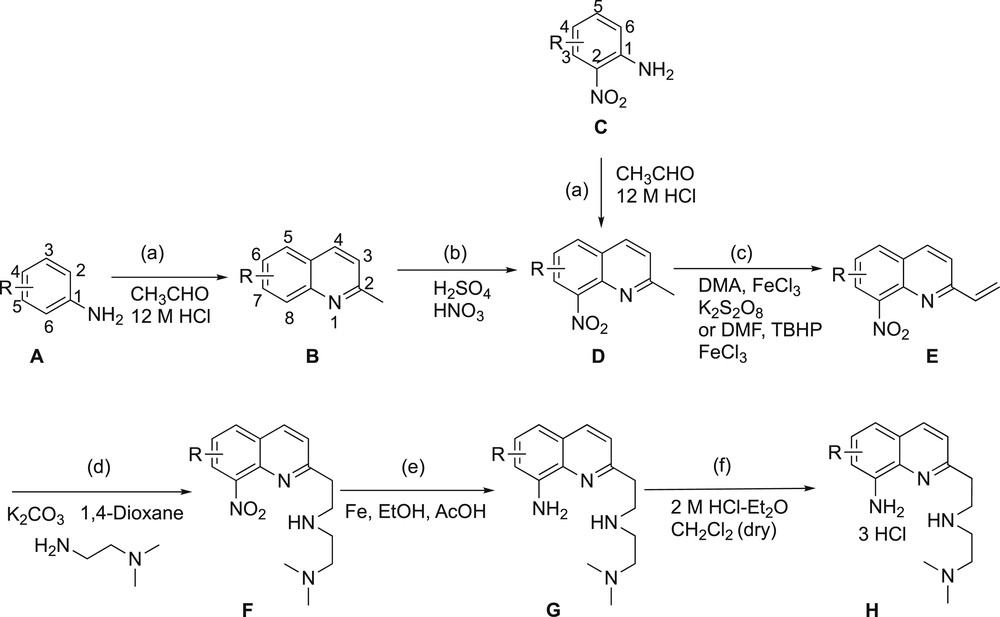
Synthesis of TDMQ31, TDMQ32, TDMQ33, TDMQ38, and TDMQ39. (a) CH3CHO/12 M HCl, 80 °C, 1 h; (b) HNO3/H2SO4, rt, 1 h; (c) FeCl3, K2S2O8, DMA, 110 °C, 30 min for TDMQ31; DMF, TBHP, FeCl3, 140 °C, 3 h for TDMQ32, TDMQ33, and TDMQ39, and 5 h for TDMQ38; (d) NH2(CH2)2N(CH3)2, K2CO3, 1,4-dioxane, 12 h for TDMQ31, and 1 h for TDMQ32; Cs2CO3, 48 h for TDMQ33, TDMQ38, and TDMQ39; (e) Fe, C2H5OH, CH3COOH, 80 °C, 1 h; (f) HCl 2 M in Et2O, CH2Cl2, 1 h.
2.2.1.2 6-Methoxy-8-nitro-2-vinylquinoline (E-TDMQ31)
To a solution of D-TDMQ31 (Scheme 2, R5 = R7 = H, R6 = OCH3) in N,N-dimethylacetamide (5 mL) were added FeCl3 and K2S2O8. The mixture was stirred at 110 °C for 30 min and quenched with water. After extraction with CH2Cl2, the organic phase was dried over MgSO4, filtered, and concentrated under reduced pressure. The crude mixture was purified by silica gel flash column chromatography (hexane/dichloromethane, 50/1, v/v). After evaporation of the eluent, compound E-TDMQ31 was obtained as a red solid (17%). 1H NMR (CDCl3): δ = 8.05 (d, J = 8.8 Hz, 1H), 7.64 (d, J = 2.8 Hz, 1H), 7.61 (d, J = 8.8 Hz, 1H), 7.24 (d, J = 2.8 Hz, 1H), 7.00–6.93 (m, 1H), 6.34 (d, J = 17.6 Hz, 1H), 5.65 (d, J = 10.8 Hz, 1H), 3.97 ppm (s, 3H).
2.2.1.3 N1-(2-(6-Methoxy-8-nitroquinolin-2-yl)ethyl)-N3,N3-dimethylpropane-1,3-diamine (F-TDMQ31)
To a mixture of E-TDMQ31 and K2CO3 in 1,4-dioxane (10 mL) was added N,N-dimethyl-1,2-ethanediamine. The reaction mixture was stirred at room temperature for 12 h and quenched with water. After extraction with CH2Cl2, the organic phase was dried over MgSO4, filtered, and concentrated under reduced pressure. The crude mixture was purified by silica gel flash column chromatography (ethyl acetate/isopropanol/25% ammonium hydroxide, 5/1/0.2, v/v/v). After evaporation of the eluent, F-TDMQ31 was obtained as a brown solid (21%). 1H NMR (CDCl3): δ = 8.01 (d, J = 8.6 Hz, 1H, H4), 7.64 (d, J = 2.6 Hz, 1H), 7.36 (d, J = 8.6 Hz, 1H), 7.24 (d, J = 2.6 Hz, 1H), 3.96 (s, 3H), 3.18–3.12 (m, 4H), 2.77 (t, J = 6.6 Hz, 2H), 2.45 (t, J = 6.6 Hz, 2H), 2.22 ppm (s, 6H).
2.2.1.4 N1-(2-(8-Amino-6-methoxyquinolin-2-yl)ethyl)-N3,N3-dimethylpropane-1,3-diamine (G-TDMQ31)
Iron (131.6 mg, 2.36 mmol) and acetic acid (3 mL) were added to a solution of F-TDMQ31 in ethanol (9 mL). The mixture was stirred at 80 °C under reflux for 1 h. The reaction mixture was poured into an aqueous saturated NaCl solution (200 mL) and extracted with CH2Cl2 (3 × 100 mL). The combined organic layers were dried over MgSO4, filtered, and concentrated under reduced pressure. The crude product was purified by silica gel flash chromatography (ethyl acetate/hexane, 1/10, v/v). Evaporation of the solvent afforded G-TDMQ31, which was obtained as a yellow oil (70%). 1H NMR (CDCl3): δ = 7.86 (d, J = 6.8 Hz, 1H), 7.21 (d, J = 6.8 Hz, 1H), 6.55 (d, J = 2.0 Hz, 1H), 6.45 (d, J = 2.0 Hz, 1H), 5.02 (s, 2H), 3.86 (s, 3H), 3.11 (s, 4H), 2.76 (t, J = 4.8 Hz, 2H), 2.43 (t, J = 4.8 Hz, 2H), 2.22 ppm (s, 6H).
2.2.1.5 TDMQ31
To a solution of G-TDMQ31 in dry dichloromethane was added excess hydrogen chloride solution (2.0 M in diethyl ether). The precipitate was filtered, washed with diethyl ether, and dried under reduced pressure to give TDMQ31, which was obtained as a yellow solid (96%). 1H NMR (DMSO-d6): δ = 9.58 (br s, 2H, CH2–NH2+–CH2), 8.26 (d, J = 8.4 Hz, 1H, H4), 7.52 (d, J = 8.4 Hz, 1H, H3), 7.14 (s, 1H, H5), 7.07 (s, 1H, H7), 3.87 (s, 3H, C6–OCH3), 3.62 (t, J = 6.4 Hz, 2H, C2–CH2–CH2), 3.59 [m, 4H, NH2+–CH2–CH2–N+H(CH3)2], 3.45 (t, J = 6.4 Hz, 2H, C2–CH2), 2.86 ppm [s, 6H, NH+(CH3)2]; 13C NMR (100 MHz, DMSO-d6): δ = 158.3 (C6), 155.4 (C2), 144.7 (C8), 135.2 (C4), 134.7 (C8a), 128.1 (C4a), 122.4 (C3), 101.5 (C7), 94.5 (C5), 59.1 [CH2–NH+(CH3)2], 55.2 (C6–OCH3), 49.1 (C2–CH2–CH2), 47.2 (NH+(CH3)2), 45.6 (NH2+–CH2–CH2), 38.1 ppm (C2–CH2); mp 153 °C; IR (KBr): ν = 804, 865, 1027, 1166, 1199, 1265, 1340, 1396, 1471, 1504, 1608, 1650, 2948, 2977, 3236, 3401, 3465 cm−1; HRMS (ESI) calcd for C16H25ON4: 289.20235 ([M + H]+), found: 289.20229; elemental analysis for C16H24ON4·3.0HCl·1.9·0.4C2H5OC2H5·0.2CH2Cl2 (apparent MW = 471.5444): calcd C, 45.34; H, 7.52; N, 11.88; found C, 45.34; H, 7.40; N, 11.75.
2.2.2 Synthesis of TDMQ32
2.2.2.1 5-Methoxy-2-methyl-8-nitroquinoline (D-TDMQ32)
The procedure was similar to that described for the preparation of D-TDMQ31, but starting from 5-methoxy-2-nitroaniline (C-TDMQ32, Scheme 1, R5 = OCH3). D-TDMQ32 was obtained as a yellow solid (70%). 1H NMR (CDCl3): δ = 8.48 (d, J = 8.6 Hz, 1H), 8.01 (d, J = 8.6 Hz, 1H), 7.37 (d, J = 8.6 Hz, 1H), 6.78 (d, J = 8.6 Hz, 1H), 4.07 (s, 3H), 2.77 ppm (s, 3H).
2.2.2.2 5-Methoxy-8-nitro-2-vinylquinoline (E-TDMQ32)
To a solution of D-TDMQ32 (Scheme 2, R6 = R7 = H, R5 = OCH3) in N,N-dimethylformamide (5 mL) were added FeCl3 (37.2 mg, 0.23 mmol) and TBHP (660 μL). The mixture was stirred at 140 °C for 3 h and quenched with water. After extraction with CH2Cl2, the organic phase was dried over MgSO4, filtered, and concentrated under reduced pressure. The crude mixture was purified by silica gel flash column chromatography (hexane/dichloromethane, 10/1, v/v). After evaporation of the eluent, compound E-TDMQ32 was obtained as a yellow solid (50%). 1H NMR (CDCl3): δ = 8.41 (d, J = 8.6 Hz, 1H, H4), 8.01 (d, J = 8.6 Hz, 1H), 7.48 (d, J = 8.6 Hz, 1H), 6.94–6.87 (m, 1H), 6.66 (d, J = 8.6 Hz, 1H), 6.36 (d, J = 17.6 Hz, 1H), 5.62 (d, J = 10.8 Hz, 1H), 3.96 ppm (s, 3H).
2.2.2.3 N1-(2-(5-Methoxy-8-nitroquinolin-2-yl)ethyl)-N3,N3-dimethylpropane-1,3-diamine (F-TDMQ32)
The procedure was similar to that described for the preparation of F-TDMQ31, but starting from E-TDMQ32 (Scheme 2, R6 = R7 = H, R5 = OCH3) and the reaction time was 1 h. F-TDMQ32 was obtained as a yellow solid (65%). 1H NMR (CDCl3): δ = 8.48 (d, J = 8.8 Hz, 1H), 8.12 (d, J = 8.4 Hz, 1H), 7.38 (d, J = 8.8 Hz, 1H), 6.78 (d, J = 8.4 Hz, 1H), 4.07 (s, 3H), 3.20–3.15 (m, 4H), 2.79 (t, J = 6.4 Hz, 2H), 2.46 (t, J = 6.4 Hz, 2H), 2.22 ppm (s, 6H).
2.2.2.4 N1-(2-(8-Amino-5-methoxyquinolin-2-yl)ethyl)-N3,N3-dimethylpropane-1,3-diamine (G-TDMQ32)
The procedure was similar to that described for the preparation of G-TDMQ31, but starting from F-TDMQ32 (Scheme 2, R6 = R7 = H, R5 = OCH3). G-TDMQ32 was obtained as a yellow solid (60%). 1H NMR (CDCl3): δ = 8.40 (d, J = 8.4 Hz, 1H), 7.26 (d, J = 8.4 Hz, 1H), 6.84 (d, J = 8.0 Hz, 1H), 6.68 (d, J = 8.0 Hz, 1H), 3.92 (s, 3H), 3.19–3.18 (m, 4H), 2.80 (t, J = 6.0 Hz, 2H), 2.46 (t, J = 6.0 Hz, 2H), 2.21 ppm (s, 6H).
2.2.2.5 TDMQ32
To a solution of G-TDMQ32 in dry dichloromethane was added an excess hydrogen chloride solution (2.0 M in diethyl ether). The precipitate was filtered, washed with diethyl ether, and dried under reduced pressure to give TDMQ32, which was obtained as a yellow solid (99%). 1H NMR (D2O): δ = 8.61 (d, J = 8.6 Hz, 1H, H4), 7.69 (d, J = 8.2 Hz, 1H, H7), 7.53 (d, J = 8.6 Hz, 1H, H3), 7.02 (d, J = 8.2 Hz, 1H, H6), 4.00 (s, 3H, OCH3), 3.74 (t, J = 6.8 Hz, 2H, C2–CH2–CH2–NH2+), 3.58–3.54 [m, 4H, NH2+–CH2–CH2–NH+(CH3)2], 3.45 (t, J = 6.8 Hz, 2H, C2–CH2), 2.93 ppm [s, 6H, N(CH3)2]; mp 146 °C; IR (KBr): ν = 827, 1020, 1085, 1194, 1272, 1411, 1483, 1602, 1645, 2638, 2746, 2873, 2975, 3450 cm−1; HRMS (ESI) calcd for C16H25ON4: 289.20251 ([M + H]+), found: 289.20229; elemental analysis for C16H24ON4·3.0HCl·2.5H2O·0.2C2H5OC2H5·0.15CH2Cl2 (apparent MW = 470.3732): calcd C, 43.28; H, 7.35; N, 11.91; found C, 43.30; H, 7.04; N, 11.89.
2.2.3 Synthesis of TDMQ33
2.2.3.1 7-Methoxy-2-methylquinoline (B-TDMQ33)
The procedure was similar to that described for the preparation of D-TDMQ31, but starting from 3-methoxyaniline (A-TDMQ33, Scheme 2, R3 = OCH3). B-TDMQ33 was obtained as a yellow oil (36%). 1H NMR (CDCl3): δ = 7.93 (d, J = 8.6 Hz, 1H), 7.62 (d, J = 8.6 Hz, 1H), 7.36 (s, 1H), 7.13 (d, J = 8.0 Hz, 1H), 7.11 (d, J = 8.0 Hz, 1H), 3.92 (s, 3H), 2.70 ppm (s, 3H).
2.2.3.2 7-Methoxy-2-methyl-8-nitroquinoline (D-TDMQ33)
To a stirred solution of B-TDMQ33 in neat sulfuric acid (10.0 mL) was added fuming nitric acid (5.0 mL) dropwise over a period of 1 h at an ambient temperature. The resulting mixture was stirred for an additional hour and was then poured into ice. The mixture was allowed to warm to ambient temperature and neutralized with aqueous ammonium hydroxide. After extraction with CH2Cl2, the organic phase was dried over MgSO4, filtered, and concentrated under reduced pressure. The crude mixture was purified by silica gel flash column chromatography (ethyl acetate/hexane, 1/30, v/v). Evaporation of the solvent afforded D-TDMQ33 as a yellow solid (32%). 1H NMR (CDCl3): δ = 7.90 (d, J = 8.4 Hz, 1H), 7.75 (d, J = 9.0 Hz, 1H), 7.22 (d, J = 9.0 Hz, 1H), 7.14 (d, J = 8.4 Hz, 1H), 3.94 (s, 3H), 2.60 ppm (s, 3H).
2.2.3.3 7-Methoxy-8-nitro-2-vinylquinoline (E-TDMQ33)
The procedure was similar to that described for the preparation of E-TDMQ32, but starting from D-TDMQ33 (Scheme 2, R6 = R5 = H, R7 = OCH3). E-TDMQ33 was obtained as a yellow solid (34%). 1H NMR (500.0 MHz, CDCl3): δ = 8.45 (d, J = 8.5 Hz, 1H), 8.20 (d, J = 9.0 Hz, 1H), 7.81 (d, J = 8.5 Hz, 1H), 7.74 (d, J = 9.0 Hz, 1H), 6.97–6.91 (m, 1H), 6.43 (d, J = 17.5 Hz, 1H), 5.75 (d, J = 10.5 Hz, 1H), 4.08 ppm (s, 3H).
2.2.3.4 N1-(2-(7-Methoxy-8-nitroquinolin-2-yl)ethyl)-N3,N3-dimethylpropane-1,3-diamine (F-TDMQ33)
The procedure was similar to that described for the preparation of F-TDMQ31, but starting from E-TDMQ33 (Scheme 2, R6 = R5 = H, R7 = OCH3). The catalyst was replaced by Cs2CO3 and the reaction time was 48 h. F-TDMQ33 was obtained as a yellow oil (75%). 1H NMR (500.0 MHz, DMSO-d6): δ = 8.40 (d, J = 8.5 Hz, 1H), 8.21 (d, J = 9.0 Hz, 1H), 7.73 (d, J = 9.0 Hz, 1H), 7.50 (d, J = 8.5 Hz, 1H), 4.07 (s, 3H), 3.13 (t, J = 7.0 Hz, 2H), 3.04 (t, J = 7.0 Hz, 2H), 2.73 (t, J = 6.0 Hz, 2H), 2.38 (t, J = 6.0 Hz, 2H), 2.14 ppm (s, 6H).
2.2.3.5 N1-(2-(8-Amino-7-methoxyquinolin-2-yl)ethyl)-N3,N3-dimethylpropane-1,3-diamine (G-TDMQ33)
The procedure was similar to that described for the preparation of G-TDMQ31, but starting from F-TDMQ33 (Scheme 2, R6 = R5 = H, R7 = OCH3). G-TDMQ33 was obtained as a yellow solid (80%). 1H NMR (500.0 MHz, CDCl3): δ = 7.92 (d, J = 8.4 Hz, 1H), 7.20 (d, J = 8.4 Hz, 1H), 7.12 (s, 1H), 7.10 (s, 1H), 4.96 (s, 2H, C8–NH2), 3.98 (s, 3H), 3.14 (s, 4H), 2.76 (t, J = 6.2 Hz, 2H), 2.43 (t, J = 6.2 Hz, 2H), 2.21 ppm (s, 6H).
2.2.3.6 TDMQ33
To a solution of G-TDMQ33 in dry dichloromethane was added an excess of hydrogen chloride solution (2.0 M in diethyl ether). The precipitate was filtered, washed with diethyl ether, and dried under reduced pressure to give TDMQ33 as a yellow solid (99%). 1H NMR (400.0 MHz, D2O): δ = 8.33 (d, J = 8.4 Hz, 1H, H4), 7.92 (d, J = 9.2 Hz, 1H, H5), 7.57 (d, J = 9.2 Hz, 1H, H6), 7.41 (d, J = 8.4 Hz, 1H, H3), 4.05 (s, 3H, OCH3), 3.72 (t, J = 6.8 Hz, 2H, C2–CH2–CH2), 3.56–3.52 [m, 4H, NH2+–CH2–CH2–NH+(CH3)2], 3.44 (t, J = 6.8 Hz, 2H, C2–CH2), 2.92 ppm [s, 6H, NH+(CH3)2]; mp 97 °C; IR (KBr): ν = 786, 848, 1002, 1045, 1147, 1268, 1456, 1513, 1625, 1643, 2642, 2750, 2869, 2956, 3083, 3436 cm−1; HRMS (ESI) calcd for C16H25ON4: 289.20249 ([M + H]+), found: 289.20229; elemental analysis for C16H24ON4·3.0HCl·1.8H2O·0.3C2H5OC2H5·0.02CH2Cl2 (apparent MW = 454.1344): calcd C, 45.54; H, 7.47; N, 12.34; found C, 45.53; H, 7.56; N, 12.63.
2.2.4 Synthesis of TDMQ38
2.2.4.1 N1,N1-Dimethyl-2-nitrobenzene-1,3-diamine (C-TDMQ38)
To a solution of 3-chloro-2-nitroaniline in dry N,N-dimethylformamide were added K2CO3 and dimethylamine. The mixture was stirred at 140 °C for 12 h and quenched with water. After extraction with CH2Cl2, the organic phase was dried over MgSO4, filtered, and concentrated under reduced pressure. The crude mixture was purified by silica gel flash column chromatography (hexane/ethyl acetate, 10/1, v/v). After evaporation of the eluent, compound C-TDMQ38 was obtained as a yellow solid (99%). 1H NMR (CDCl3): δ = 7.07 (dd, J = 8.8 Hz, 1H), 6.25 (d, J = 8.8 Hz, 1H), 6.18 (d, J = 8.8 Hz, 1H), 5.0 (s, 2H), 2.84 ppm (s, 6H).
2.2.4.2 N,N,2-Trimethyl-8-nitroquinolin-7-amine (D-TDMQ38)
The procedure was similar to that described for the preparation of D-TDMQ31, but starting from C-TDMQ38. D-TDMQ38 was obtained as a yellow solid (54%). 1H NMR (CDCl3): δ = 7.88 (d, J = 8.4 Hz, 1H), 7.66 (d, J = 9.2 Hz, 1H), 7.18 (d, J = 9.2 Hz, 1H), 7.13 (d, J = 8.4 Hz, 1H), 3.05 (s, 6H), 2.66 ppm (s, 3H).
2.2.4.3 N,N-Dimethyl-8-nitro-2-vinylquinolin-7-amine (E-TDMQ38)
The procedure was similar to that described for the preparation of E-TDMQ32, but starting from D-TDMQ38 and the reaction time was 5 h. E-TDMQ38 was obtained as a yellow solid (22%). 1H NMR (CDCl3): δ = 7.89 (d, J = 8.4 Hz, 1H, H6), 7.60 (d, J = 9.2 Hz, 1H), 7.31 (d, J = 8.4 Hz, 1H), 7.13 (d, J = 9.2 Hz, 1H), 6.86 (dd, J = 10.8, 17.6 Hz, 1H), 6.27 (d, J = 17.6 Hz, 1H), 5.54 (d, J = 10.8 Hz, 1H), 3.01 ppm (s, 6H).
2.2.4.4 N1-(2-(7-(Dimethylamino)-8-nitroquinolin-2-yl)ethyl)-N2,N2-dimethylethane-1,2-diamine (F-TDMQ38)
The procedure was similar to that described for the preparation of F-TDMQ33, but starting from E-TDMQ32. F-TDMQ38 was obtained as a yellow oil (70%). 1H NMR (CDCl3): δ = 7.91 (d, J = 8.4 Hz, 1H), 7.67 (d, J = 9.2 Hz, 1H), 7.20 (d, J = 9.2 Hz, 1H), 7.15 (d, J = 8.4 Hz, 1H), 3.12–3.09 (m, 4H), 3.07 (s, 6H), 2.76 (t, J = 6.6 Hz, 2H), 2.45 (t, J = 6.6 Hz, 2H), 2.23 ppm (s, 6H).
2.2.4.5 2-(2-((2-(Dimethylamino)ethyl)amino)ethyl)-N7,N7-dimethylquinoline-7,8-diamine (G-TDMQ38)
The procedure was similar to that described for the preparation of G-TDMQ31, but starting from F-TDMQ38. G-TDMQ38 was obtained as a yellow solid (60%). 1H NMR (CDCl3): δ = 7.96 (d, J = 8.4 Hz, 1H), 7.34 (d, J = 8.8 Hz, 1H), 7.18 (d, J = 8.4 Hz, 1H), 7.13 (d, J = 8.8 Hz, 1H), 5.23 (s, 2H, C8–NH2), 3.26 (m, 4H), 2.90 (t, J = 5.8 Hz, 2H), 2.80 (s, 6H), 2.55 (t, J = 5.8 Hz, 2H), 2.26 ppm (s, 6H).
2.2.4.6 TDMQ38
To a solution of G-TDMQ38 in dry dichloromethane was added an excess of hydrogen chloride solution (2.0 M in diethyl ether). The precipitate was filtered, washed with diethyl ether, and dried under reduced pressure to give TDMQ38 as a yellow solid (99%). 1H NMR (D2O): δ = 8.26 (d, J = 8.6 Hz, 1H, H6), 7.64 (d, J = 9.0 Hz, 1H, H4), 7.54 (d, J = 8.6 Hz, 1H, H5), 7.48 (d, J = 9.0 Hz, 1H, H3), 3.75 (t, J = 7.2 Hz, 2H, C2–CH2–CH2), 3.56–3.54 [m, 4H, NH2+–CH2–CH2–NH+(CH3)2], 3.43 (t, J = 7.2 Hz, 2H, C2–CH2), 3.32 [s, 6H, C7–(CH3)2], 2.93 ppm [s, 6H, NH+(CH3)2]; mp 127 °C; IR (KBr): ν = 728, 848, 999, 1118, 1267, 1394, 1471, 1635, 2458, 2657, 2967, 3344, 3423 cm−1; HRMS (ESI) calcd for C17H28N5: 302.23392 ([M + H]+), found: 302.23392; elemental analysis for C17H27N5·3.0HCl·2.2H2O·0.25C2H5OC2H5·0.13CH2Cl2 (apparent MW = 480.0179): calcd C, 45.36; H, 7.80; N, 14.59; found C, 45.28; H, 7.85; N, 14.64.
2.2.5 Synthesis of TDMQ39
2.2.5.1 N1,N1-Dimethyl-4-nitrobenzene-1,3-diamine (C-TDMQ39)
To a solution of 5-chloro-2-nitroaniline in dry N,N-dimethylformamide were added K2CO3 and dimethylamine. The mixture was stirred at 150 °C for 12 h and quenched with water. After extraction with CH2Cl2, the organic phase was dried over MgSO4, filtered, and concentrated under reduced pressure. The crude mixture was purified by silica gel flash column chromatography (hexane/ethyl acetate, 10/1, v/v). After evaporation of the eluent, compound C-TDMQ39 was obtained as a yellow solid (99%). 1H NMR (CDCl3): δ = 8.01 (d, J = 9.6 Hz, 1H, H3), 6.14 (dd, J = 9.6 Hz, 2.6 Hz, 1H, H4), 6.12 (d, J = 2.6 Hz, 1H, H6), 3.05 ppm [s, 6H, C5–N(CH3)2].
2.2.5.2 N,N,2-Trimethyl-8-nitroquinolin-5-amine (D-TDMQ39)
The procedure was similar to that described for the preparation of D-TDMQ31, but starting from C-TDMQ39. D-TDMQ39 was obtained as a yellow solid (64%). 1H NMR (CDCl3): δ = 8.35 (d, J = 8.6 Hz, 1H, H4), 8.01 (d, J = 8.6 Hz, 1H, H6), 7.32 (d, J = 8.6 Hz, 1H, H7), 6.87 (d, J = 8.6 Hz, 1H, H3), 3.08 [s, 6H, C5–N(CH3)2], 2.75 ppm (s, 3H, C2–CH3).
2.2.5.3 N,N-Dimethyl-8-nitro-2-vinylquinolin-5-amine (E-TDMQ39)
The procedure was similar to that described for the preparation of E-TDMQ32, but starting from D-TDMQ39. E-TDMQ39 was obtained as a yellow solid (20%). 1H NMR (CDCl3): δ = 8.36 (d, J = 8.8 Hz, 1H, H6), 7.98 (d, J = 8.6 Hz, 1H, H4), 7.49 (d, J = 8.8 Hz, 1H, H7), 6.91 (dd, 1H, C2–CH), 6.82 (d, J = 8.6 Hz, 1H, H3), 6.36 (d, J = 17.6 Hz, 1H, C2–CH–CHtrans), 5.64 (d, J = 10.8 Hz, 1H, C2–CH–CHcis), 2.93 ppm [s, 6H, C5–N(CH3)2].
2.2.5.4 N1-(2-(5-(Dimethylamino)-8-nitroquinolin-2-yl)ethyl)-N2,N2-dimethylethane-1,2-diamine (F-TDMQ39)
The procedure was similar to that described for the preparation of F-TDMQ33, but starting from E-TDMQ39. F-TDMQ39 was obtained as a yellow oil (70%). 1H NMR (CDCl3): δ = 8.37 (d, J = 8.8 Hz, 1H), 8.09 (d, J = 8.6 Hz, 1H), 7.33 (d, J = 8.8 Hz, 1H), 6.89 (d, J = 8.6 Hz, 1H), 3.26–3.20 (m, 4H), 3.01 (s, 6H), 2.84 (t, J = 6.4 Hz, 2H), 2.51 (t, J = 6.4 Hz, 2H), 2.24 ppm (s, 6H).
2.2.5.5 2-(2-((2-(Dimethylamino)ethyl)amino)ethyl)-N5,N5-dimethylquinoline-5,8-diamine (G-TDMQ39)
The procedure was similar to that described for the preparation of G-TDMQ31, but starting from F-TDMQ39. G-TDMQ39 was obtained as a brown solid (80%). 1H NMR (MHz, CDCl3): δ = 8.48 (d, J = 8.6 Hz, 1H), 7.27 (d, J = 8.6 Hz, 1H), 6.99 (d, J = 8.0 Hz, 1H), 6.86 (d, J = 8.0 Hz, 1H), 3.36 (s, 4H), 3.00 (t, J = 5.6 Hz, 2H), 2.77 (s, 6H), 2.64 (t, J = 6.0 Hz, 2H), 2.02 ppm (s, 6H).
2.2.5.6 TDMQ39
To a solution of G-TDMQ39 in dry dichloromethane was added an excess of hydrogen chloride solution (2.0 M in diethyl ether). The precipitate was filtered, washed with diethyl ether, and dried under reduced pressure to give TDMQ39 as a black solid (99%). 1H NMR (D2O): δ = 8.42 (d, J = 8.8 Hz, 1H, H7), 7.71 (d, J = 8.8 Hz, 1H, H6), 7.65 (d, J = 8.8 Hz, 1H, H4), 7.24 (d, J = 8.8 Hz, 1H, H3), 3.76 (t, J = 7.0 Hz, 2H, C2–CH2–CH2), 3.57–3.51 [m, 4H, NH2+–CH2–CH2–NH+(CH3)2], 3.46 (t, J = 7.0 Hz, 2H, C2–CH2), 3.34 [s, 6H, C5–N(CH3)2], 2.93 ppm [s, 6H, NH+(CH3)2]; mp 100 °C; IR (KBr): ν = 806, 1051, 1124, 1186, 1259, 1480, 1633, 1664, 2892, 2954, 2973, 3440 cm−1; HRMS (ESI) calcd for C17H28N5: 302.23376 ([M + H]+), found: 302.23392; elemental analysis for C17H27N5·3.0HCl·3.5H2O·0.3C2H5OC2H5·1.0CH2Cl2 (apparent MW = 581.0351): calcd C, 39.69; H, 7.29; N, 12.05; found C, 39.60; H, 7.28; N, 12.04.
2.3 UV–visible titrations
All solvents and commercially available reagents were purchased from Fluka and TCI, and they were used without further purification. CuSO4·5H2O and ZnSO4·7H2O were used as the sources of metal ions. UV–visible (UV–vis) spectra were recorded from 200 to 600 nm, at room temperature, using an Evolution 300 spectrophotometer equipped with a magnetic stirrer. Preparation of TDMQ ligands is described in Section 2.2. Except otherwise noted, the stock solutions were as follows: TDMQ ligand (5 mM in H2O), CuSO4·5H2O (5 mM in H2O), ZnSO4·7H2O (5 mM in H2O), and Tris–HCl buffer 50 mM, pH 7.4. For each experiment, the solution of metal ions was added to the solution of TDMQ ligand in buffer. Final concentrations in the cuvette were as follows: [buffer] = 50 mM and [TDMQ] = 40 μM. The final volume was 1.5 mL.
Note that, according to the Recommendations of IUPAC for Nomenclature of Inorganic Chemistry, M2+ stands for the metal cation (salt) and M(II) stands for the oxidation state of the metal involved in a complex.
3 Results and discussion
3.1 Syntheses
These new TDMQ chelators consist of an 8-aminoquinoline scaffold substituted by methoxy or dimethylamino groups at variable positions. The structures of these ligands are presented in Scheme 1. To investigate the role of the substitution position, the 5-OCH3, 6-OCH3, and 7-OCH3 derivatives were prepared (TDMQ31, TDMQ32, and TDMQ33, respectively). To investigate the role of the different donating groups, TDMQ33 (7-OCH3) was compared with TDMQ38 [7-N(CH3)2], and TDMQ32 (5-OCH3) was compared with TDMQ39 [5-N(CH3)2].
The synthesis procedure of TDMQ31–TDMQ39 is summarized in Scheme 2. Starting from the suitable aniline (compound A) or 2-nitroaniline (C), the quinoline ring was generated by reaction with acetaldehyde in concentrated hydrochloric acid. The side chain is then introduced by vinylation at position 2 of the 8-nitroquinoline (E) and further reaction with N,N-dimethylethylenediamine (F). The 8-nitro group is then reduced by iron/acetic acid to yield the TDMQ base (G). This compound is then protonated by HCl to yield the water-soluble chloride salt (H).
3.2 Affinity of TDMQs for copper(II) and zinc(II)
The abilities of TDMQ31, TDMQ32, TDMQ33, TDMQ38, and TDMQ39 to chelate Cu(II) and Zn(II) were monitored by using UV–vis titration, in the range 200–600 nm, as previously reported [21]. Spectral modifications upon addition of aliquots of concentrated aqueous solutions of Cu2+ or Zn2+ sulfates in a TDMQ-buffered solution were recorded and considered significant of the metal complexation by the TDMQ ligands.
Upon addition of copper sulfate, a similar behavior was observed for ligands TDMQ31, TDMQ32, TDMQ33, TDMQ38, and TDMQ39. For example, titration of TDMQ33 (40 μM in Tris–HCl buffer, pH 7.4) by Cu2+ is depicted in Fig. 1. From 0.1 to 1.0 mol equivalent (mol equiv) of Cu2+, spectral changes were immediately observed. The absorbance at 255 nm of the free ligand decreased in favor of bands at 241 and 335 nm assigned to the CuII–TDMQ33 complex. Isosbestic points were detected at 247, 274, and 363 nm, well defined in the range 0–1 mol equiv of Cu2+ (Fig. 1), in agreement with the formation of a single Cu(II) complex. The plot of absorbance at 335 nm with respect to the Cu2+/TDMQ33 mole ratio (Fig. 1, Inset) clearly indicates that the metal chelation was complete under these experimental conditions (no equilibrium in solution), and that the metal/ligand stoichiometry of the complex was 1/1, as previously reported for another series of N4-tetradentate chelators, bis(8-aminoquinolines) [17,18,22]. No further changes were observed when an excess of Cu2+ was added (up to 2–50 mol equiv with respect to the ligand). Results obtained for TDMQ31, TDMQ32, TDMQ38, and TDMQ39 were similar to those for TDMQ33, significant of the formation of high-affinity Cu(II) complexes with L/M stoichiometries = 1/1 [23].

Titration of TDMQ33 (40 μM in Tris–HCl buffer 50 mM, pH 7.4) with CuSO4·5H2O. The reaction was carried out from 0 to 50 mol equiv of Cu2+ with respect to TDMQ33. Inset: absorbance at 355 nm as a function of the Cu2+/TDMQ33 mole ratio.
Under these conditions, the affinity of TDMQ ligands for Cu(II) cannot be calculated from titration curves. We therefore used 1,2-cyclohexylenedinitrilotetraacetic acid {CDTA, log Kapp[CuII–CDTA] at pH 7.4 = 17.1 [24]} as a competitive chelator, and the proportion of Cu–TDMQ at equilibrium in a mixture containing TDMQ/Cu(II)/CDTA = 1/1/1 was evaluated by UV–vis spectroscopy in Tris–HCl buffer at pH 7.4 [17]. Under these conditions (40 μM), the proportions of Cu(II) chelated by TDMQ31, TDMQ32, TDMQ33, TDMQ38, and TDMQ39 were 0.52, 0.53, 0.52, 0.68, and 0.26, respectively. As an example, results obtained for TDMQ33 are depicted in Fig. 2. Consequently, the log Kapp values for [Cu–L] were 17.2 for TDMQ31, TDMQ32, and TDMQ33, 17.8 for TDMQ38, and 16.2 for TDMQ39 (Table 1).

Determination of log Kapp of CuII–TDMQ33 by UV–vis spectroscopy by competition with CDTA in Tris–HCl buffer at pH 7.4. The blue trace is the spectrum of TDMQ33 alone (40 μM), the red trace is the spectrum of TDMQ33/Cu/CDTA = 1/1/1, the green trace is the spectrum of Cu/TDMQ33 = 1/1, and the yellow trace is the spectrum of Cu/CDTA = 1/1.
Affinity constants of TDMQ ligands for Cu(II) and Zn(II); selectivity for Cu(II) with respect to Zn(II).
n | M = Cu(II)a | M = Zn(II) | Cu(II)/Zn(II)b |
31 | 17.2 | 6.0c | 11.2 |
32 | 17.2 | 6.3c | 10.9 |
33 | 17.2 | 6.2c | 11.0 |
38 | 17.8 | 5.6c | 12.2 |
39 | 16.2 | 9.9d | 6.6 |
20e | 16.5 | 4.2 | 12.3 |
a Measured by UV–vis spectroscopy in the presence of CDTA as a competitor.
b Calculated as {log Kapp[CuII–TDMQ]} − {log Kapp[ZnII–TDMQ]}.
c Calculated from UV–vis titration of TDMQ ligand by Zn2+.
d Measured by UV–vis spectroscopy in the presence of EGTA as a competitor.
e Given for comparison. Data are from Ref. [19].
Titration of ligands TDMQ31, TDMQ32, TDMQ33, TDMQ38, and TDMQ39 (40 μM) with Zn2+ was performed in a way similar to titration with Cu2+ but using ZnSO4·7H2O (up to 100 mol equiv) as a metal source. The reactions were monitored by UV–vis spectroscopy. In all cases, chelation of Zn(II) by the TDMQ ligand was observed. For example, upon addition of Zn2+ into a solution of TDMQ33 (40 μM in Tris–HCl buffer, pH 7.4), the absorbance of the free ligand (255 nm) disappeared in favor of absorption bands at 244 and 335 nm assigned to the ZnII–TDMQ33 complex (Fig. 3). Isosbestic points detected at 246, 288, and 357 nm are in agreement with the formation of a single ZnII–TDMQ33 complex. Significantly different from titration with Cu2+, TDMQ33 was not saturated upon addition of a single Zn2+ mol equiv, but an excess of metal ion was required to achieve full metalation. The plot of absorbance at 335 nm with respect to the Zn2+/TDMQ33 mole ratio (Fig. 3, inset) clearly indicates the formation of a ZnII–TDMQ33 complex with 1/1 stoichiometry. In the presence of equimolar concentrations of Zn2+ and TDMQ33, ZnII–TDMQ, free TDMQ, and Zn2+ coexist in solution. The proportion of Zn–TDMQ33 (88% with respect to the overall amount of TDMQ33) allowed us to calculate the affinity of Zn(II) for TDMQ33: log Kapp [ZnII–TDMQ33] = 6.2. Details of the calculation are given in Ref. [25].
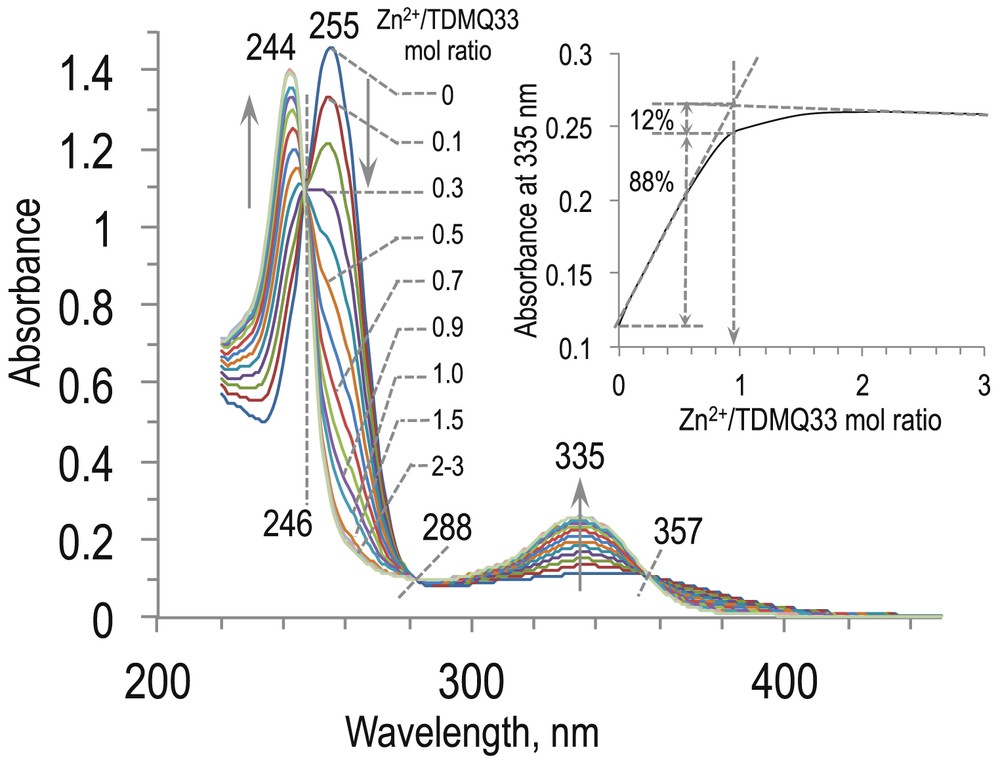
Titration of TDMQ33 (40 μM) with ZnSO4·7H2O (0–100 mol equiv) in Tris–HCl buffer 50 mM, pH 7.4. Inset: absorbance at 335 nm as a function of the Zn2+/TDMQ33 mole ratio.
Titration of TDMQ31, TDMQ32, and TDMQ38 with Zn2+ provided similar results, with metalation values of 85%, 90%, and 77%, respectively, upon addition of 1 mol equiv of Zn2+. The calculated values of log Kapp [ZnII–TDMQ] were 6.0, 6.3, and 5.6 (reported in Table 1).
Chelation of Zn(II) by TDMQ39 was more complicated (Fig. 4). Plot of the absorbance at 259 nm with respect to the Zn/TDMQ39 mole ratio (Fig. 4b) suggested the formation of a low affinity transitory complex with stoichiometry Zn/TDMQ39 = 1/2, followed by irreversible formation of a 1/1 complex under these conditions. This feature was confirmed by the absence of any clear isosbestic point in the range 310–340 nm (Fig. 4a, inset). The affinity of TDMQ39 for Zn in the 1/1 complex was measured by competitive chelation using ethylene glycol-bis(2-aminoethylether)-N,N,N′,N′-tetraacetic acid (EGTA) as a competitive chelator and was found to be log Kapp [ZnII–TDMQ39] = 9.9 at pH 7.4 (Fig. 5).

(a) Titration of TDMQ39 (40 μM) with ZnSO4·7H2O (0–100 mol equiv) in Tris–HCl buffer 50 mM, pH 7.4; inset: expansion of the region 290–390 nm. (b) Absorbance at 335 nm as a function of the Zn2+/TDMQ39 mole ratio.

Determination by UV–vis of log Kapp[ZnII–TDMQ39] by competition with EGTA in Tris–HCl buffer pH 7.4. The blue trace is the spectrum of TDMQ39 alone (40 μM), the red trace is the spectrum of TDMQ39/Zn/EGTA = 1/1/1, the green trace is the spectrum of TDMQ39/Zn = 1/1, and the yellow trace is the spectrum of Zn/EGTA = 1/1. log Kapp [ZnII–EGTA] = 9.4 at pH 7.4 [24].
This study indicates that TDMQ derivatives substituted by a methoxy group on the quinoline ring (TDMQ31–TDMQ33) exhibit the same high affinity for Cu(II) (log Kapp = 17.2), and similar lower affinities for Zn(II) (log Kapp in the range 6.0–6.3), giving rise to high selectivity values for Cu(II) with respect to Zn(II) (close to 11 orders of magnitude), whatever the position of the substituents on the quinoline ring. Substitution by dimethylamine at C7 (TDMQ38) resulted in a slight increase in the affinity for Cu (17.8) with respect to its methoxy analogue (17.2 for TDMQ33), whereas the affinity for Zn slightly decreased (5.6 for TDMQ38 compared with 6.2 for TDMQ33), resulting in a higher Cu/Zn selectivity. Substitution by dimethylamine at C5 (TDMQ39) resulted in a decrease in the affinity for Cu (16.2) with respect to substitution at C7 by 1.6 log unit. More importantly, TDMQ39 exhibited a rather high affinity for Zn(II), with a log Kapp value of 9.9. Consequently, the Cu/Zn selectivity was drastically reduced for TDMQ39 compared with other TDMQ ligands.
These data have been compared with those previously reported for TDMQ20, with the same side chain, but were based on a 5,7-dichloroquinoline nucleus [19] (Table 1). Log Kapp[CuII–TDMQ20] was 16.5, very similar to that of TDMQ39 (5-dimethylamino-, log Kapp = 16.2) and not far from the affinities of the methoxy derivatives TDMQ31–TDMQ33 (17.2), consistent with a coordination of Cu(II) in a N4-square planar environment. For Zn(II), the affinity of TDMQ20 was 4.2, significantly lower than that of all TDMQ derivatives bearing electron donating substituents. This feature suggests that the substituent-induced electronic effects do not clearly influence the affinity of TDMQ chelators for Cu(II), and slightly increased the affinity for Zn(II). The drastically different affinity of TDMQ38 and TDMQ39 (7- and 5-dimethylamino derivatives, respectively) for Zn(II) (5-log units of difference) should be noted. It may be due to the significantly different basicity induced by the N(CH3)2 substitution at C5 or C7 on the 8-aminoquinoline nucleus. Taking everything into consideration, for selective chelation of Cu in the context of AD pharmacology, TDMQ38 should be preferred.
4 Conclusion
The present study reports a series of new 8-aminoquinoline chelators containing electron donating groups, and their affinities for Cu(II) and Zn(II). These compounds exhibit high affinity for Cu(II) (log Kapp > 16), consistent with the formation of N4-square planar complexes with a Cu/TDMQ = 1/1 stoichiometry, as already reported by X-ray crystallography for TDMQ20, another 8-aminoquinoline chelator bearing the same side chain at C2 [19]. The affinity for Zn(II) is low, and associated with a very high selectivity for Cu with respect to Zn (≥11). The single exception is that of TDMQ39, which bears a N(CH3)2 substituent at C5. These results confirm that metal selectivity in the TDMQ series is more driven by the capacity of these chelators to specifically chelate Cu in a N4-square planar environment with the suitable side chain than by electronic factors induced by different substituents on the quinoline ring.
On the basis of its selectivity for Cu with respect to Zn, TDMQ38 is one of the promising chelators for regulation of copper homeostasis, with the previously reported TDMQ20, suggesting that such low-molecular-weight copper chelators should be able to regulate the homeostasis of copper in the brains of patients with AD [18], and should then be considered as anti-AD drug candidates. In addition, the C7–N–(CH3)2 substituent of TDMQ38 might improve the pharmacological behavior of TDMQ38 with respect to TDMQ20. Further investigation on TDMQ38 is therefore in progress.
Acknowledgments
This work was supported by CNRS (France), the Guangdong Province [grant number 2050205], and the Guangdong University of Technology [grant number 220418037] (PR China).