1 Introduction
The study of electrooxidation of organic substances on different types of electrodes has gained a lot of scientific interest because of its importance in the production of new materials by electropolymerization or electrodeposition. There is a high potential for their utilization because of the size exclusion properties of their deposited layers and also their potential to improve the sorption properties of solid surfaces.
Hydroxybenzaldehydes are phenol derivatives; therefore, their electrochemistry should be similar to that of phenols. Numerous works can be found in the literature about the electrooxidation of phenol and phenol derivatives performed in acetonitrile. Phenol and its derivatives get quickly electropolymerized onto platinum and carbon-based electrodes [1–5], but many derivatives produce reproducible voltammograms [6].
Because acetonitrile has a wide potential window and relatively high dielectric constant, it is suitable for the investigation of a wide range of redox-active materials. Moreover, many chemicals are soluble in this solvent owing to its moderate permittivity. Owing to these advantages, acetonitrile seemed to be an appropriate choice as a medium for our experiments.
In the work of Sharma et al. [7], electrochemical methoxylation was carried out with benzaldehyde derivatives. However, to the best of our knowledge, benzaldehyde and hydroxybenzaldehydes have not yet been investigated in nonaqueous solvents, and therefore, their electrochemistry is in the focus of this article.
2 Experimental
Analytical-grade chemicals were used to carry out the experiments. Polyether ether ketone (products of eDAQ)–sealed platinum and glassy carbon disc electrodes (diameter: 1 mm) were used as working electrodes to study the electrooxidation of the selected compounds. The solvent acetonitrile was dry with a water content of around 10−3 M. Before preparation of solutions, its water content was further reduced by adding anhydrous Na2SO4 to it. Before the investigations, both electrodes were polished with alumina (particle sizes: 1, 0.3, 0.05 μm) on a polishing cloth and washed thoroughly with deionized water. The equipment used for carrying out the experiments was a potentiostat (Dropsens, Spain). The counter electrode was a platinum rod, and a silver wire served as the reference electrode. The solutions were stirred before recording each curve. Tetrabutylammonium perchlorate (TBuClO4) served as the supporting electrolyte.
3 Results and discussion
3.1 Investigation of the hydroxybenzaldehydes on the platinum electrode
To get insights into the electrochemical behavior of the four hydroxy derivatives of benzaldehyde, five cyclic voltammograms were recorded with both electrodes. The solutions were stirred before each scan. The potential window was set to the range of 0–3 V (v = 0.1 V/s). The subsequent cyclic voltammograms obtained with the platinum electrode are shown in Fig. 1. Using the platinum electrode, only one peak showed up in the voltammograms that is attributable to the phenolic hydroxy group. The selected compounds contained a formyl substituent, which does not react in the chosen potential window. Therefore, their electrochemistry is very similar to that of phenol derivatives having electroinactive substituents. The results clearly show that 3-hydroxybenzaldehyde fouled the surface of platinum, but hardly any evidence of fouling can be observed in the case of 4-hydroxybenzaldehyde. These results suggest that the position of the substituent significantly influences the behavior of the selected compounds. This indicates that the radical formed during the charge transfer process reorganizes mainly to the resonant form where the unpaired electron is in the para position being ready to couple with another radical or reactant molecule. If this position is free from substituents, quick polymerization can take place.
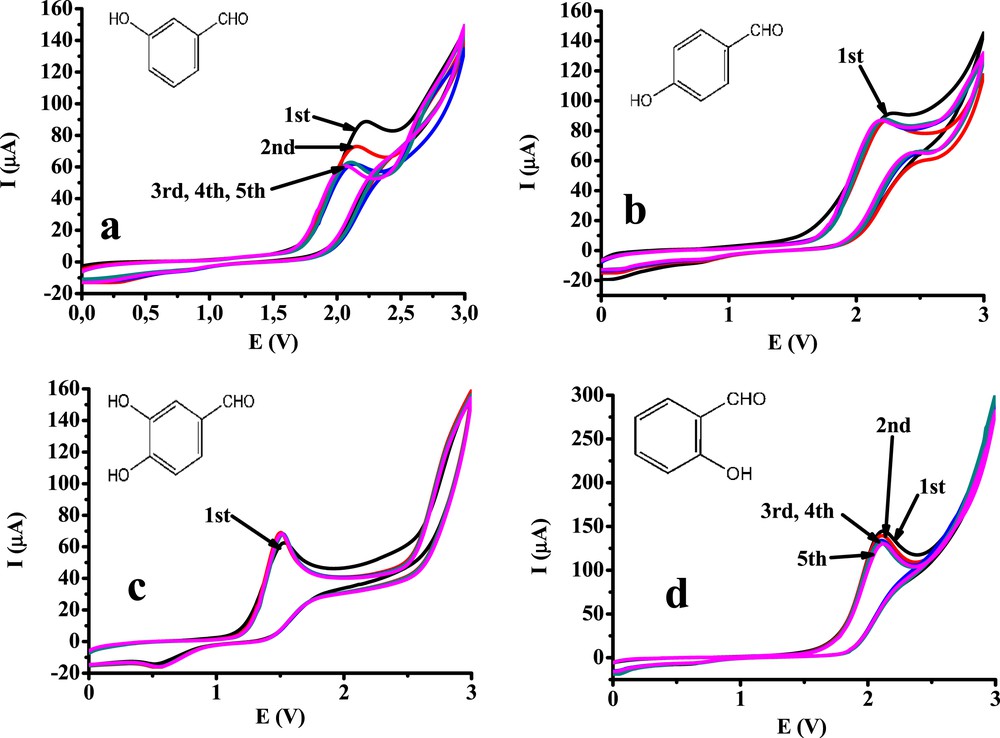
Repetitive cyclic voltammograms of 10 mM 3-hydroxybenzaldehyde (a), 4-hydroxybenzaldehyde (b), 3,4-dihydroxybenzaldehyde (c), and 2-hydroxybenzaldehyde (d) on a platinum electrode (v = 0.1 V/s, supporting electrolyte 0.1 M TBuClO4).
Contrary to the previous findings, 2-hydroxybenzaldehyde serves as an example for a derivative having no substituent in the para position showing weak passivation. The peak currents decreased slowly by repeating the scans. An explanation to this finding might be the steric hindrance caused by the formyl group located in the ortho position. When the corresponding radical forms during the electrooxidation, the other substrate molecules cannot reach the phenolic oxygen atom properly, but they can reach the para position of the cyclohexadienone ring because of the close neighborhood of the substituent. The radicals can therefore undergo mainly dimerization. As a result, the peak currents are a little higher than those of the other monohydroxy derivatives.
3,4-Dihydroxybenzaldehyde has a 1,2-dihydroxybenzene moiety (the same part as characteristic for 1,2-dihydroxybenzene), and its voltammograms have an oxidation peak at 1.5 V attributed to this moiety, yielding the corresponding 1,2-quinone. The peak heights were identical for all five scans as usual in the case of 1,2-dihydroxybenzene. Interestingly, the anodic peak currents hardly differed from those of the monohydroxy derivatives, despite the fact that two electrons are involved in the charge transfer process. It suggests that its diffusion coefficient is significantly lower than those of the monohydroxy benzaldehydes.
In summary, it can be concluded that on a platinum electrode, only the phenolic hydroxy group(s) react; thus, their electrochemistry is similar to that of other substituted phenols.
3.2 Investigation of the hydroxybenzaldehydes on a glassy carbon electrode
The cyclic voltammetry of the selected benzaldehydes was also performed with a glassy carbon electrode in acetonitrile (Fig. 2). As can be seen, the electrode was passivated quickly by derivatives containing only one hydroxy group. In an earlier work where para-substituted acetophenones and among them, 4′-hydroxyacetophenone was investigated in acetonitrile at higher anodic potentials, passivation took place continuously by repeating the scans [8]. In that work, it was demonstrated that the solvent itself contributed to the process. This separated experiment confirmed that the glassy carbon surface at high anodic potentials changes, which is attributable to the solvent acetonitrile because after scanning in it, no peak appeared in the voltammogram of the substrate 4′-hydroxyacetophenone. The observed fouling by the monohydroxy derivatives of benzaldehyde is more significant than that observed in the aforementioned earlier work by 4′-hydroxyacetophenone as very low currents were observed in the fifth cycle. The current enhancement that attributed to the solvent electrooxidation close to 3 V was not present, indicating that diffusion of the solvent molecules is also hindered because of the presence of a very compact film. An additional smaller voltammetric peak appeared at the shoulder of the one that is related to the phenolic hydroxy group (around 2.3 V) in the case of monohydroxy derivatives. The latter peak might be attributed to the oxidation of formyl group, which takes place on the glassy carbon electrode. It results in the formation of the corresponding benzoyl radicals coming from the electrooxidation of this group. They trigger the formation of chain polymers, which as radicals can couple with other monomers through the phenolic oxygen-producing ester bonds or dimerize through the benzoyl moieties. At potentials close to 3 V, both the phenolic hydroxy and the formyl group oxidize simultaneously producing biradicals; therefore, their coupling might occur in all possible modes. These products can be adsorbed on the electrode surface. Mainly, this process is responsible for the rapid deactivation (Fig. 2).
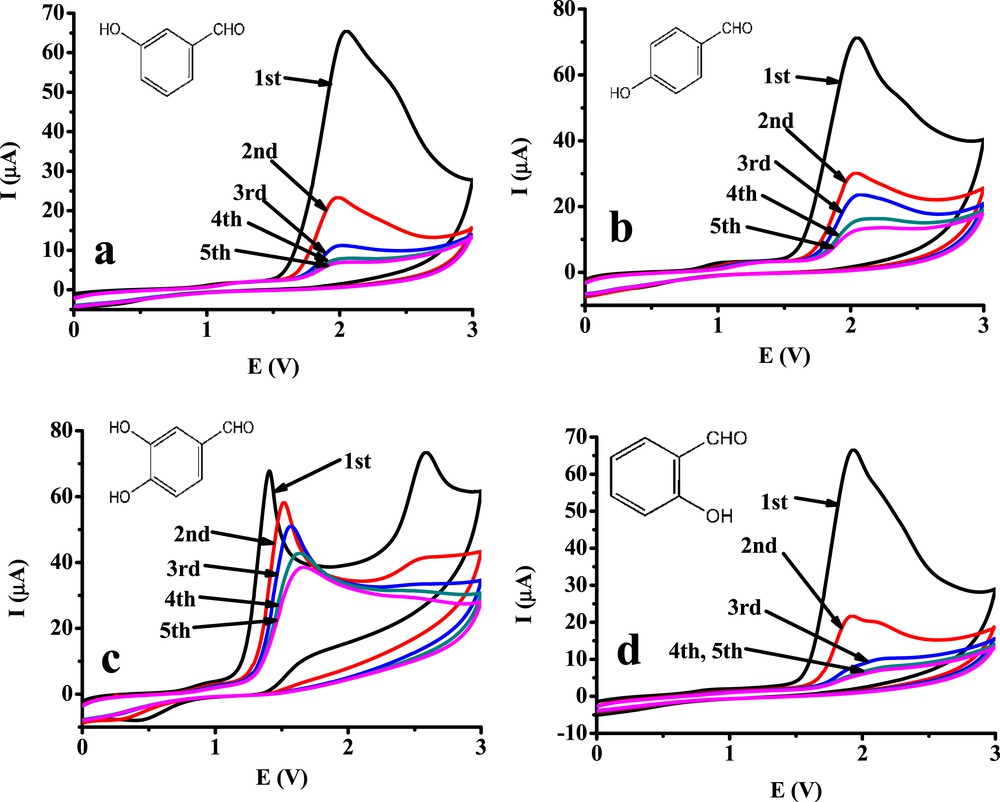
Repetitive cyclic voltammograms of 10 mM 3-hydroxybenzaldehyde (a), 4-hydroxybenzaldehyde (b), 3,4-dihydroxybenzaldehyde (c), and 2-hydroxybenzaldehyde (d) on a glassy carbon electrode (v = 0.1 V/s, supporting electrolyte 0.1 M TBuClO4).
The anodic peaks of 3,4-dihydroxybenzaldehyde associated with the 1,2-dihydroxybenzene moiety around 1.5 V decreased continuously in subsequent scans, and the peak potentials shifted continuously to more positive potentials. It is partly due to the surface deactivation caused by solvent electrooxidation in the previous scans at higher potentials. The second peak at around 2.6 V is due to the oxidation of the formyl group. By the appearance of this peak, the predominant formation of di(3,4-benzoquinone)-ethanedione occurs. By comparing its voltammograms with those of monohydroxy derivatives, it is obvious that the deactivation is less significant. The two neighboring hydroxy groups of 3,4-dihydroxybenzaldehyde oxidize rapidly to oxo groups, not being capable of coupling with radicals. This indicates that in the case of the monohydroxy derivatives, only the hydroxy group reacts, producing a phenoxyl radical that is capable of initializing polymerization. In the case of monohydroxy derivatives, the formed radical increases the rapid formation of polymer molecules which in turn are adsorbed onto the glassy carbon surface. The probable cause for the appearance of second smaller anodic peaks, in case monohydroxy derivatives are attributed to the subsequent oxidation of formyl groups at less positive potentials, attributable to the negative shift caused by the adsorption of the previously formed polyphenol.
The voltammograms of 3,4-dihydroxybenzaldehyde were taken with the glassy carbon electrode also between 0 and 1.7 V (Fig. 3). The curves show that lowering the upper potential limit to the appearance of the first oxidation peak drastically decreases the rate of fouling as oxidation is limited mainly to the quinone moiety. The small decrease in anodic peak currents might be because of a very slow deactivation of the electrode surface. The used solvent provides an aprotic environment for the formed quinone molecules as they bound readily to the oxygen-containing functional groups present on the glassy carbon surface through hydrogen bonds [9]. The small increase in the cathodic peak heights seems to confirm it.
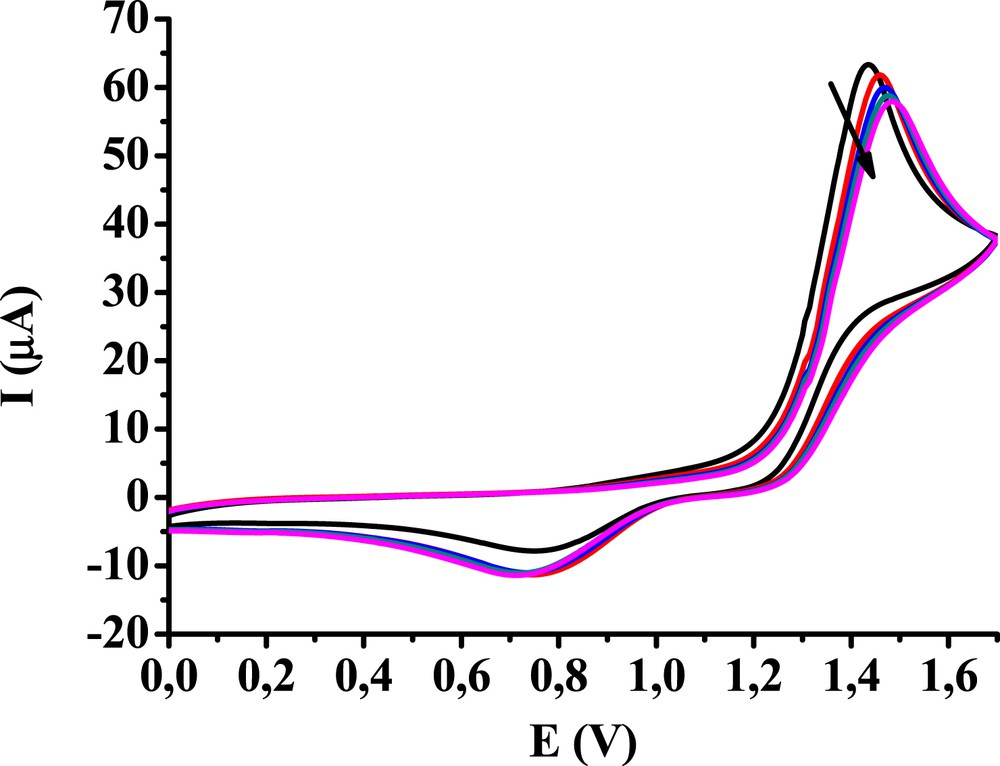
Subsequent cyclic voltammograms of 10 mM 3,4-dihydroxybenzaldehyde in acetonitrile between 0 and 1.7 V (v = 0.1 V/s, supporting electrolyte 0.1 M TBuClO4).
3.3 Complementary experiments with benzaldehyde
In the voltammograms of the benzaldehyde derivatives, a peak showed up at around 2.5 V. Complementary cyclic voltammetric experiments were carried out also with the unsubstituted benzaldehyde in acetonitrile between 0 and 3 V. On the platinum electrode, there was no oxidation peak at around 2.55 V and no fouling when investigating benzaldehyde (close to 3 V, its oxidation begins), but a peak appeared in the first voltammogram using the glassy carbon electrode (Fig. 4.). The maximum currents measured with this electrode are significantly lower than those measured with the platinum electrode, indicating surface passivation. Further unambiguous evidence is the significant current decrease in the subsequent scans and the absence of voltammetric peak in the second scan. In the first step, benzoyl radicals form which dimerize resulting in the final 1,2-diphenylethanedione product. This compound might be adsorbed on the glassy carbon surface and further oxidized on the aromatic ring, resulting in the formation of polymers. The benzoyl moiety can also react as it was demonstrated in an earlier work in the case of benzophenone: an anodic peak appeared in the first scan with the glassy carbon electrode [8]. It evidences unambiguously that in the previous studies with 3,4-dihydroxybenzaldehyde, oxidation of the formyl group took place where the second peak appeared and where the second smaller peak showed up by the monohydroxy derivatives. Moreover, for additional evidence, a voltammogram was also recorded with 2,4-dichlorobenzaldehyde (not shown) with the glassy carbon electrode. An anodic peak also appeared, but shifted to 2.75 V, because of the electron-withdrawing effect of chloro substituents.
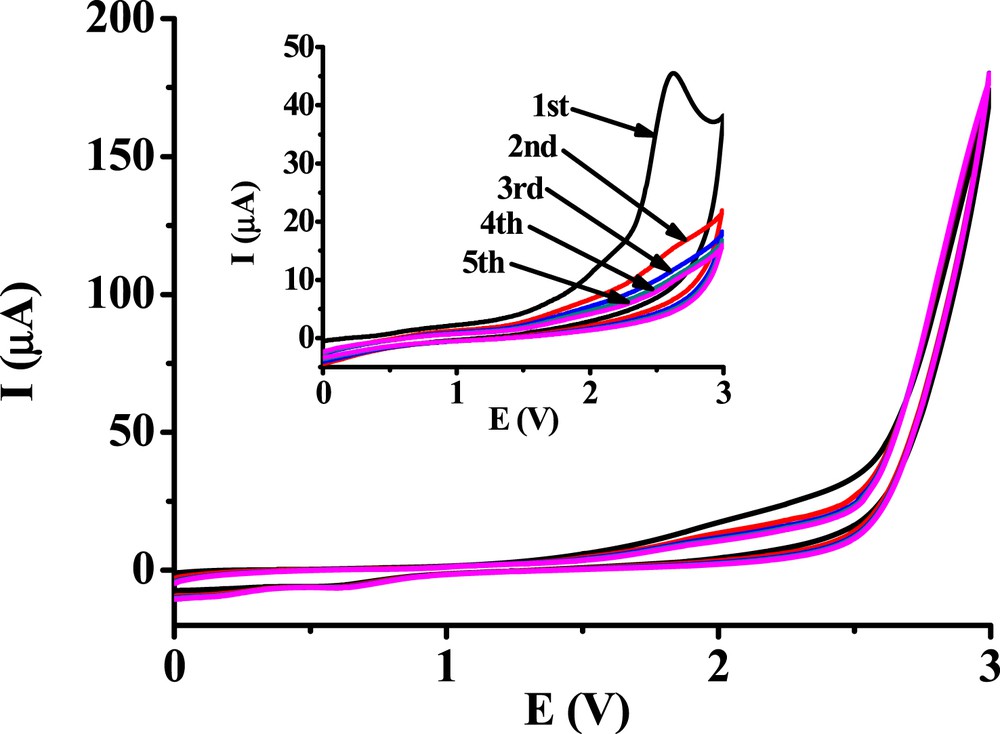
Subsequent cyclic voltammograms of 10 mM benzaldehyde at a platinum electrode and a glassy carbon electrode (inset graph) (v = 0.1 V/s, supporting electrolyte 0.1 M TBuClO4).
4 Conclusion
The study of electrooxidation of benzaldehyde and hydroxybenzaldehydes showed that these derivatives react on platinum and glassy carbon electrodes in very different ways. On the glassy carbon surface, the polymer formation can take place, making possible the preparation of new types of polymers originating from monomers bearing a formyl group. Further work is needed to clarify the transport properties of 3,4-dihydroxybenzaldehyde.
Acknowledgements
Financial support of the GINOP 2.3.2-15-2016-00022 (Hungary) is highly appreciated.