1 Introduction
Noncellulosic wood polysaccharides are of great interest because of their structural diversity and functionality. The potential for application of polymeric hemicelluloses has been demonstrated by several authors [1–5]. As a result, new utilizations of hemicellulosic polysaccharides are emerging in the fields of bioactive polymers, hydrocolloids, papermaking additives, or coating films [6,7]. Softwood hemicelluloses are mainly constituted of galactoglucomannans (GGMs), approximately 14–20% of the wood [8], and arabinoglucuronoxylans (7–10% of the wood). Softwood xylans are not acetylated, but the xylan backbone is substituted at carbon 2 and 3 with 4-O-methyl-α-d-glucuronic acid and α-l-arabinofuranosyl residues, respectively. Softwood xylan has a relatively low polymerization degree (PD) of about 100 [9]. GGMs have been described to have an approximate degree of polymerization of 100–150, matching to a molar mass of 16–24 kDa [9]. The potential applications of spruce GGMs in biomaterials and hydrocolloids, such as barriers in food packaging, bioactive polysaccharides, hydrogels and fibers, and health-promoting agents, have been studied [3,5,10–14].
The extraction of polymeric hemicelluloses has been investigated for years. Extraction of softwood hemicelluloses has been studied by hydrothermal treatment at high temperatures (≥ 170 °C) [15,16], by chemical extraction (alkaline) [17–20] and ionic liquids [21], by pressured hot water [22,23], and by microwave heat fractionation (>180 °C) [24–26].
The fractionation of wood at high temperatures leads to high solubilization of all macromolecules, including hemicelluloses. However, extraction at high temperatures for long reaction times led to a low pH level, causing hydrolytic cleavages. As a result, GGMs extracted under these conditions are generally highly deacylated and exhibit low molecular mass. Chemical treatments, especially alkaline extractions, are described to produce higher molecular weight hemicelluloses. Nevertheless, alkaline extraction of hemicelluloses has some disadvantages. In addition to causing saponification of polysaccharides, extraction with highly concentrated alkaline solutions for long periods can lead to peeling reactions [27].
Nowadays, new processes of extraction have been developed to reduce the amount of chemical solvents, treatment time and temperature, as well as energy consumption, hence improving the recovery of valuable compounds with lower costs [28]. Microwave-assisted extraction (MAE) is one of the advantageous green extraction methods based on electromagnetic radiations with a frequency from 0.3 to 300 GHz. In this process, microwave irradiations are used to extract biomolecules. Unlike conventional heat treatment where the energy is transferred to the material by convection, conduction, or radiation from the surface of the material, microwave energy is delivered directly to the material through a molecular interaction with the electromagnetic field [29]. This interaction allows the heating of the material and a transfer of energy from the inside to the outside of the cell [30].
Several studies have been carried out on the effect of microwaves on the extraction of hemicelluloses from lignocellulosic materials. Microwave heating was shown to be efficient for the extraction of softwood hemicelluloses [25,26,31]. In 2014, Panthapulakkal has observed a slightly higher xylans extraction yield from wood fibers by MAE (58% of xylans) than by conventional extraction (54%) [32]. Similar results have been reported in pretreatment of maize cane using water and acid [33] and alkaline pretreatment of Panicum virgatum [34]. Free sugars are generally released from the degradation of polysaccharides during the extraction process [35]. Most of the published works are carried out at a temperature above 100 °C and at pressure above atmospheric pressure, with these conditions leading to degradation and depolymerization of hemicellulose chains.
In the present work, microwave heating at atmospheric pressure was carried out for the extraction of polymeric hemicelluloses under mild conditions (temperature <100 °C) to limit any possible degradation and depolymerization. The effect of MAE was compared with that of a conventional treatment at the same temperature. The effect of different conditions (power and/or temperature, pH of extraction) on the yield and the physicochemical properties of extracted hemicelluloses was assessed.
2 Materials and methods
2.1 Raw material
Spruce sawdust with a particle size of 1–5 mm was produced from a spruce tree from the Lorraine region of France and stored at room temperature in sealed polyethylene bags in dark until use. The average moisture content of the raw material was 10% w/w. The hemicellulose content of the sawdust was approximately 30% w/w dry biomass. Carbohydrate composition of sawdust includes 72% glucan, 7.5% xylan, and 16.3% mannan and other sugars.
2.2 Impregnation
For each experiment, a mass of 20 g of spruce sawdust was soaked with a solution of 1 M sodium hydroxide (ratio solid/liquid = 1:7) or water (ratio solid/liquid = 1:7). The sawdust was left for 15 h before treatment at room temperature.
2.3 Experimental methodology of extraction
2.3.1 Microwave activation
The MAE experiments for the extraction of hemicelluloses from spruce sawdust were carried out using a microwave laboratory system (230V-50 Hz, NEOS-GR; Milestone Technologies, Italy). This mono-mode apparatus operates at 2.45 GHz with maximum delivered power of 900 W regulated in 10-Wincrements with a temperature and power feedback/control. This instrument is equipped with a time controller. The experiments were performed at atmospheric pressure, and time and power were controlled and followed by the software. The MAE experiments were conducted in a glass extraction vessel. The samples were treated for 1 h using different selected powers. Indeed, the effect of microwave irradiation, nature of solvent, and consumed energy were studied. The liquid-to-solid ratio was fixed at 7 during microwave treatment.
The power values presented in all figures correspond to the effective power received by the sample () which was calculated by the following equations:
(1) |
(2) |
2.3.2 Diffusion
After microwave treatment, supplementary diffusion step was carried out by heating under reflux at low temperature to avoid degradation of polymeric chains (70 °C for 3 h). The liquid-to-solid ratio was fixed at 15 (w/w).
For comparison, conventional extraction, without microwave treatment, from impregnated spruce sawdust was treated in a similar extraction vessel heated using a water bath at 100 °C for 1 h (to highlight the effect of microwave, the time to reach 100 °C for conventional extraction is not included) and then followed by 3 h of extraction at 70 °C.
2.3.3 Separation of extracted hemicelluloses
After each experiment, the extract was filtered on a qualitative filter paper with retention particle size of 5–13 μm (VWR International, Fontenay-sous-Bois, France). The volume and end pH of the extract solutions were measured at room temperature shortly after the extraction. The liquid fraction from 1 M NaOH impregnated sawdust was neutralized to a pH of 5.5–6 by a solution of 72% H2SO4. Ten milliliters was taken from the filtered extract for analyses. The extract solution was concentrated by vacuum evaporation (BUCHI, France) using a water bath at 50 °C. To precipitate high-molecular-weight hemicelluloses, four volumes of 95% ethanol were added to the liquid extracts. The liquid phase was separated from the precipitated hemicelluloses by settling at room temperature and decantation. Hemicelluloses were further separated from the remaining liquid by centrifugation at 4000 rpm for 10 min at room temperature. The precipitated hemicelluloses were redissolved in distilled water and dialyzed using a dialysis membrane with a cutoff of 3.4 kDa (Spectrum Laboratories, USA). Finally, dialyzed hemicellulose solutions were freeze-dried. Fig. 1 shows the experimental procedure of extraction and separation from spruce sawdust.
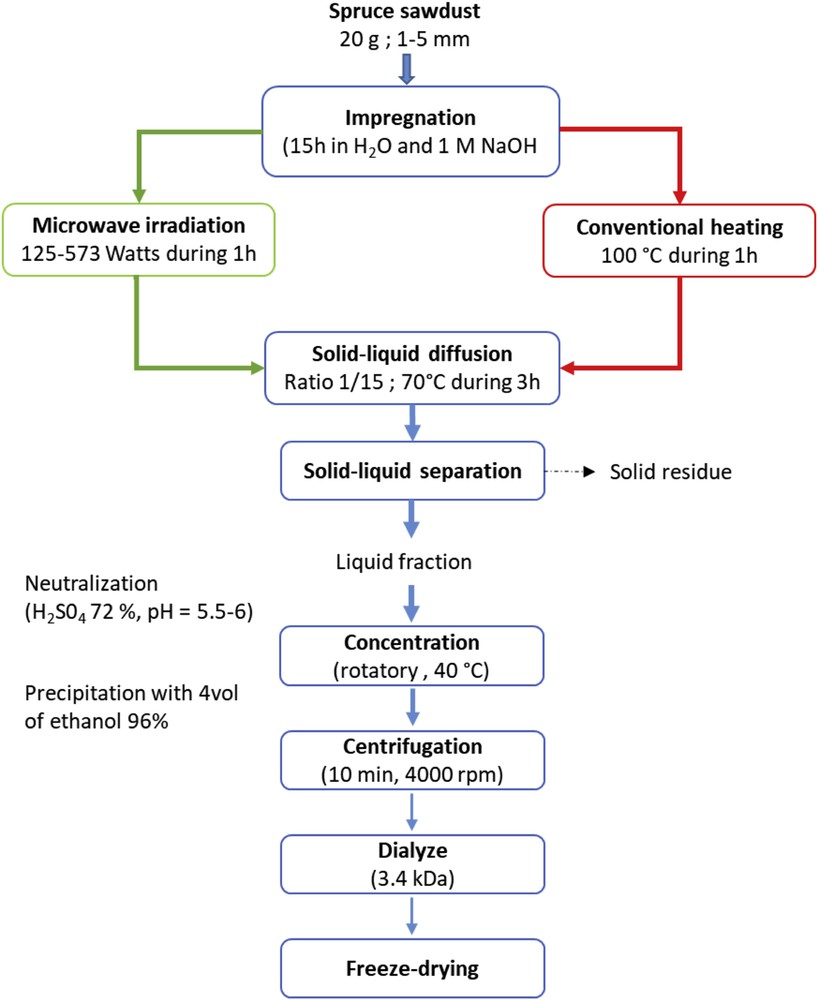
Scheme for extraction and separation of hemicelluloses from spruce sawdust using microwave-assisted extraction and conventional extraction.
2.4 Measurement of monosaccharides and uronic acids using HPAEC-PAD (high-perforformance anion exchange chromatography – pulsed amperometry detection)
The monosaccharide content of the soluble fraction was analyzed according to the method of Hames et al [36]. The sugar concentrations were measured by HPAEC-PAD (high-perforformance anion exchange chromatography – pulsed amperometry detection) (ICS-3000 Dionex, Dionex Corp., Sunnyvale, USA) equipped with a Dionex CarboPac™ PA-20 (3 × 150 mm) analytical column. Filtered samples (20 μL) were eluted at 35 °C and at 0.4 mL/min with the following composition: pure water 99.2%/250 mM NaOH 0.8%: 0–20 min; pure water 75%/250 mM NaOH 20%/NaOCOCH3(1 M)- NaOH (20 mM) 5%: 20–37 min; and pure water 40%/250 mM NaOH 20%/NaOCOCH3 (1 M)- NaOH (20 mM) 40%: 37–41 min. Each elution was followed by a wash and subsequent equilibration step. External sugar and uronic acid standards were used for calibration (7 points per curve). The reagents and standards are provided by Sigma-Aldrich and Fisher Scientific.
2.5 Degradation products
Degradation products of hemicelluloses (furfural, hydroxymethylfurfural [HMF], formic acid, and levulinic acid) are analyzed according to the procedure followed by Sluiter et al [37]. All the products are quantified using an Ultimate 3000 HPLC system. The components are separated in 45 min on an Agilent Hi-Plex H column at 55 °C with an isocratic flow rate of 0.4 mL·min−1 of sulfuric acid (5 ×10−3 M). Acids were detected by UV at 210 nm, while HMF and furfural are detected by UV at 280 nm. An external calibration is performed with six calibration points per standard. Reagents and standards are provided by Sigma-Aldrich.
2.6 Acetyl content
Acetyl content of extracted hemicelluloses was calculated by measuring the acetic acid concentration of hemicelluloses after a saponification reaction. Acid acetic was measured following the same procedure as degradation products with UV detection at 210 nm instead of 280 nm.
2.7 Size-exclusion chromatography
A Shimadzu Prominence HPLC system is used to determine molecular-weight distribution of hemicelluloses by gel filtration chromatography. The stationary phase is a Phenomenex Polysep GFC-P guard column (7.8 × 35 mm), Phenomenex Polysep GFC-P3000 and GFC-P2000 analytical columns (7.8 × 300 mm). The mobile phase is 0.25 M aqueous NaNO3. Samples are filtered using a 0.45-μm membrane, and 20 μL is injected. Samples are separated at 30 °C under a flow of 0.5 mL min−1, and detection is done using a refractive index detector (Shimadzu RID-20A). Each analysis runs for 70 min. Aqueous solutions (1 mg/mL) of dextran standards with molecular weights of 1000–80,000 were used to prepare the calibration curve.
2.8 Fourier-transform infrared
Fourier-transform infrared (FTIR) analysis for extracted hemicelluloses was performed by an FTIR spectrophotometer (Thermo Scientific iD3ZnSe ATR—Nicolet iS 5 FTIR Spectrometer unit). Hemicellulose powder was placed on a ZnSe crystal plate. Each infrared (IR) spectrum was obtained over the wave number range of 400–4000 cm−1 with eight scans at resolution of 4 cm−1. The spectra were analyzed using Thermo Scientific OMNIC software.
2.9 Two-dimensional (2D) 1H–13C heteronuclear single-quantum coherence (HSQC) analysis
Two-dimensional 1H–13C heteronuclear single-quantum coherence (HSQC) spectra were recorded using a Bruker III 400 MHz spectrometer equipped with a 5-mm BBFO (Broad Band Fluorine Observe) probe. The experiment was conducted with 20 mg of sample dissolved in 1 ml of D2O. The number of collected complex points was 1024 for the 1H dimension, with a relaxation of 1.5 times and 256 times increments recorded in 13C dimension. HSQC spectra were taken at room temperature.
2.10 Soluble lignin
The acid-soluble lignin (ASL) content was determined from absorbance values at 205 nm according to the laboratory analytical procedure (LAP) provided by the National Renewable Energy Laboratory [36].
(3) |
2.11 Wood solubilization
Wood solubilization (WS) was presented as the ratio of the wood dissolved to the weight of the wood used for extraction as expressed in Eq. 4:
(4) |
3 Results and discussion
3.1 Wood solubilization
Before microwave activation, spruce sawdust was impregnated in a solution of distilled water (neutral medium) or 1 M NaOH (basic medium) for one night. The aim of the impregnation step is to open the wood structure and to enhance the accessibility of hemicelluloses during the microwave extraction [38–40]. After microwave treatment, a diffusion step was carried out for 3 h at 70 °C. The effect of impregnation and diffusion steps on hemicelluloses yields +19% and 2.5%, respectively, in neutral medium and +16.4% and +30%, respectively, in basic medium. See Fig. S1 in Supplementary Material. The temperature profiles for conventional extraction and for MAE at different power are also given in SD section (Fig. S2).
Solubilization of wood was calculated using Eq. 3. As expected, the solubilization of wood is significantly higher in basic medium (Fig. 3). The MAE increased notably the effect of sodium hydroxide (+81% for 573 WW). The advantage of MAE over conventional extraction could be explained by the rapid and the selective heating of wood that lead to destabilization and structural damage of wood matrix, thus improving biomolecule extraction and also the heat and mass transfer which act in the same direction. Furthermore, the effect of MAE is related not only to the fast increase of temperature but also to the power transmitted to the wood matrix which destabilizes and weakens structural linkages between lignin and carbohydrates. In conventional extraction, heat transfer and mass transfer had opposite directions [41].
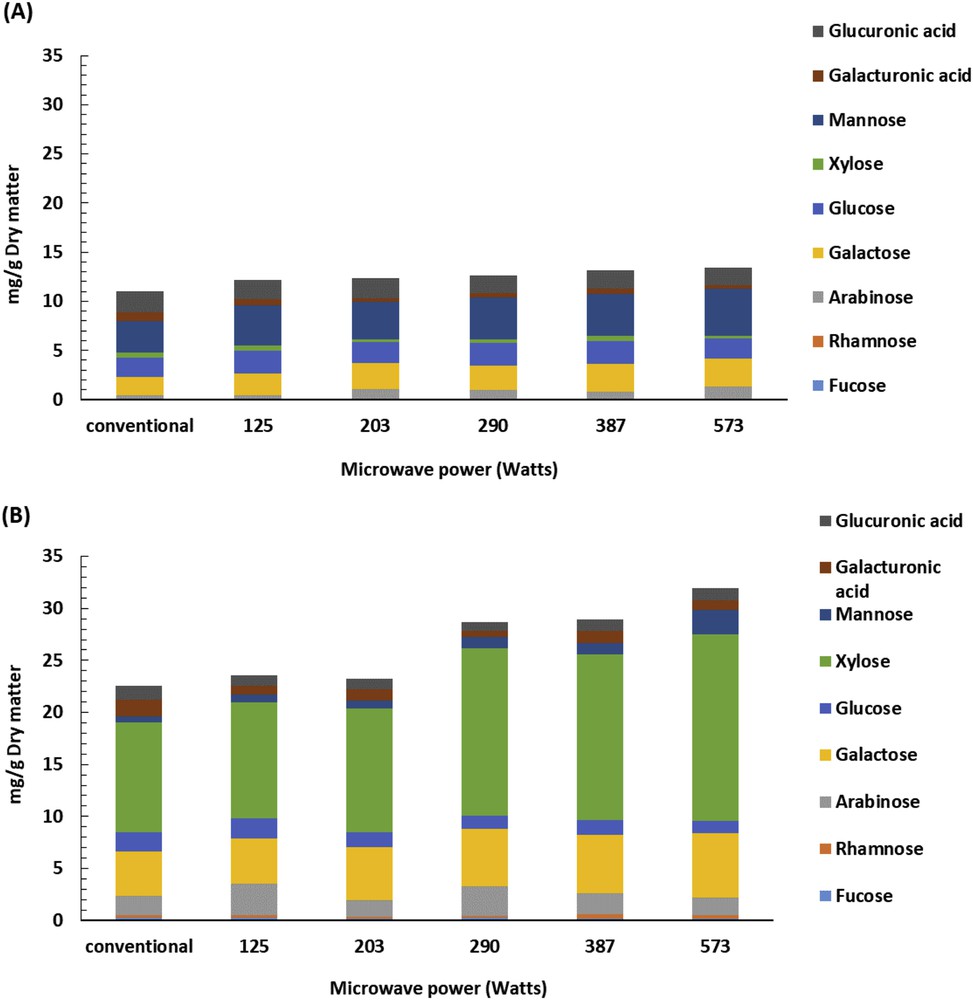
Yield and sugar composition of extracted hemicellulose polymers in neutral medium (A) and basic medium (B).
Wood solubilization steadily increased with the increase of microwave power. The solubilization of sawdust is mainly affected by microwave power which is the main parameter affecting the irradiation strength and the rate of temperature increase (Fig. 2). For basic impregnation, alkaline solutions increase the water absorption capacity, swell up cellulosic fibers and decrease their crystallinity, and thus increase the specific surface area [42]. Based on previous reports, the mechanism of action is due to the decrease of intermolecular hydrogen bonds by solvation [43], as well as lignin-hemicellulose bond scissions.
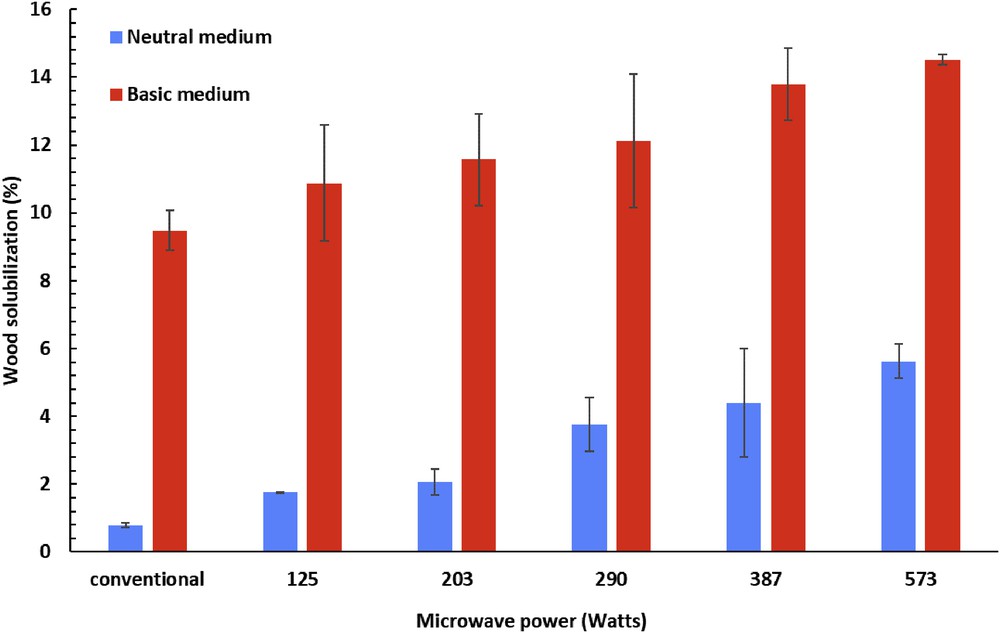
Solubilization of wood after MAE and conventional extractions (1 h under microwave, 3 h diffusion). MAE, microwave-assisted extraction.
3.2 Hemicellulose extraction
Polymeric hemicelluloses extracted in the liquid fraction were quantified by subtracting the amount of monomeric sugars (determined by ionic chromatography) from the total sugar content in the liquid fraction obtained after extraction. As expected, Figs. 3(A and B) show that the extraction of hemicelluloses highly depends on the pH of the impregnation step, as it affects the hydrolysis of the hemicellulose-lignin linkages. In fact, at maximum power (573 W) when sodium hydroxide was used as solvent, the effect of microwave on the extraction yield was higher than that of the conventional extraction (32.3 and 22.5 mg/g of dry wood, respectively). On the other hand, lower extraction yields were observed using water as solvent. To explain the effect of MAE on wood, Panthapulakkal et al. [44] have suggested that the disruption of wood structure and the rupture of wood fibers are due to the selective interaction of the microwaves with the inhomogeneous lignocellulosic materials. Hu et al., and Panthapulakkal et al., have found that microwave treatment improves surface disruption and breaking of lignin structures in alkaline-treated switch grass, while in conventional heating, the fibers remain intact [34,44].
The composition of the liquid fraction in terms of monomeric sugars (after acid hydrolysis) at different conditions of microwave extraction is also given in Figs. 3(A and B). Polysaccharides extracted in the neutral medium are mainly composed of mannose (33%), glucose (18%), and galactose (20%). As a result, we can assume that the hemicellulosic extract is mainly composed of galactoglucomannans [9]. On the other hand, in the basic medium, the high contents of xylose (57%) and arabinose (10%) suggest the dominant extraction of arabinoxylans. This selectivity of extraction as a function of the pH of the medium is in accordance with previous reports. It has been described that in neutral or mild acid medium (pH ≈ 5), hemicelluloses extracted from softwood are rich in mannose and acetylated glucomannans, exhibiting a relatively high water solubility [9,31]. In basic medium, the high xylose content can be rationalized by the dominant extraction of arabinoglucuronoxylans. In fact, arabinoglucuronoxylans are substituted with methyl-glucuronic acid and arabinose moiety which affect their solubility [45]. Thornton et al. [46] have found from the Norway spruce wood that at pH values greater than 10, the concentration of extracted arabinose and xylose units increases, suggesting the release of arabino-(4-O-methylglucurono)xylans.
The oligomeric and polymeric fractions of the extracted hemicelluloses were calculated by subtracting the amount of monomeric sugars from the total sugar content in the extracts. The monomer proportion is very low (< 2%) whatever the extraction conditions, the mild conditions used in this study (T < 100 °C, pH > 5) limiting the hydrolytic cleavage of hemicelluloses. Based on literature data, the hydrolysis of hemicelluloses into monomeric sugars is promoted by extraction at higher temperatures for longer times and lower pH level [31].
Solubilization of lignin during the extraction of hemicelluloses is expected especially in the basic medium. As shown in Fig. 4B, the amount of soluble lignin increased after microwave sawdust irradiation. A significant difference was observed for MAE at high power input (4.8% and 0.49% at 573 W for 1 h compared with 3.3% and 0.3% at 100 °C for 1 h in basic and neutral medium, respectively). Acetic, uronic, formic and levulinic acids have also been detected in the liquid effluents. The carboxylic acid contents were relatively important in basic medium (Fig. 4A). Acetic and formic acids are the major carboxylic acids formed (6 and 1.7 g/l, respectively). Levulinic acid presents the lowest concentration, accounting for 0.3 g/l. The peeling reactions occurring during alkaline treatments lead to endwise degradation of polysaccharides with formation of formic acid and different dihydroxy and dicarboxylic acids [47]. Acetic acid is released from the hemicellulosic backbone by saponification of acetate groups.
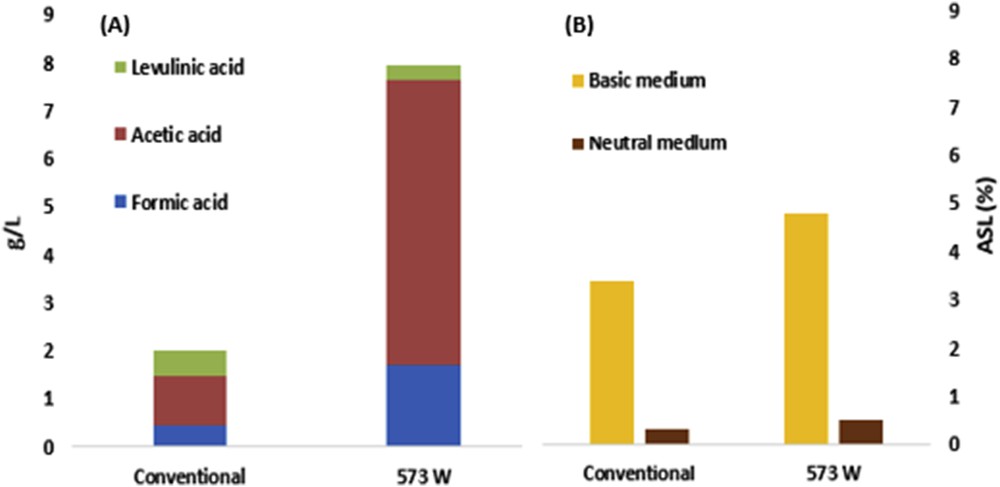
Degradation products in the liquid fraction in basic medium (A) and ASL (%) (B). ASL, acid-soluble lignin.
3.3 Composition of the recovered hemicellulose powder
The sugar composition of polymeric hemicelluloses recovered after precipitation and dialysis is similar to the sugar composition of the liquid fraction (Fig. 5). Galactoglucomannans and arabinoxylans are the main polysaccharides extracted in neutral and basic medium, respectively. In neutral medium, MAE promotes the extraction of arabinose and galactose compared with conventional extraction. In fact, hemicelluloses obtained from neutral medium account for 24% and 19.5% of galactose and 10.5% and 5% of arabinose for mircowave (573 W) and conventional extraction, respectively. The advantage of MAE not only seems to be due to the temperature but also is owed to the physicochemical interactions induced by microwave heating of the wood matrix.
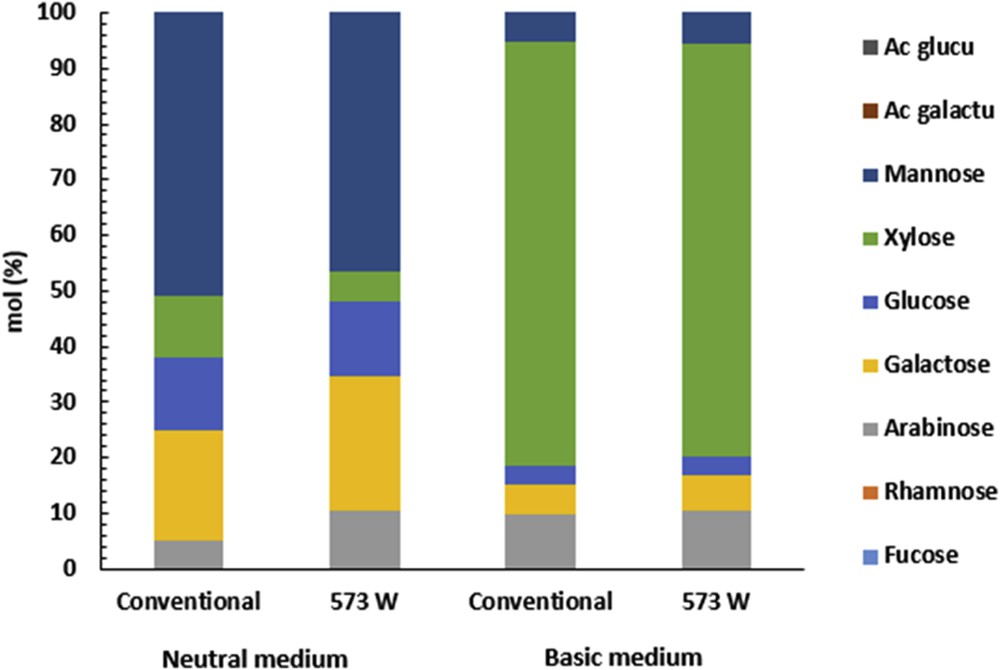
Sugar composition of recovered hemicelluloses by MAE and conventional extraction.
In native softwood, mannopyranosyl units are acetylated at C-2 or C-3 with a degree of acetylation of about 0.5 [9,17]. In our study, the precipitated hemicelluloses recovered from a neutral medium had a lower degree of acetylation, in the range of 0.10–0.15. In basic medium, the acetyl groups have been totally released from the hemicellulosic backbone by saponification.
These results have been confirmed by an FTIR study. In Fig. 6, two weak absorbances at 1735 and 1247 cm−1 due to acetyl groups attached to the hemicellulose chains were observed for the fractions extracted in neutral medium but not for the basic medium extracts. Moreover, the absorption at 1515 cm−1 observed in all the hemicellulosic fractions was assigned to lignin's aromatic skeletal vibrations [48]. The higher intensity of this signal for the fractions isolated from basic media confirms the higher lignin contamination for hemicelluloses obtained in basic medium than those obtained in neutral medium previously observed (Fig. 4B).
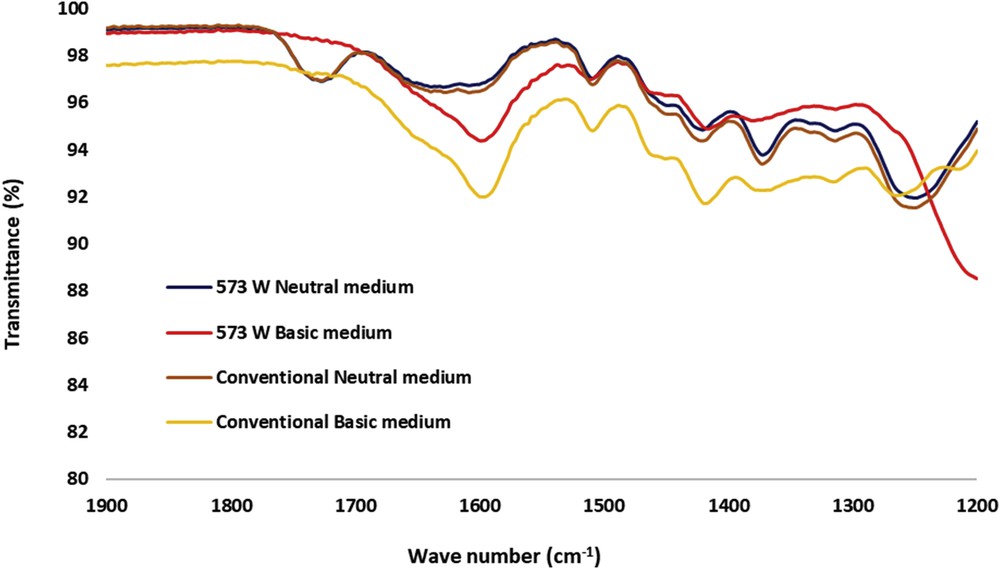
FTIR spectra of extracted hemicelluloses. FTIR, Fourier-transform infrared.
Two-dimensional 1H–13C HSQC spectra clearly show the typical signal spots expected for the hemicellulosic moieties. In basic medium (Fig. 7B), β-d-xylopyranoside units are specified by these signals at δC/δH 60/3.4; 62/4; 72/4.3; 72.5/3.2; 74/3.5; 72.5/3.7. The signals at 72/3.5 are assigned to α-d-xylopyranoside of xylans reducing end, and those at 62/3.3 and 71/3.5, to β-d-xylopyranoside of xylans nonreducing end. In neutral medium (Fig. 7A), the signals at 3.4/74; 3.5/74; 3.8/76; 4.2/70 are attributed to β-d-mannopyranoside. The same results were found in the study by Giummarella and Lawoko [49]. The signal in neutral medium at 5.45/72 was assigned to 6-O-acetyl β-d-mannopyranoside. In fact, as confirmed by the FTIR analysis, the extraction in neutral medium preserved some of the acetyl groups of the backbone units of GGM. These results are in close agreement to those obtained by Thornton et al [46] who found that deacetylation of the O-acetyl-galactoglucomannans appeared to begin at pH values above 8. The presence of acetyl residues is critical for the water solubility of GGM. The acetyl moieties are generally cleaved during the extraction at high temperatures leading to the release of acetic acid. The signals at 98/5.25 and 82/3.1 are assigned to 4-O-methyl-α-D-glucuronic acids that are spotted only in basic medium which confirms the extraction of arabinoglucuronoxylans in these conditions.
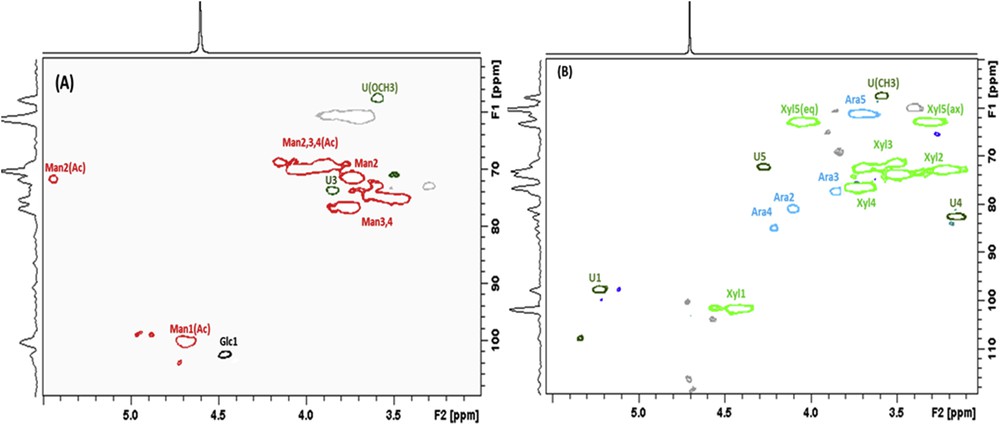
HSQC spectra of extracted hemicelluloses (A) in neutral medium and (B) in basic medium.
Weight-average (Mw) and number-average (Mn) molecular weights, as well as the polydispersity index (PDI), were determined by gel-permeation chromatography. The results in Table 1 show that hemicellulose fractions isolated from microwave irradiation display higher molecular mass than the conventionally extracted fractions (for conventional extraction, Mw ∼38,000 and ∼56,000 g mol−1 in neutral and basic medium, respectively, and ∼42,000 and ∼67,000 g mol−1 for hemicelluloses extracted with microwave activation in neutral and basic medium, respectively). It can be also observed that the hemicelluloses extracted in neutral medium had much lower molecular weights than those isolated in basic medium. These results were in close agreement to those reported by Sun et al [50] who found that increasing the alkali concentration during extraction increased the Mw of extracted hemicelluloses by 46%. They suggested that the extraction of high Mw hemicelluloses was owed to the increase of the coextraction of lignin-carbohydrates complexes. Considering the sugar composition given in Fig. 5, it can be concluded that the neutral medium released GGM with relatively low molecular weight, while basic extraction allowed the recovery of high-molecular-mass xylans.
Weight-average (Mw) and number-average (Mn) molecular weights, polydispersity index (PDI), and the polymerization degree (PD) of the hemicellulosic fractions obtained with MAE at 573 W and conventional extraction.
Basic medium | Neutral medium | |||||||
Mn | Mw | PDI | PD | Mn | Mw | PDI | PD | |
Conventional | 27,266 | 56,298 | 2.06 | 351 | 20,730 | 37,870 | 1.82 | 236 |
MAE 573 W | 54,227 | 66,564 | 1.22 | 416 | 23,875 | 41,589 | 1.74 | 259 |
4 Conclusion
In this study, the extraction of the hemicellulose polymers by MAE at atmospheric pressure was investigated. The highest amount of hemicelluloses extracted was 27.5 mg/g of dry wood from presoaked wood in a 1M NaOH solution using microwave treatment at 573 W for more than 1 h. At this condition, the Mw of the extracted hemicellulose chains was 66 kDa. At the same conditions, i.e. temperature and time, the yield and the Mw were lower than those obtained by conventional heating than microwave extraction, which highlights the efficacy of MAE for extraction of hemicellulose chains from spruce sawdust. Presoaking in sodium hydroxide improves the solubilization of wood and hence the extraction of high-molecular-weight hemicelluloses. However, presoaking in water preserves acetyl groups in the hemicellulose chains which could be advantageous in various polymer applications. High extraction selectivity was observed as a function of the presoaking medium: Acetylated galactoglucomannans were isolated after presoaking in neutral medium, and high-molecular-mass arabinoglucoronoxylans, in basic medium. MAE at atmospheric pressure and mild conditions (temperature < 100 °C and pH 5) has shown its effectiveness to extract high-molecular-weight hemicellulose chains from spruce wood with less depolymerization and degradation. This technology has great potential as an alternative method to autohydrolysis and hydrothermal treatments of wood in the aspects of molecular weight of extracted hemicelluloses.
Acknowledgements
This work was financially supported by SOFREN Group and ANRT (“Association nationale de la recherche et de la technologie”, France). The authors appreciate the financial support from SOFREN and ANRT. LERMAB is supported by the French National Research Agency through the Laboratory of Excellence ARBRE (ANR-12- LABXARBRE-01).
Appendix A Supplementary data
The following are the supplementary data to this article: Effect of the impregnation and the diffusion on the yield of extracted hemicelluloses by MAE at 573 Watts in neutral and basic mediums Temperature profile during MAE and conventional extraction