1. Introduction
Currently, the treatment of oilfield produced water is increasingly considered with the continuous exploitation of oil and gas field wastewater [1, 2, 3, 4, 5]. The complex is widely used in the process of oil recovery, so the treatment of oilfield produced water is increasingly difficult [6, 7, 8]. Consequently, it is significant to explore appropriate processes for the effective treatment of wastewater. The Fenton method is commonly used and considered expressly promising for wastewater treatment [9, 10, 11]. Fenton method has its extensive application prospects due to the fact that the H2O2 in Fenton system can break down into environmentally harmless species such as H2O and oxygen (O2) [12, 13]. However, the conventional Fenton system has rigorous requirements on the acidity and alkalinity of the oilfield wastewater [14, 15]. In recent years, there have been numerous studies on expanding the pH range of the Fenton system, but there are still some disadvantages such as high cost, limited pH range, and no practical application in the oilfields [16]. Therefore, we find an effective Fenton-based catalyst oxidation method for improving the application of Fenton oxidation under high pH value.
Therefore, our research aims at the orderly processing of waste in oilfield and seeking for efficient Fenton oxidation processing for the treatment of oilfield wastewater under an extensive pH range. In this study, the heterogeneous catalyst of B@Co(II)L was established for the Fenton oxidation. The catalyst was measured for degradation performance to the viscosity of hydroxypropyl guar gum (HPGG) and the chemical oxygen demand (COD). Besides, the catalyst was implemented at the oilfield wastewater, fracturing fluid, and drilling fluid. The experimental conditions of Fenton oxidation were optimized and the structures of catalyst were characterized.
Hydroxyl radical (HO∙) is one of the most effective oxidants, generated from a Fenton-like process; the supreme oxidation potential of HO∙ makes it a strong oxidant for water treatment. In this work, the main attempt was devoted to partially destroy HPGG contained in oilfield produced water and improve the degradability of the wastewater through the oxidation of metal–hydrogen peroxide (H2O2) in a wide pH range. H2O2 can be activated to produce HO∙ by using B@Co(II)L as a catalyst for the treatment of wastewater.
2. Materials and methods
2.1. Chemicals and materials
5-sulfosalicylic acid (98%), CoCl2, 6H2O (98%), NaOH (98%), and H2O2 (30%) were employed in the catalyst preparation. KMnO4 (98%), K2Cr2O7 (98%), FeSO4 (98%), and (NH4)2Fe(SO4)2∙6H2O (98%) were utilized in the COD removal. All reagents used were of analytical grade and were used as purchased without further purification. Hydroxypropyl guar gum (HPGG), carboxymethyl cellulose (CMC), and polyacrylamide (PAM) (purity > 95%) were obtained from Changqing Oilfield. Bentonite was obtained from Fengyun Chemical Co., Ltd., Xi’an, China. The instrument for measuring automatically for kinematic viscosity of petroleum products (SYD-265H) was purchased from Benshan Instrument Equipment Co., Shanghai, China. Ubbelohde viscometer was purchased from Shenyi Glass Co., Shanghai, China.
2.2. Catalyst preparation
Complex of Co(II)L was prepared by blending 2.4 g CoCl2, 6H2O and the ligand solution (L) of 2.5 g 5-sulfosalicylic acids in the ratio of 1:1 in a flask under stirring at room temperature for 2 hours. The synthesis process of Co(II)L complex was exhibited in Figure 1(a).
Then 5.5 g bentonite (B), dried before the experiment, was added to the solution achieved above, and stirred for 4 hours under 60 °C for 2 hours. Then the solid sample was centrifuged at 3000 rpm and washed twice with 20 mL distilled water. Finally, the supported catalyst (B@Co(II)L) was obtained after drying overnight at 70 °C. The preparation diagram is presented in Figure 1(b).
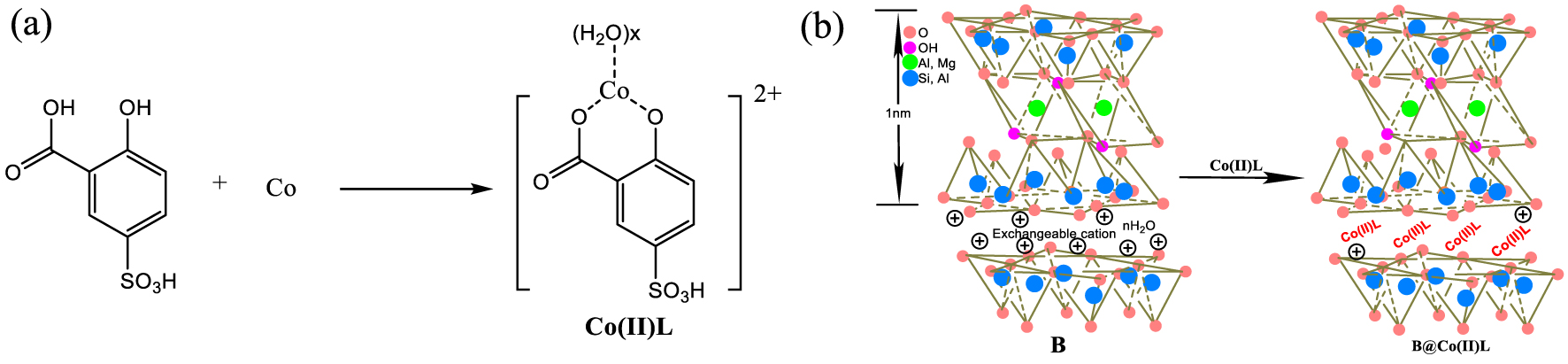
Synthesis of Co(II)L complex (a) and the preparation diagram of B@Co(II)L (b).
2.3. Degradation process
First, 1.2 g HPGG was dispersed in 200 mL distilled water at room temperature and stirred for 30 minutes under a magnetic stirrer with a stirring rate of 100 rpm for 12 hours to complete the swelling. Then, different amounts of H2O2 and catalysts were added to the solution weighing to obtain a heterogeneous mixture, and the pH was adjusted by NaOH [17, 16, 18]. Finally, the relative viscosity of hydroxypropyl guar gum solution compared with water was carried out at 45 °C using an Ubbelohde viscometer intermittently [19], and the relative molecular mass was measured using Ubbelohde viscometer at a certain temperature according to the previous report [18, 19]. The significant factors of different reaction temperature, pH value, catalyst dosage, and hydrogen peroxide (H2O2) concentration in heterogeneous degradation process were investigated in detail. The chemical oxygen demand (COD) was evaluated according to the Chinese National Standard-GB11914.
2.4. Catalyst characterization
The supported catalyst was characterized by various characterization methods. The morphology and elemental composition analysis of heterogeneous catalysts were obtained with powdered samples by means of a field emission scanning electron microscope (SEM, JSM-6390A, JEOL, Japan). The phase composition and purity of the catalyst were analyzed by powder X-ray diffraction (XRD) (JDX-3530, JEOL, Japan) on an XRD-6000 diffractometer used with Cu Kα radiation at 40 kV voltage and 15 mA current. All IR measurements using the KBr pellet technique (1 mg of a sample homogenized with 200 mg KBr) were performed on a Fourier infrared spectrometer at room temperature in the range 400–4000 cm−1 and each sample of 64 scans with a resolution of 0.4 cm−1 were recorded. N2 adsorption–desorption isotherms were performed on a Micrometrics ASAP 2020 HD88 instrument (Norcross, GA, USA) at 77 K, for which the samples were degassed at 573.15 K for 4 hours before the measurement. The surface area and pore structure were calculated utilizing the Brunauer–Emmett–Teller (BET) method and Barrett–Joyner–Halenda (BJH) Model, respectively.
3. Results and discussion
3.1. Catalyst characterization
B@Co(II)L was a complex generated from the reaction of 5-sulfosalicylic acid and cobalt chloride loaded on bentonite (B). The morphology of B@Co(II)L was characterized by scanning electron microscopy (SEM) scanned in ×5000 at first. As shown in Figure 2(a), the morphology of the supported cobalt-based complex has no significant change in that of bentonites.
Meanwhile, the phase composition and purity of catalyst were analyzed by powder X-ray diffraction (XRD). As exhibited in Figure 2(b), the reflections marked by (Q) are the type of impurities corresponding to quartz, and the other reflections are mainly the characterizations of the montmorillonite marked by (M) [20]. It is obtained that the diffraction reflections at 2θ = 36.58°, 61.54°, and 68.45° (ICDD) are assigned to cobalt oxide, which indicates the loading of cobalt-based complex Co(II)L over bentonite.
Furthermore, the catalyst was analyzed by infrared spectrum as shown in Figure 2(c). The peaks at 3424.7 and 1615.8 cm−1 correspond to the –OH stretching vibrations of the structural hydroxyl groups in the clay and interlayer adsorbed water and bending vibrations of water molecules present in the montmorillonite and in KBr. In addition, the peak at 1355.3 cm−1 is attributed to the bending vibrations of O–H and the stretching vibrations of S =O in the structure; the band at 1034.7 cm−1 is attributed to the stretching vibration of Si–O; the absorption bands at 528.6 cm−1 and 487.9 belong to Si–O–Al and Si–O–Si bending vibrations, respectively [21, 22, 23, 24]. According to the data above, it is obviously obtained that the Co(II)L complex has loaded on bentonite.
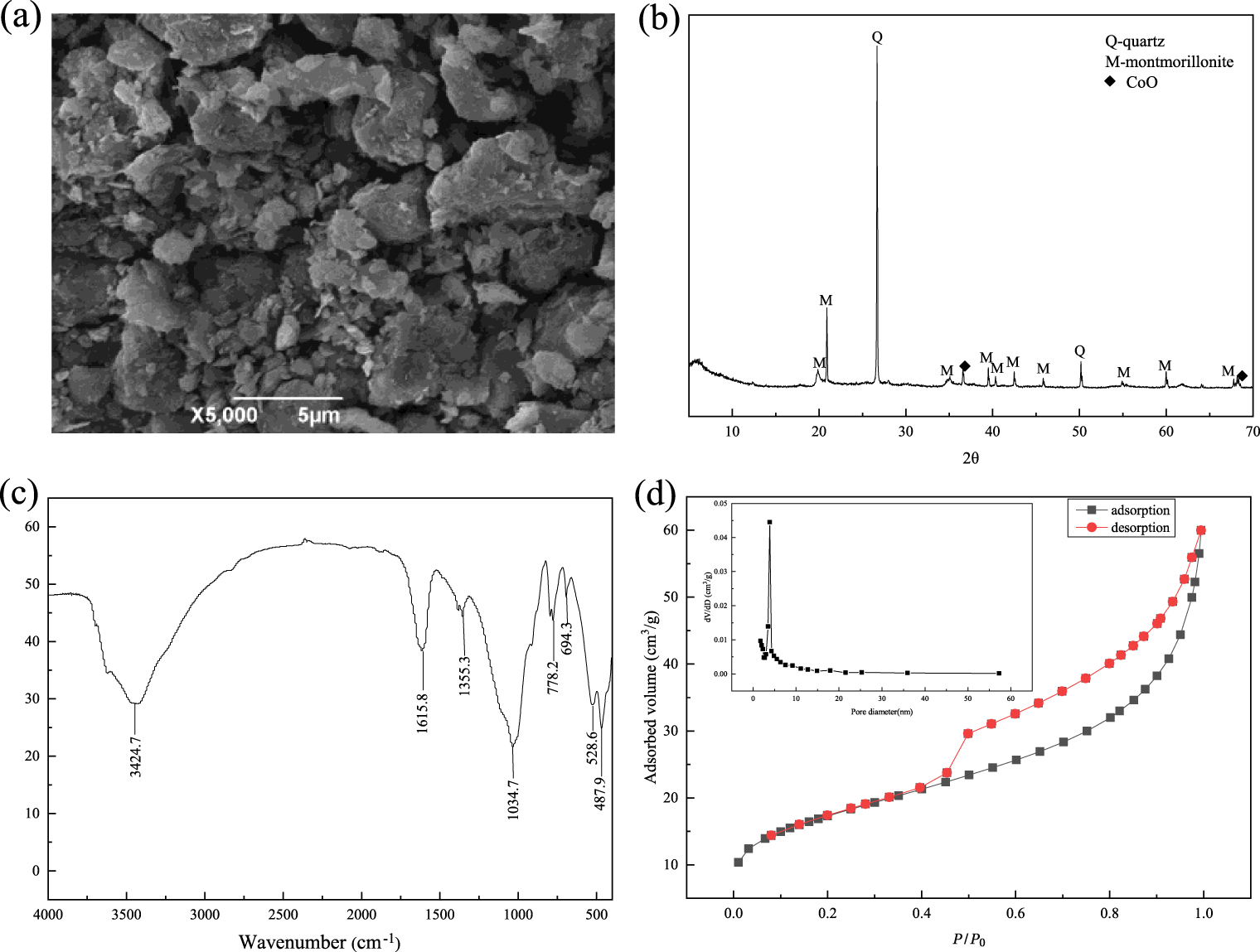
SEM of B@Co(II)L (a), XRD pattern of B@Co(II)L (b), FT-IR spectra of B@Co(II)L and nitrogen adsorption–desorption isotherms and pore size distribution of B@Co(II)L.
Pore properties of B@Co(II)L
BET surface area (m2/g) | Pore volume (cm3∕g) | Pore size (nm) |
---|---|---|
59.904 | 0.089 | 5.6155 |
In addition, the N2 adsorption–desorption isotherms of B@Co(II)L were achieved by the method of Brunauer–Emmett–Teller (BET). The surface areas, pore volumes, and pore size are showed in Table 1, and the N2 adsorption–desorption isotherm of the supported catalyst is an IV isotherm with the high relative pressure [25] and has a H3-type hysteresis loop (P∕P0 > 0.4), as exhibited in Figure 2(d). It demonstrates that there are micropores, and B@Co(II)L has uniform pores of a narrow size. Besides, H3-type hysteresis indicates the most prevalent plate-like particle in slit-shaped pores [23, 24, 25]. Based on the image inserted, it can be confirmed that most pores are distributed in the pore size of 4 nm, and the pore distribution is uniform. Actually, the pore volume is 0.089 cm3∕g and the BET surface area is 59.904 m2∕g, which further illustrates that obtained supported catalyst (B@Co(II)L) has high absorption ability to organic polymers.
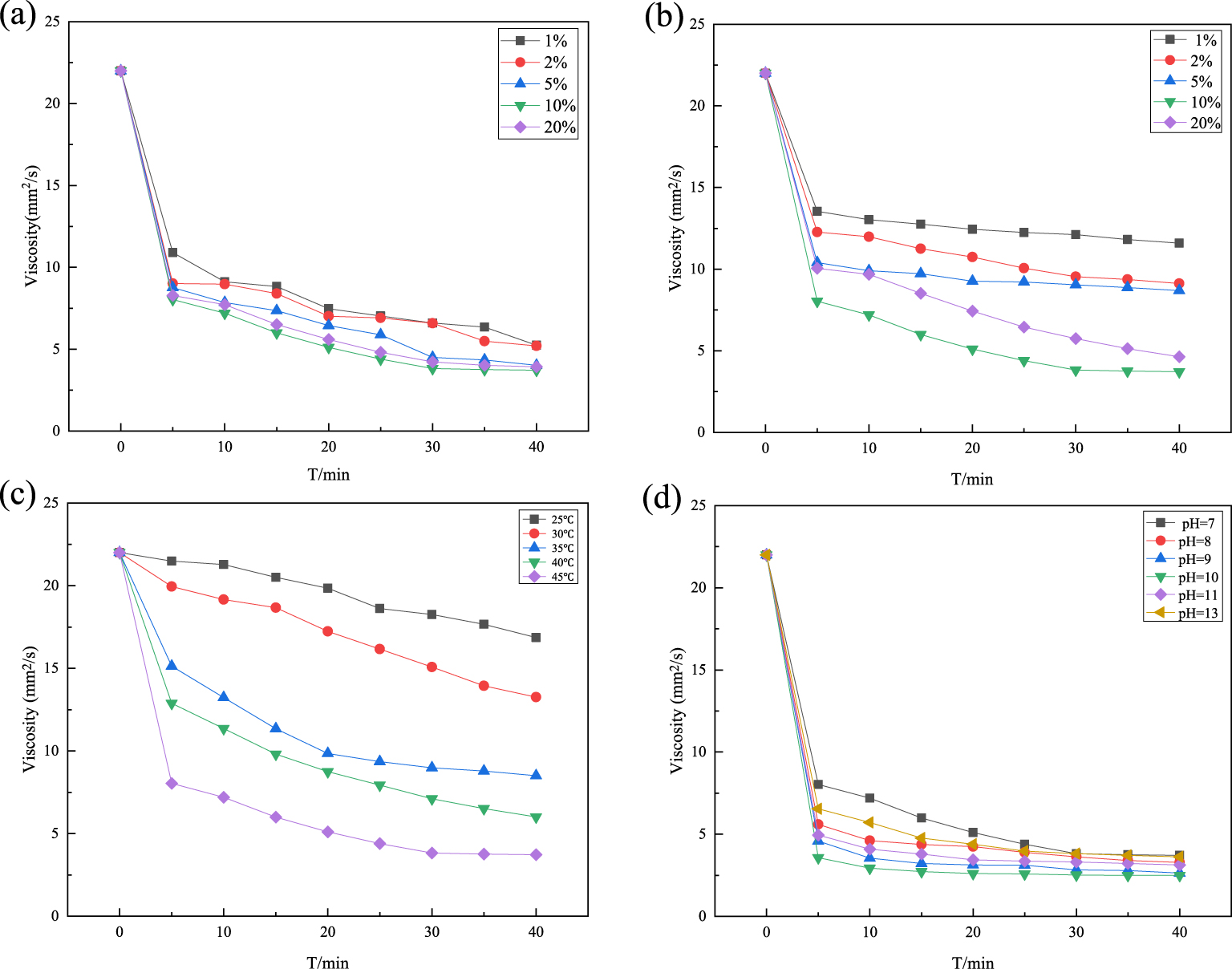
Effect of B@Co(II)L dosage (a), effect of H2O2 concentration (b), effect of reaction temperature (c), and effect of pH (d).
3.2. Optimization of catalytic performance
In order to optimize the catalytic performance of B@Co(II)L, the concentration of the catalyst in reaction was evaluated. Figure 3(a) presents the results of the viscosity of HPGG catalyzed by B@Co(II)L catalysts with different concentrations increased from 1 to 20% (mass ratio to H2O2). The obtained results illustrated that the viscosity of HPGG significantly decreased in the presence of B@Co(II)L when the B@Co(II)L concentration raised from 1 to 10%. However, the HPGG degradation efficiency had no obvious increase when the concentration of catalyst increased to 20%. This is because the amount of active sites for H2O2 decomposition gradually increase as the B@Co(II)L concentration raise; but above 10%, the reactive sites were enough. Therefore, 10% was chosen as the optimum catalyst concentration in the following degradation process.
The concentration of H2O2 as one of the most significant parameters should be highly considered in the Fenton process [19]. So the effect of H2O2 was investigated by varying its concentration from 1 to 20% (mass ratio to HPGG). Results shown in Figure 3(b) indicate that the degradation performance increased with the increasing H2O2 from 1 to 10% due to the effect of many HO∙ radicals produced. However, an evident improvement was not found for a higher concentration (20%). It is because excessive H2O2 reacts with HO∙ competing with organic compounds and consequently reducing treatment efficiency, and hydroxyl radicals scavenging effect resulting in performance dropdown under an excessive hydrogen peroxide according to (1) listed below [22, 23, 24, 25, 19], Therefore, 10% was selected as the optimum H2O2 concentration.
(1) |
The temperature represents a determinant factor in the kinetics of reactions [19]. The influence of reaction temperature on the degradation performance for HPGG was investigated at 25 °C, 30 °C, 35 °C, 40 °C, and 45 °C using B@Co(II)L as the catalyst. The obtained results shown in Figure 3(c) demonstrate that temperature affects the viscosity reduction obviously below 40 °C, while it is not further effective as it is above 40 °C. It is obviously attained that the oxidation degradation is an endothermal reaction and a higher reaction temperature is beneficial to oxidation process on degradation efficiency of HPGG, which is consistent with the results previous studied [19, 26, 27]. Therefore, reaction temperature of 45 °C was chosen as the optimum temperature in the following experiments.
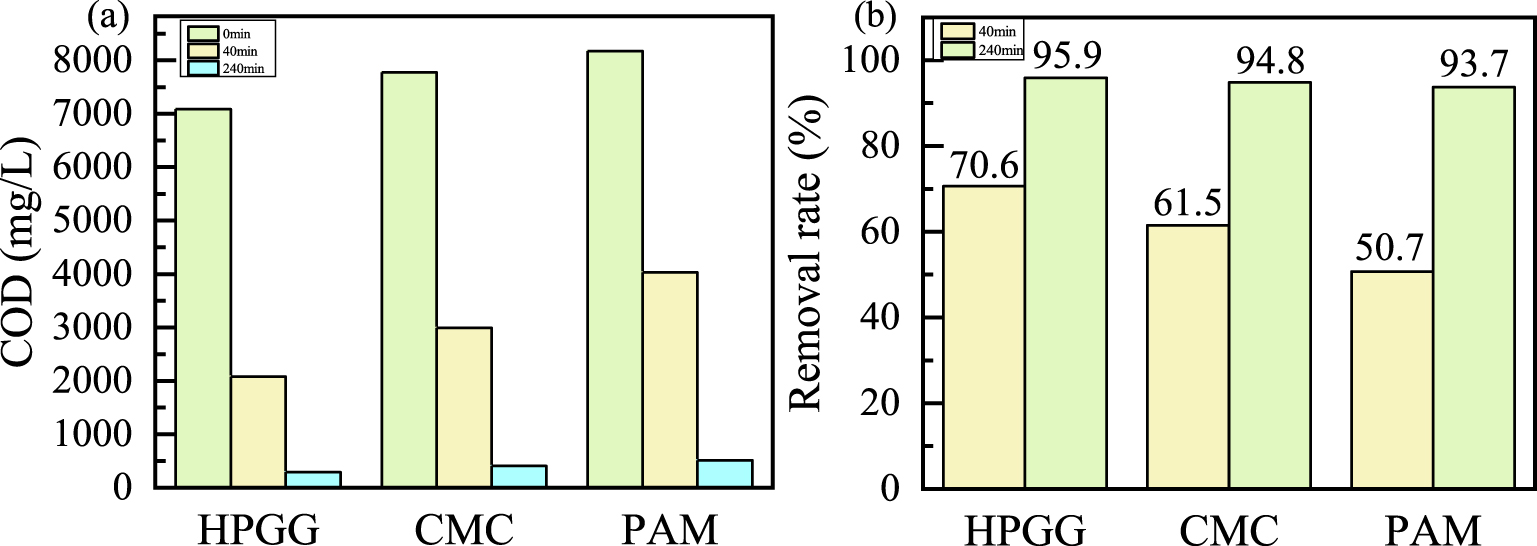
COD degradation of diverse polymers (a), removal rate of diverse reaction time (b).
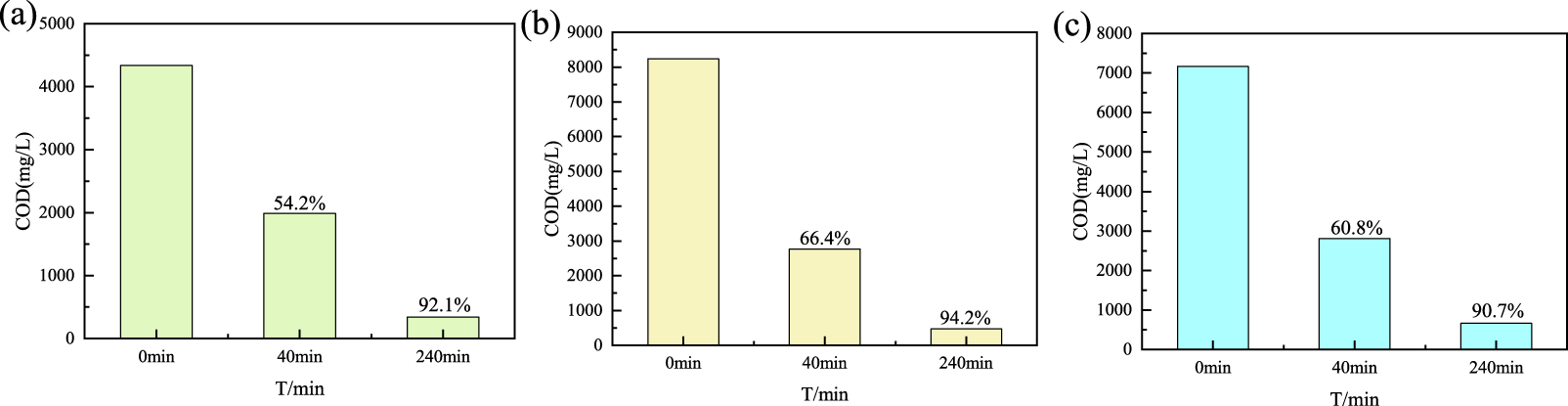
Application in oilfield wastewater (a), application in fracturing fluid (b), application in drilling fluid (c).
Fenton-like process is highly dependent on the solution pH, and the traditional Fenton oxidation with
3.3. COD removal
The chemical oxygen demand is considered a significant factor in evaluating water quality. HPGG, carboxymethyl cellulose (CMC), and polyacrylamide (PAM), as thickeners, are commonly used in oilfields [19, 26, 27]. Therefore, three polymers were used extensively to evaluate the catalytic oxidation performance of catalyst on the COD removal. Three polymers with the mass concentration of 0.6% were respectively oxidized by enough H2O2 at 45 °C and pH of 10, and the results were exhibited in Figures 4(a) and (b). It is obviously found that the COD of three polymers obviously decreased within 40 minutes, and the removal rate respectively reach to 70.6%, 61.5%, and 50.7%. The removal rate increased to 95.9%, 94.8%, and 93.7% within 240 minutes under the same conditions. The results illustrate that B@Co(II)L has high catalytic oxidation performance to multifarious polymers.
3.4. Application in oilfield
In order to evaluate its catalytic oxidation performance, B@Co(II)L was applied to the oilfield wastewater, fracturing fluid, and drilling fluid. The results were shown in Figure 5(a, b, c). As expected, B@Co(II)L performed a great efficiency for the oilfield wastewater, fracturing fluids, and drilling fluid, and the removal rate can be achieved by 92.1%, 94.2%, and 90.7% within 240 minutes. The results illustrate that the catalyst has high oxidation performance in the oilfield applications.
4. Conclusions
In this paper, B@Co(II)L was prepared for catalytic degradation of HPGG so as to achieve the purpose of polymer wastewater treatment under high pH value. It was found that B@Co(II)L performs high catalytic performance for polymer wastewater treatment, and the viscosity of the HPGG can be decreased effectively from 22 to 2.5 with 10% H2O2 (mass ratio to HPGG) and 10% B@Co(II)L (mass ratio to H2O2) at 45 °C. The COD removal rate of HPGG, CMC, and PAM can be significantly increased 95.9%, 94.8%, and 93.7% with enough H2O2 within 240 minutes, and the removal rate of application in the oilfield wastewater, fracturing fluids, and drilling fluid can reach to 92.1%, 94.2%, and 90.7%. The results show that B@Co(II)L can not only broaden the scope of application of the traditional Fenton reagent, but also reduce the difficulty of oilfield wastewater treatment and has a good application prospect in the practical application of oilfield wastewater treatment. This study recommends that the catalyst obtained from this study can be applied for further oilfield application in the treatment of polymer wastewater. In addition, we can also prepare other bentonite supported metal complex catalysts for the treatment of polymers and oilfield wastewater.
Vous devez vous connecter pour continuer.
S'authentifier