1 Introduction
Because of the widespread resistance of many Plasmodium falciparum strains to widely used drugs, malaria's incidence is dramatically increasing [1]. There is therefore an urgent need for efficient drugs with new chemical structure [2, 3]. Combination of drugs is now recommended both to increase the efficiency of the treatment and to impede the development of resistance [4, 5].
Trioxaquines are a new class of antimalarial compounds that we have recently synthesised [6, 7]: they covalently combine a trioxane moiety known to be responsible for artemisinin activity [8–12] and a 4-aminoquinoline entity contained in chloroquine.
Encouraged by the excellent antimalarial activity of the first trioxaquine 1 (DU-1102, Fig. 1) [7, 13], we began a program of structural modulations to find out the structure–activity relationship and to explore for more potent antimalarials [14, 15].
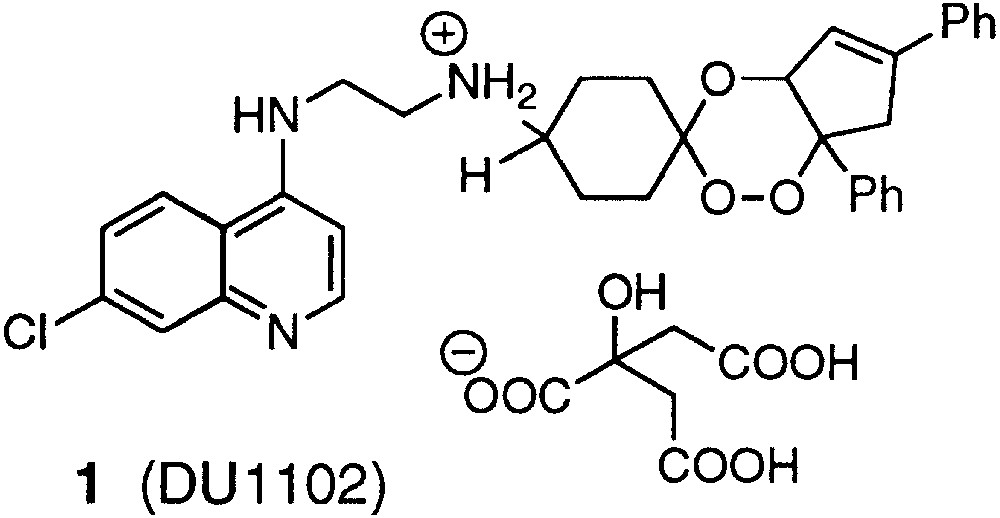
Structure of trioxaquine 1 (DU-1102).
Here we report the convergent synthesis and the biological evaluation of a new trioxaquine named DU-1202 in which the elaboration of the trioxane moiety is done through the halogenocyclisation of a hemiperoxyacetal derived from an allylic hydroperoxide.
2 Results and discussion
2.1 Synthesis of trioxaquine DU-1202
In the synthesis of the first trioxaquine DU-1102, the trioxane moiety was obtained via an initial [4+2] cycloaddition of singlet oxygen with 1,4-diphenyl-1,3-cyclopentadiene, and the trioxane–ketone was finally obtained through the Me3 SiOTf-catalyzed condensation with 1,4-cyclohexanedione [7]. Another major reaction of the singlet oxygen is the ene-reaction with olefins having allylic hydrogen atoms [16]. This reaction has been used for the synthesis of a 1,2,4-trioxane by Bloodworth et al. [17, 18] or by Singh [19]. One of the syntheses proposed by Bloodworth is depicted in Fig. 2 [18]. The ene-reaction of singlet oxygen on 2,3-dimethyl-2-butene 5 leads to the formation of the 2,3-dimethyl-1-buten-3-yl hydroperoxide 6, that is further condensed with an aldehyde to provide the unsaturated hemiperoxyacetal 7. Finally, the electrophile-mediated cyclisation of 7 involving N-iodosuccinimide (NIS) leads to the 1,2,4-trioxane 8.
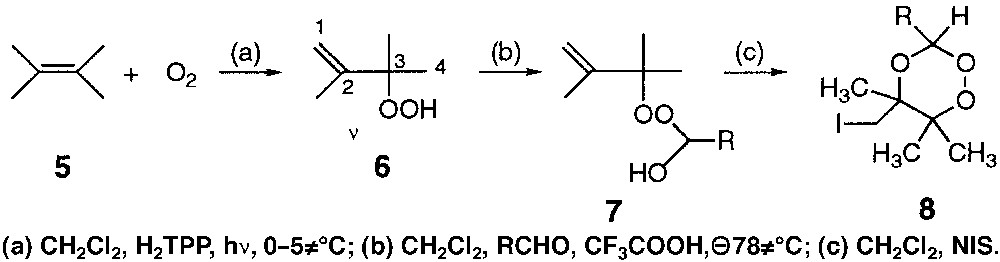
Synthesis of a 1,2,4-trioxane via an initial ene-reaction of singlet oxygen on an olefin.
The covalent link between an aminoquinoline entity and the trioxane 8 could be achieved through a nucleophilic substitution of the iodide by an amine. Attempts to substitute the iodine atom of the trioxane 9 (Fig. 3) prepared from acetaldehyde [18] were unsuccessful under a variety of conditions described in Table 1. We then focused our attention on the use of a functionalised aldehyde that can be engaged in a coupling reaction with an aminoquinoline functionalised by a primary amine 13 (Fig. 4), previously prepared for the trioxaquine DU-1102 [7]. As depicted in Fig. 4, we prepared an oxo-aldehyde to link the trioxane entity to the aminoquinoline by reductive amination [7].
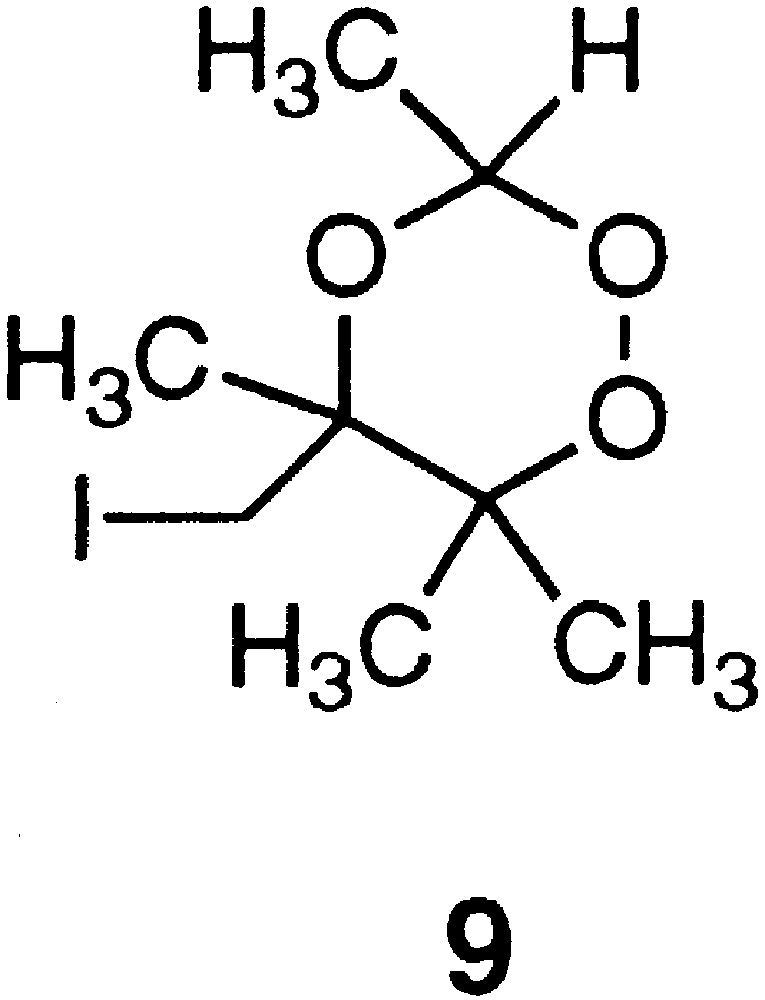
Structure of trioxane 9 prepared from acetaldehyde.
Attempts of nucleophilic substitution of the iodide atom of the trioxane 9
Nucleophile | Solvent, temperature | Additive |
Chloroquine | CH2Cl2, 20 °C | no |
Chloroquine | CH3CN, 80 °C | no |
MeO(CH2)3NH2 | CH3CN, 80 °C | no |
MeO(CH2)3NH2 | CH3CN, 80 °C | CH3COOAg |
MeO(CH2)3NH2 | CH3CN, 80 °C | AgOTf |
HO(CH2)2NHCH3 | CH3CN, 80 °C | no |
Bu4N+OH– | CH3CN, 80 °C | no |

Convergent synthesis of 15 (DU-1202).
The oxo-aldehyde 4-oxopentanal 11 was easily prepared by the pyridinium chlorochromate oxidation of the 5-hydroxypentan-2-one 10 [20]. Then the synthesis of the 1,2,4-trioxane 12 was carried out in a one-pot procedure as described by Bloodworth: the crude hydroperoxide 6 obtained by tetraphenylporphyrin-sensitised photooxygenation of 2,3-dimethyl-2-butene 5 was condensed on the aldehyde 11 using trifluoroacetic acid as a catalyst and the resulting unsaturated hydroperoxyacetal was cyclised with N-iodosuccinimide. The trioxane 12 consists of a pair of diastereoisomeric racemates 12a and 12b, as expected from the presence of chiral centres at C3 and C5 (Fig. 5). The reaction is diastereoselective with the isomer ratios, as determined by NMR, ranging from 4:1 to 5:1 in the different syntheses. By analogy to what Bloodworth described for the trioxane 9, we propose that the major isomer 12a has the alkyl group at C3 and the iodomethyl group at C5 cis to one another, so that they are both equatorial in the favoured conformation, as depicted in Fig. 5 [18]. These two diastereoisomeric racemates were not separated and their mixture was used in the next step.

Structure of trioxanes 12a and 12b prepared from 4-oxopentanal. Compounds 12a and 12b are diastereoisomeric racemates; only one enantiomer of each is depicted.
The reductive amination of the trioxane 12 by the aminoquinoline 13 under previously reported conditions [7] provided the trioxaquine 14. The acyclic ketone function of the trioxane exhibited a poor reactivity in this step. The reductive amination of trioxane 12 was completed in two weeks, whereas that of the trioxane used in the synthesis of DU-1102 1 was completed in 18 h, and the yield was only 30% after purification. All attempts to improve this sluggish reaction failed: heating the reaction mixture (up to 90 °C), adding an acid (acetic acid) or using a stronger reducing agent (NaBH3 CN) led to the formation of by-products or to the degradation of the starting material. The trioxaquine 14 consists a priori in a mixture of eight stereoisomers, as expected from the presence of chiral centres at C3, C5 and C13: at this stage of the study, the separation of the isomers was not performed and the mixture of the stereoisomers was used for the next step.
In the final step, trioxaquine 14 was protonated by citric acid in acetone to provide the trioxaquine citrate 15 (DU-1202). As attested by both elemental analysis and 1H NMR, the monoprotonated trioxaquine was obtained with one additional citric acid molecule (Fig. 4). This feature has already been observed for trioxaquine 1 (DU-1102) [7] and for the trioxaquines prepared from cis-bicyclo[3.3.0]octane-3,7-dione [14].
2.2 Biological evaluation of DU-1202
The trioxaquine citrate 15 (DU-1202, mixture of eight stereoisomers) was tested against three different strains of P. falciparum: a Nigerian chloroquine-sensitive (CQS) strain, FcB1 and FcM29 (CQ-resistant and highly CQ-resistant, respectively). The inhibition concentrations able to reduce the parasitemia by 50% within 48 h (IC50 values) were determined and compared to those of trioxaquine 1 (DU-1102), chloroquine and artemisinine (Table 2).
In vitro antimalarial activity of trioxaquine 15 on three different strains of P. falciparum. IC50 values in nM (SEM, standard error of the mean is given in parentheses). IC50 values are mean values of 2–4 independent experiments.
Trioxaquine | Nigerian CQSa | FcB1–Columbia CQR | FcM29–Cameroon CQR+ |
15 (DU-1202) | 536 (212) | 365 (161) | 188 (27) |
1 (DU-1102) | 22 (1) | 27 (3) | 27 (10) |
Chloroquineb,c | 62 (10) | 116 (57) | 174 (26) |
Artemisininb | 6 (1) | 5 (1) | 8 (1) |
The IC50 values obtained for 15 were ranging from 188 nM on FcM29 (CQR) to 536 nM on the Nigerian strain (CQS). This compound showed a significantly lower antimalarial activity than the first trioxaquine 1, whose IC50 values were ranging from 22 to 27 nM. This loss of activity might be linked to the structural modification around the trioxane moiety. The major difference between the two trioxaquines is in the substitution of the C3 (Fig. 6): in 15, C3 is a secondary carbon, bearing the hydrogen atom of the initial aldehyde RCHO, whereas in 1, C3 is a spiro carbon atom linking the trioxane ring and the cyclohexyl ring. As depicted in Fig. 6, this difference can at least partly account for the differential antimalarial activity.

Activation of the peroxide bond in trioxaquines 1 and 15.
Trioxaquines with a high antimalarial activity are expected to have alkylating properties after activation of the peroxide bond by iron(II)-heme, as it has been established for artemisinin [8–12] or other related trioxanes [21, 22]. In the case of trioxaquine 1, the reductive activation by FeII-heme results in the formation of the alkoxy radical 1a. By subsequent homolysis of either C3–C10 or C3–C14 bond, 1a can promptly rearrange to the sterically unhindered C-centred radical 1b, which can be responsible for the alkylation of the heme and so for the antimalarial activity of 1 (Fig. 6a). In the case of trioxaquine 15, the reductive activation by FeII-heme results in the formation of the alkoxy radical 15a, that can rearrange by β-scission of the C3–C11 bond, giving rise to the potentially alkylating radical 15b. As a competitive reaction, the C3–H bond scission may give rise to H• and 15c, which is devoided of any alkylating properties towards heme (Fig. 6b). Further studies are being done in our laboratory to determine which part the mechanism of reductive activation/alkylation has in the antimalarial activity of the trioxaquines but nevertheless, it seems that the presence of the hydrogen atom in α-position of the endoperoxide, leading to the formation of a non-alkylating compound, resulted in a lower in vitro antimalarial activity.
3 Conclusion
A new trioxaquine 15 (DU-1202) has been prepared through a convergent synthesis exploiting a halogenocylisation route to the 1,2,4-trioxane moiety. The trioxaquine 15 exhibits a lower antimalarial activity than the first trioxaquine 1 on three different strains of P. falciparum, suggesting that the presence of an hydrogen atom in α-position of the endoperoxide is not recommended for a good antimalarial activity of trioxane-containing molecules.
4 Experimental section
4.1 Instrumentation, materials and methods
NMR spectra were recorded on Brucker AM 250 or DPX 300 spectrometers. DCI mass spectra were acquired on a Nermag R10-10H instrument and ES mass spectra on a API 365 Sciex Perkin Elmer instrument. Chromatography columns were done on silica gel 60 ACC Chromagel, 70–200 μm granulometry, SDS, or on neutral alumina, Brockman activity II-III, 63–200 μm granulometry, Merck. Silica plates (60 F254, Merck) were used for thin layer chromatography.
4.2 Synthesis of the trioxane 12
4.2.1 2,3-Dimethyl-1-buten-3-yl hydroperoxide 6
2,3-Dimethyl-2-butene 5 (2.8 g, 34 mmol) in dichloromethane (30 ml) was irradiated by a 600 W visible lamp in the presence of molecular oxygen (1.6 bar) during 6 h at 0–5 °C, using tetraphenylporphyrin (5 mg) as photosensitiser. The hydroperoxide 6 was quantitatively obtained and the crude dichloromethane solution was used in the next step without any further treatment. An aliquot was withdrawn and evaporated to dryness for NMR characterisation.
1H NMR (250 MHz, CDCl3) δ, ppm: 1.34 (s, 6H, H3 C4, H3 C6), 1.79 (dd, 4J = 0.8 Hz and 1.5 Hz, 3H, H3 C5), 4.94 (qd, 4J = 0.8 Hz and 2J = 1.5 Hz, 1H, Hb C1), 4.98 (qd, 4J = 1.5 Hz and 2J = 1.5 Hz, 1H, Ha C1), 7.47 (s, 1H, HOO).
4.2.2 4-Oxopentanal 11
To a stirred solution containing 6.4 g (30 mmol) of pyridinium chlorochromate in 25 ml of dichloromethane was slowly added 2.0 g (20 mmol) of 5-hydroxypropan-2-one 10 at room temperature, and the solution was stirred for 2 h. The mixture was passed through a fritted-disk Büchner funnel using a short pad of silica gel. The residue was washed through the funnel with 100 ml of diethyl ether. The diethyl ether/dichloromethane solution was evaporated under reduced pressure. The expected aldehyde was obtained as a brown liquid (purity 82%, yield in aldehyde 58%, with 4′-oxopentyle-4-oxopentanoate as by-product) and was used in the next step without any further purification.
1H NMR (250 MHz, CDCl3) δ, ppm: 2.14 (s, 3H), 2.70 (unresolved m, 4H), 9.75 (s, 1H).
4.2.3 Trioxane 12
In a flask protected from light by aluminium foil, the hydroperoxide 6 (3.8 g, 33 mmol) and aldehyde 11 (7.0 g, 70 mmol) in dichloromethane (60 ml) were stirred with trifluoroacetic acid (5 drops) for 60 min before adding N-iodosuccinimide (10.0 g, 45 mmol). After 3 h, the reaction mixture was washed with 20 wt% aqueous sodium thiosulphate, then with water. The organic layer was dried, the solvent removed under reduced pressure and the 1,2,4-trioxane 12 isolated by chromatography on alumina gel using hexane/diethyl ether (from 90:10 to 50:50, v/v) as eluent. Two diastereomeric racemates 12a and 12b were obtained and were not separated (molar ratio 12a/12b = 85/15 evaluated by 1H NMR). The characterisation was performed on the mixture, but 1H NMR of each diastereoisomer is described separately for clarity.
MS (DCI/NH3+) m/z (%): 359 (22), 360 (MNH4+, 100), 361 (14), 362 (2)
1H NMR for the diastereoisomer 12a (300 MHz, CDCl3) δ, ppm: 1.08 (s, 3H, H3 C10), 1.49 (d, 5J = 0.9 Hz, 3H, H3 C7), 1.50 (s, 3H, H3 C8), 1.82 (m, 2H, H2 C11), 2.15 (s, 3H, H3 C14), 2.57 (m, 2H, H2 C12), 3.14 (d, 2J = 10.0 Hz, 1H, HC9), 3.33 (dq, 5J = 0.9 Hz and 2J = 10.0 Hz, 1H, HC9), 5.41 (t, 3J = 5.3 Hz, 1H, HC3).
13C NMR for the diastereoisomer 12a (75.4 MHz, CDCl3) δ, ppm: 14.7 (C9), 20.9 (C10), 21.7 (C7), 21.8 (C8), 26.2 (C11), 30.4 (C14), 37.5 (C12), 75.5 (C5), 81.1 (C6), 98.2 (C3H), 207.9 (C13).
1H NMR for the diastereoisomer 12b (300 MHz, CDCl3) δ, ppm: 1.08 (s, 3H, H3 C10), 1.49 (m, 3H, H3 C7), 1.50 (s, 3H, H3 C8), 1.82 (m, 2H, H2 C11), 2.15 (s, 3H, H3 C14), 2.57 (m, 2H, H2 C12), 3.31 (d, 2J = 11.0 Hz, 1H, HC9), 4.09 (dq, 5J = 1.2 Hz and 2J = 11.0 Hz, 1H, HC9), 5.22 (dd, 3J = 4.2 Hz and 6.2 Hz, 1H, HC3).
4.3 Synthesis of the trioxaquine citrate 15
Trioxaquine 14. The trioxane 12 (500 mg, 1.5 mmol) and the aminoquinoline 13 (500 mg, 2.3 mmol) were dissolved in dichloromethane (75 ml) before addition of sodium triacetoxyborohydride (400 mg, 1.9 mmol). The reaction mixture was stirred at room temperature for two weeks and then washed with distilled water. The organic layer was then dried and evaporated under reduced pressure to dryness and the obtained trioxaquine 14 was purified by chromatography on silica gel using ethyl acetate/triethylamine (80:20, v/v) as eluent (yield 31%). Several isomers were obtained and not separated. The characterisation was performed on the mixture but NMR description is that of the major isomer (90%).
1H NMR (300 MHz, CDCl3) δ, ppm: 1.07 (s, 3H, H3 C10), 1.11 (d, 3J = 6.2 Hz, 3H, H3 C14), 1.47 (s, 3H, H3 C7), 1.48 (s, 3H, H3 C8), 1.56 (m, 2H, H2 C12), 1.65 (m, 2H, H2 C11), 1.97 (broad s, 1H, HNC12'), 2.74 (q, 3J = 6.2 Hz, 1H, HC13), 2.99 (m, 2H, H2 C12'), 3.12 (d, 2J = 10.0 Hz, 1H, HC9), 3.30 (m, 3H, H2 C11' and HC9), 5.36 (t, 3J = 5.0 Hz, 1H, HC3), 6.06 (broad s, 1H, HNC4'), 6.35 (d, 3J = 5.4 Hz, 1H, HC3'), 7.36 (dd, 3J = 8.9 Hz and 4J = 2.2 Hz, 1H, HC6'), 7.72 (d, 3J = 8.9 Hz, 1H, HC5'), 7.91 (d, 4J = 2.2 Hz, 1H, HC8'), 8.50 (d, 3J = 5.4 Hz, 1H, HC2').
13C NMR (75.4 MHz, CDCl3) δ, ppm: 14.9 (C9), 20.9 (C10), 21.7 (C7), 21.8 (C8 and C14), 28.8 (C11), 31.1 (C12), 42.8 (C12'), 45.0 (C11'), 52.7(C13H), 75.4 (C5), 81.1 (C6), 99.5 (C3H), 99.6 (C3'H), 117.8 (C10'), 121.9 (C5'H), 125.7 (C6'H), 128.9 (C8'H), 135.2 (C7'), 149.4 (C9'), 150.3 (C4'), 152.3 (C2'H).
MS (DCI/NH3+) m/z (%): 547 (6), 548 (MH+, 100), 549 (29), 550 (37), 551(9).
Trioxaquine citrate 15. The trioxaquine 14 (125 mg, 0.23 mmol) was solubilised in acetone (6 ml) before adding a solution of citric acid (93 mg, 0.48 mmol) in acetone (5 ml). The trioxaquine citrate 15 precipitated spontaneously. After centrifugation, the solid precipitate was washed with diethyl ether and dried under vacuum.
1H NMR (250 MHz, DMSO-d6) δ, ppm: 1.04 (s, 3H, H3 C10), 1.11 (d, 3H, H3 C14), 1.40 (s, 6H, H3 C7 and H3 C8), 1.55 (m, 3H, H2 C11 and HC12), 1.80 (m, 1H, HC12), 2.55 and 2.66 (2 × d, 2J = 15.1 Hz, 2 × 4H, citric acid/citrate), 3.20 (m, 3H, HC13 and H2 C12′), 3.25 (d, 2J = 10.2 Hz, 1H, HC9), 3.54 (dd, J = 10.2 Hz and 1.5 Hz, 1H, HC9), 3.63 (m, 2H, H2 C11′), 5.35 (t, 3J = 4.4 Hz, 1H, HC3), 6.64 (d, 3J = 5.5 Hz, 1H, HC3′), 7.57 (dd, 3J = 8.9 Hz and 4J = 2.0 Hz, 1H, HC6′), 7.85 (d, 4J = 2.0 Hz, 1H, HC8′), 8.24 (d, 3J = 8.9 Hz, 1H, HC5′), 8.50 (d, 3J = 5.5 Hz, 1H, HC2′), 10.5 (br).
Anal. calc. for C34 H47 O17 N3 ClI: C 43.81, H 5.08, N 4.51; found C 43.62, H 4.75, N 4.30.
MS (ESI+) m/z (%): 548 (M+, 100), 549 (30), 550 (45), 551(11).
4.4 Antimalarial activity of the trioxaquine 15
4.4.1 P. falciparum strains and in vitro culture
Three strains of P. falciparum were cultured according to a modified Trager and Jensen method in a 5% CO2 atmosphere at 37 °C [23, 24]. The chloroquine-sensitive strain, Nigerian, and two chloroquine-resistant strains, FcB1-Columbia and FcM29-Cameroon were chosen for this study. The parasites were maintained in vitro in human red blood cells (O±) diluted to 1% hematocrit in RPMI 1640 medium (BioMedia, France) supplemented with 25 mM Hepes and complemented with 5% human AB+ serum (‘Centre de transfusion sanguine', Toulouse, France). Parasite cultures tested were not synchronised in vitro.
4.4.2 In vitro antimalarial activity
The antiplasmodial activity of the trioxaquine 15 was evaluated by the radioactive microdilution method described by Desjardins et al. and modified as follows [25, 26]. Drug dilutions were tested several times in triplicate in 96-well plates (TPP, Switzerland) with non-synchronised P. falciparum cultures at 1% parasitaemia with 1% hematocrit. For each test, the plates of parasite culture were incubated with drugs at decreasing concentrations for 48 h, radioactive [3H]-hypoxanthine (Amersham Pharma Biotech, France) being added to the medium at 24 h after the beginning of incubation. The mother solution of the trioxaquine (5 mg ml–1) was prepared in DMSO (Acros Organics, Belgium), and the following dilutions were done with RPMI 1640. Parasite growth was estimated by [3H]-hypoxanthine incorporation. Concentrations of trioxaquine inhibiting 50% of the parasite growth (IC50 ) were graphically determined by plotting the drug concentration versus percent of parasite growth inhibition curves at 48 h of incubation time [26]. The IC50 value given in the text represents the mean value of 2 to 4 independent experiments. The chloroquine and artemisinin sensitivities of the three strains were routinely tested.