1 Introduction
Electroluminescent (EL) organic and coordination compounds have been the subject of a very active research area for some years because of their various potential applications in advanced materials devoted to the design of light, flat and sometimes soft displays [1,2]. Light-emitting diodes based on evaporated molecules are often multi-layer devices consisting of a hole-injecting layer (HIL), a hole-transporting layer (HTL), an emissive layer, and possibly an electron-transporting layer (ETL) sandwiched between two properly chosen electrodes. These structures can be restricted to one, two or three-layer devices if a molecule has the ability to transport electrons and/or holes and at the same time emit light. In this sense, the development of molecules able to transport either holes and electrons is still a challenge. Among the various EL organic compounds recently described in the literature, silole-based ones have occupied a growing position since Tamao et al. firstly reported a general one-pot synthesis of 2,5-diaryl-3,4-diphenylsiloles, and secondly, discovered their high efficiency in EL devices owing to their high electron-transporting properties [3–5]. This very interesting property originates from a low-lying LUMO, which is the consequence of an effective interaction between the σ*-orbital of the silole silicon atom and the π*-orbital of the butadiene fragment [6]. In the course of their investigations to increase the electron-transporting properties of siloles, and therefore, their efficiency in EL devices, Tamao et al. have found that 2,5-dipyridyl-3,4-diphenylsiloles gave excellent results when used in multi-layer EL devices, showing performances that sometimes exceed the well-known tris(quinolinato)aluminum (Alq) with high durability [7]. Moreover, adding diphenylamino groups to the above mentioned 2,5-dipyridylsiloles, they have been able to remove the HTL to achieve single-layer organic EL devices [8]. The design of other siloles having both hole and electron transporting abilities as well as enhanced luminescent properties is therefore an interesting way to follow. In this perspective, we have designed silole-based molecular systems in which the efficiency of the silole, as the emitting center, may be enhanced by an energy transfer process as previously observed in some polymer-based EL devices [9,10]. Basically, this process usually leads to fluorescence quenching of the shorter wavelength emission chromophore and fluorescence enhancement of longer wavelength emission chromophore.
We report in this communication the synthesis and the use in EL devices of a new diarylsilole having di-2-pyridylaminophenyl as aryl groups. The design of this molecule was motivated by the idea that besides being a potential hole transporter, the di-2-pyridylaminophenyl side-group may also induce the enhancement of the silole efficiency by energy transfer (Fig. 1). Moreover, since the di-2-pyridylaminophenyl side-groups are able to bind metal ions [11], they may exhibit some interesting properties as fluorescent sensor, a subject that will also be treated briefly here.
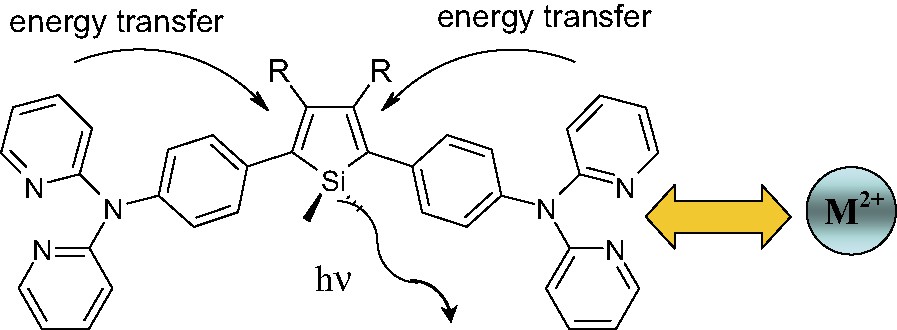
Schematic representation of silole 3 (R = Ph) and of the expected intramolecular energy transfer.
2 Experimental section
2.1 General information and instrumentation
Solvents were distilled prior to use. THF and ether were dried over sodium/benzophenone, and distilled under Argon. All the reactions were carried out under argon atmosphere. 1H, 13C and 29Si NMR spectra were recorded on a Bruker Advance 200 DPX spectrometer, the FT-IR spectra on a Thermo Nicolet Avatar 320 spectrometer, the UV–visible spectra on a Secomam Anthelie instrument and the MS spectra on a Jeol JMS-DX 300 spectrometer. The emission and excitation spectra were performed on a SLM Amenco 8100 spectrometer using degassed 10–5 M solutions, the quantum yields were determined using perilene as standard (Φem = 0.94). I–V characteristics were recorded with a Keithley 2400 Sourcemeter, L–V with a photodiode placed under the OLED and coupled to a HP 3457A multimeter. Electroluminescence spectra were measured using an Ocean Optics PC2000 CCD spectrometer. All EL devices were kept and characterized in a glove box under nitrogen.
2.2 Synthesis of p-2,2′-dipyridylaminobromophenyl (2)
A mixture of 1,4-dibromobenzene (4.939 g, 20.9 mmol), di-2-pyridylamine (1.2 g, 6.96 mmol), K2CO3 (1.12 g, 8.37 mmol) and CuSO4 (0.174 g, 0.697 mmol) in water (20 ml) and CH2Cl2 (100 ml) was stirred well and evaporated to dryness in vacuum. The mixture was ground in a mortar and three to five drops of CH2Cl2 were added to this mixture. The mixture was heated in a sealed tube at 200 °C for 6 h. After being cooled at room temperature, the mixture was dissolved in CH2Cl2 (100 ml) and water (100 ml) and extracted. After evaporation of the solvent, the residue was subjected to column chromatography CH2Cl2 to afford compound 2 (yield: 83%). M.p.: 124 °C. 1H NMR (CDCl3, δ, ppm): 8.35 (dd, J1 = 7 Hz, J2 = 2 Hz, 2H), 7.60 (td, J1 = 7 Hz, J2 = 2 Hz, 2H), 7.51 (d, J = 9 Hz, 2H), 7.09 (d, J = 9 Hz, 2H), 7.05–6.95 (m, 4H). 13C NMR (CDCl3, δ, ppm): 157.32, 149.06, 144.81, 138.11, 133.14, 129.03, 118.93, 118.93, 117.44, 114.31. HRMS (FAB+, m-nitrobenzyl alcohol matrix) m/z: calc. for C16H12N3Br 326.0293, found 326.0271.
2.3 Synthesis of 1,1-dimethyl-2,5-bis(4-phenyl-di-2-pyridylamine)-3,4-diphenylsilole (3)
A mixture of lithium (0.055 g, 8 mmol) and naphthalene (1.03 g, 8 mmol) in THF (15 ml) was stirred at room temperature under argon for 5 h to form a deep green solution of lithiumnaphthalenide. To this mixture was added bis(phenylethynyl)dimethylsilane (1) (0.5 g, 2 mmol) in THF (10 ml). After stirring for 10 min, the reaction mixture was cooled to 0 °C and [ZnCl2(tmen)] (tmen = N,N,N′,N′-tetramethylenediamine) (2.01 g, 8 mmol) was added, followed by an addition of THF (20 ml). After stirring for an hour at room temperature, a solution of 4-(2,2′-dipyridylamino)bromobenzene (2) (1.304 g, 4 mmol) in THF (20 ml) and [PdCl2(PPh3)2] (0.100 g, 0.13 mmol) were successively added. The mixture was heated under reflux and stirred for 20 h. After hydrolysis by water, the mixture was extracted with Et2O. After evaporation of the solvents, the resulting residue was subjected to a column chromatography CH2Cl2/THF (85:15) to give 0.560 g of 3 as a bright yellow crystalline solid (yield: 40%). 1H NMR (CDCl3, δ, ppm): 8.35 (dd, J1 = 7 Hz, J2 = 2 Hz, 4H, B rings: H-1), 7.56 (td, J1 = 7 Hz, J2 = 2 Hz, 4H, B rings: H-3), 7.07–7.02 (m, 6H, A, B and C rings), 6.97–6.95 (m, 20H, A, B and C rings), 0.57 (s, 6H, Si(CH3)2).13C NMR (CDCl3, δ, ppm): 158.54, 154.58, 148.93, 142.85, 139.65, 137.89, 137.03, 130.55, 130.27, 127.93, 126.65, 126.47, 123.49, 118.56, 117.62, –2.72. 29Si NMR (CDCl3, δ, ppm): 8.038. HRMS (FAB+, m-nitrobenzyl alcohol matrix) m/z: calc. for (M+ + 1H) 753.3162 C38H32N6Si, found 753.3196.
3 Results and discussion
3.1 Synthesis
Silole 3 was conveniently prepared by the method described by Tamao et al. that involves the one-pot reductive intramolecular cyclization of bis(phenylethynyl)silane (1) and subsequent Pd(0)-catalyzed cross-coupling reaction with an arylbromide as depicted in Scheme 1. The synthesis of 4-(2,2′-dipyridylamino)bromobenzene (2) that was originaly described by Jia et al. [12] was improved by carrying out the Ullmann's condensation in sealed tubes. Silole 3 was recovered as a bright yellow solid with 24% yield.
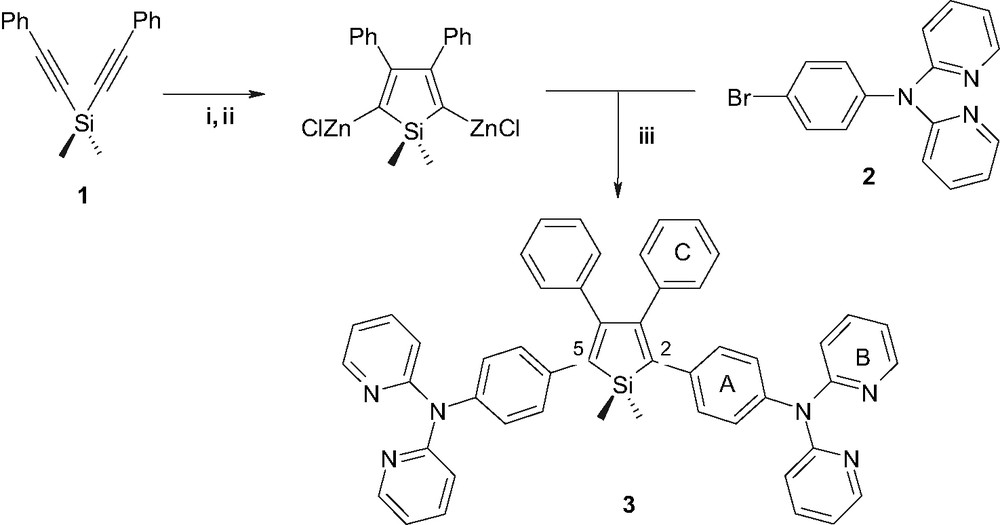
Synthesis of silole 3: (i) NpLi; (ii) ZnCl2·TMEDA; (iii) PdCl2(PPh3)2.
3.2 Photoluminescent properties
In the Fig. 2 are shown the selected UV–visible absorption and fluorescence spectra recorded in solution of compounds 2, 3 and 1,1-dimethyl-2,3,4,5-tetraphenylsilole (4), for comparison. At the first glance, the absorption spectrum of 3 may be described as the superposition of the spectra of its constitutive chromophores; the absorption bands observed at ca. 275 nm originate from π–π* transitions of the silole substituents that is to say, the phenyl groups at the three and four positions and the phenyldipyridylamino groups at the two and five positions, whereas the absorption band observed at 391 nm originates from the π–π* transition of the silole ring [3]. However, when compared with the values reported for the reference compound 4 (absorption: λmax = 359 nm, emission: λmax = 467 nm) [3], the red-shifts that are observed for 3 both in the absorption and emission spectra (absorption: λmax = 391 nm, emission: λmax = 516 nm) are indicative of a substantial perturbation of the HOMO–LUMO levels provided by the presence of the terminal dipyridylamino groups. If we consider now both the emission spectrum of 2 (λex = 290, λmax = 385 nm) and the absorption spectrum of 3, one can anticipate that an intramolecular energy transfer may take place since the dipyridylamine emission greatly overlaps the silole π–π* transition (λmax = 391 nm). As a first consequence of such a process, one of the more striking effect afforded by the presence of the dipyridylamino groups is reflected by a substantial increase of the emission quantum yield values that jumps from 0.0014 for 4 to 0.06 for 3.
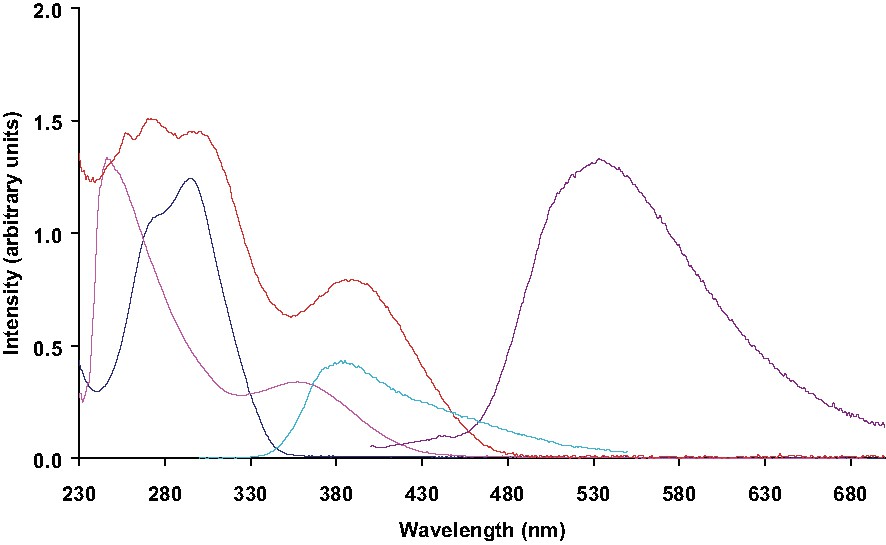
Absorption spectra of compounds 2 (dark blue), 3 (red) and 4 (mauve, left) and emission spectra of compounds 2 (pale blue λex = 290 nm) and 3 (mauve, right λex = 390 nm) measured in dichloromethane.
3.3 Fluorescent chemosensor properties
Preliminary trials have been carried out to estimate the potential of silole 3 as chemosensor for metals ions such as Zn2+ and Cu2+ since their analytical determination is of biological particular interest [13]. Emission spectra (λex = 388 nm) of free silole 3, 3·ZnCl2 and 3·CuCl2 complexes are presented in Fig. 3. 3·ZnCl2 and 3·CuCl2 complexes were prepared by reacting equimolar amounts of 3 and the desired metal ion in a dichloromethane/methanol mixture and isolated as small crystals by layering the resulting solution with hexanes. Chemical analysis of the crystals gave, in each case, a 3/M2+ ratio of 1:1, indicating a polymeric structure for the complexes as described elsewhere [14]. As seen clearly on the curves, the presence of Cu2+ ions in 1:1 ratio yields a total quenching of the fluorescence, whereas Zn2+ ions affect moderately the emission intensity arising from the silole π–π* transition (ca. 25%). The same phenomenon is observed when CuCl2 is added to silole 3 in solution and both selectivity and quenching activities are currently being studied.
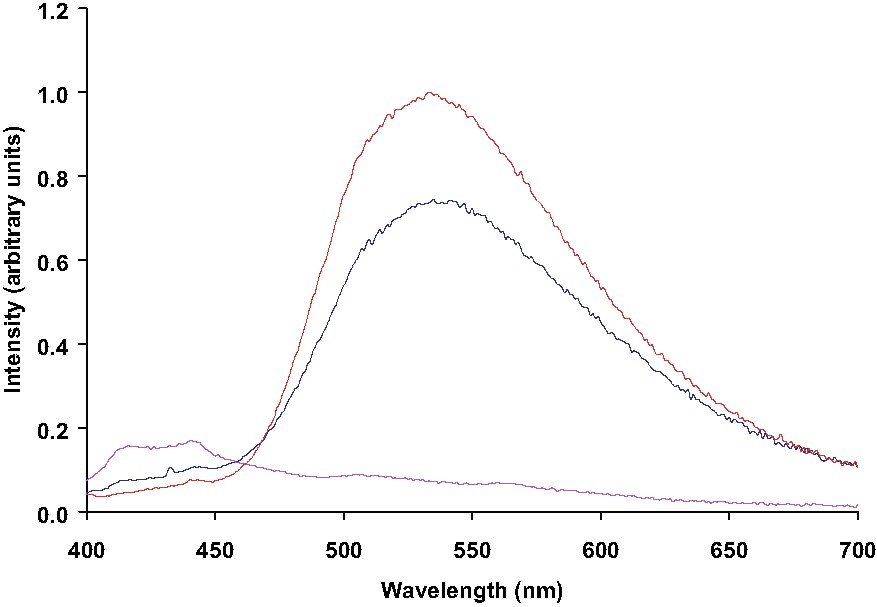
Emission spectra (λex = 388 nm) of free silole 3 (red), 3·ZnCl2 (blue) and 3·CuCl2 (mauve) complexes.
3.4 Electroluminescent properties and EL devices manufacture
Cyclic voltammetry was performed on silole 3 to estimate the HOMO and LUMO energy levels. For efficient electron-transporting materials, a low reduction potential is preferred in order to facilitate electron injection from the cathode. On the contrary, for efficient hole-transporting materials, a low oxidation potential will favor hole injection from the anode. From the onset potentials of the oxidation and reduction processes, the band gap was estimated to be 2.33 eV, which is in good agreement with the values obtained from the optical absorption spectrum (2.48 eV). The HOMO and LUMO energy levels were calculated assuming the energy level corresponding to the electrochemical potential of the Saturated Calomel Electrode (ESCE) is –4.4 eV [15]. The HOMO and LUMO levels were then estimated to be –5.22 and –2.89 eV for silole 3.
EL devices based on silole 3 were fabricated, with the structure shown in Fig. 4. The electrodes are to be chosen in order to match the HOMO and LUMO levels of the organic materials. Indium Tin Oxide (ITO) is a commonly-used anode because it is transparent and has a relatively high work function (4.8 eV), which matches quite well the HOMO level of the siloles. Between ITO and the silole a 60 nm-thick layer of poly(3,4-ethylene-dioxythiophene) doped with poly(styrene sulfonate) (also called PEDOT/PSS) was inserted. PEDOT/PSS is a conducting polymer acting as a buffer in reducing the shorting problems caused by the ITO deposition process and acts as a barrier to the oxygen and indium diffusion from ITO [16]. Moreover it slightly increases the work function of the anode, lowering the barrier to holes. PEDOT was spin-coated on top of ITO and cured at 80° for about 1 h. A 50-nm-thick silole layer was then thermally evaporated under secondary vacuum (around 10–6 mbar). Calcium was used as the cathode as its work function (2.9 eV) is close to the electron affinity of the siloles. Ca was evaporated through a shadow mask on top of the silole and capped with a layer of aluminum to minimize its oxidation. In the ITO/PEDOT/silole/Ca device the silole acts as the emitter but also as the electron and HTL.
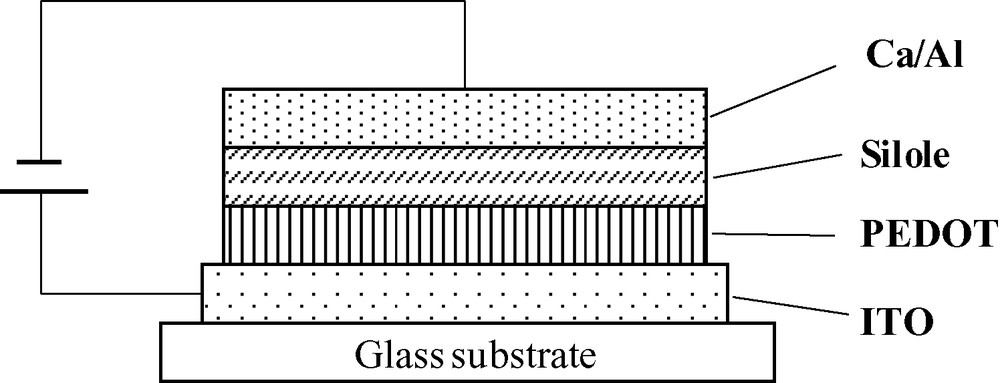
Structure of the EL devices.
The normalized EL spectrum recorded for silole 3 operating in the EL device (Fig. 5) is almost superimposable with the PL spectrum obtained from a thin film of this compound upon an excitation at 400 nm. Both spectra show a similarly featured emission peak in the yellow–green region (λmax = 545 nm) showing that the silole ring is the emissive component in each case.
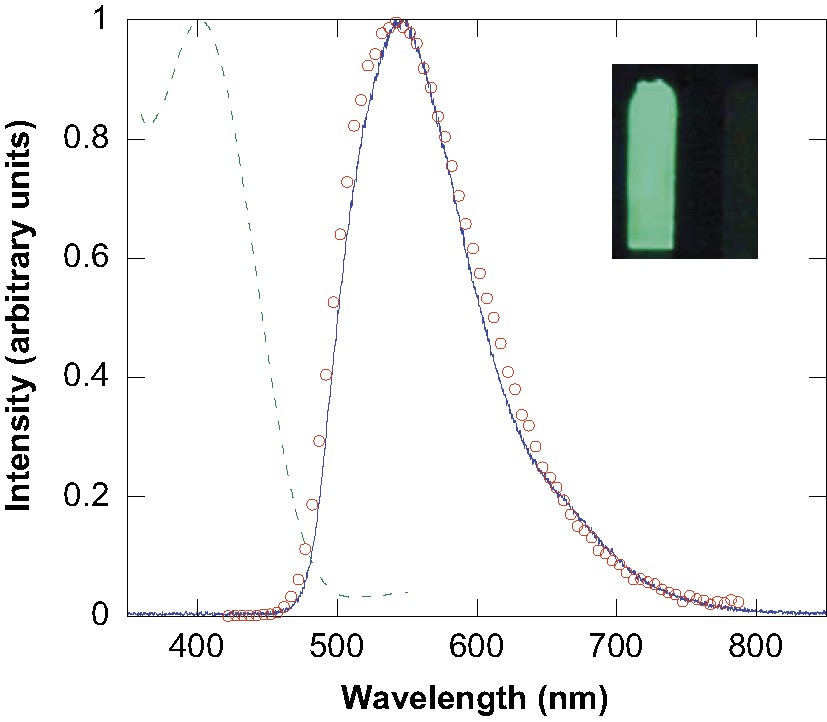
Normalized absorption (dashed line) electroluminescence (plain line) and photoluminescence (circles) spectra of silole 3 as a film or in an ITO/PEDOT/silole/Ca device. Inset: photograph of the EL device operating with silole 3.
Finally, to evaluate the performance of silole 3 as EL materials, we have examined the current density–voltage (J–V) and luminance–voltage (L–V) characteristics of ITO/PEDOT/silole/Ca devices (Fig. 6). Above a threshold voltage of 9 V a significant current flows, giving rise to light emission with excellent performance, reaching a luminance of 104 Cd/m2 at 15, a maximum efficiency of 27 Cd/A and 6 lm/W, and an efficiency of 16 Cd/A and 4 lm/W at 100 Cd/m2. For comparison, this compound is more efficient than Alq, one of the most efficient EL materials reported so far [17,18] which exhibits an efficiency of 2.2 Cd/A under the same conditions.
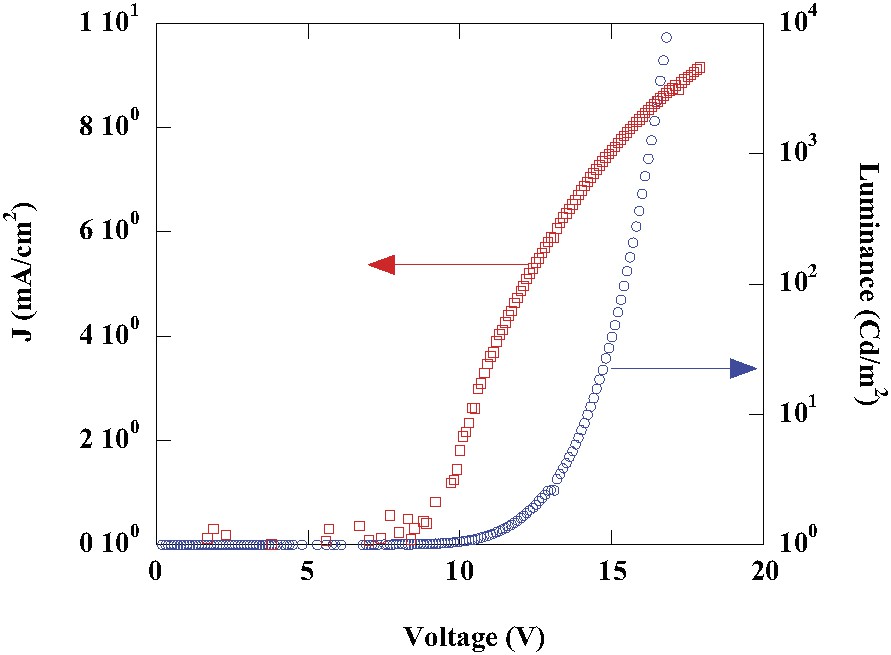
Current density–voltage (J–V, squares) and luminance–voltage (L–V, circles) characteristics of ITO/PEDOT/silole/Ca devices.
In conclusion, we have reported in this communication the synthesis and the use in EL devices of a new diarylsilole having di-2-pyridylaminophenyl as aryl groups. This compound was designed to promote an energy transfer from the aryl side-groups to the central silole ring, and therefore, enhance the luminescence efficiency. According to this assumption, the photoluminescence quantum yield of silole 3 was found to be enhanced by a factor of 40 when compared to the parent tetraphenyl silole 4. This enhancement was also observed when used in single-layer light-emitting diodes with an efficiency of more than 16 Cd/A at 100 Cd/m2, which is firstly more efficient than Alq3, and secondly the best value reported so far in the literature for siloles derivatives. Moreover, we have briefly shown that silole 3 may be used as fluorescent chemosensors, subject that is currently under development.