1 Introduction
In recent years, several soluble alumosiloxanes have been prepared and successfully employed as homogeneous models for alumosilicates and zeolites [1,2]. Suitable starting materials for the synthesis of cyclic alumosiloxanes and - oxides are Ph2Si(OH)2 and (HO)SiPh2OSiPh2(OH), which readily react under self-condensation and/or ring expansion with aluminium alkyls and hydrides to afford mainly eight-membered spirocyclic or polycyclic compounds [3–6].
As we have already reported in detail, the polycyclic alumosiloxane [Ph2SiO]8[Al(O)OH]4·4 Et2O can be obtained by the reaction of diphenylsilanediol with tert-butoxyalane [7] in diethylether as solvent [3]. As could be determined from single crystal X-ray analysis, an Al4(OH)4 eight-membered ring in the centre of the molecular structure is coordinated by four disiloxane semicircles formed by condensation [4,8]. By using the reaction of tert-butoxyalane with (HO)SiPh2OSiPh2(OH), we were able to isolate the bicyclic alumosiloxane Al2[(OPh2Si)2O]3·2 Et2O (3). Any analogy or difference in the reaction behaviour of the compound [Ph2SiO]8[Al(O)OH]4 · 4 Et2O with Lewis bases like water or ammonia leading to various new polycyclic alumosiloxanes like [(Ph2Si)2O3]6(OH)3(Al(OH)6)]·3 Et2O [9] or {(Ph2SiO)12[Al5O7(OH)][Al(OH)2(NH3)2]·Et2O}− [8–10], compared to 3, seemed to us of major interest. In this paper, we present our results concerning the reaction of 3 in different donor solvents in the presence and absence of water.
2 Reaction of the bicyclic compound Al2[(OPh2Si)2O]3·2 Et2O (3) with water in the presence of donor ligands
The starting compound, the bicyclic alumosiloxane Al2[(OPh2Si)2O]3·2 Et2O (3), can easily be obtained in a two-step reaction. In a solvent mixture of acetone and toluene, first the chloro substituents of 1,3-dichloro-1,1,3,3-tetra-phenyl-disiloxane (1) are replaced by hydroxyl groups. This reaction is carried out without direct water contact. To form the hydroxyl groups, sodium hydrogenocarbonate is used. In a salt elimination reaction, sodium chloride and carbon dioxide are released (Eq. (1)).
The pure 1,3-disiloxane 2 may be obtained either in crystalline form by concentration of the mother liquor or as a powder after removing the solvent under reduced pressure.
In the second step, the so formed 1,3-disiloxane is allowed to react with tert-butoxyalane. During a slow dropwise addition of the alane in diethylether, a strong development of hydrogen is observed (Eq. (2)).
The best yields of Al2[(OPh2Si)2O]3·2 Et2O (3) (45%) are found when the reaction is not carried out in stoichiometric amounts as shown in Equation (2) but within double the amount of 2.
In water free solvents, both diethylether molecules coordinating the Lewis-acidic aluminium in Al2[(OPh2Si)2O]3·2 Et2O (3) can easily be substituted by nitrogen containing bases like triethylamine (yield: 76%) (Fig. 1) or pyridine (yield: 48%) (Eq. (3)).
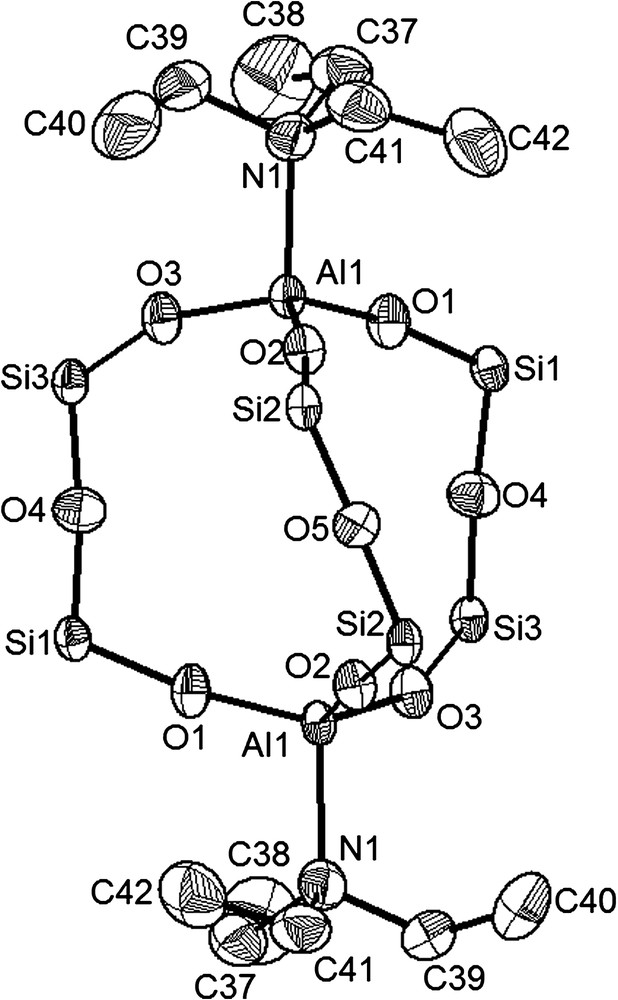
The molecule structure of Al2[(OPh2Si)2O]3·2 NEt3 (4) as determined by X-ray diffraction techniques. Thermal ellipsoids are plotted at the 50% level. Phenyl substituents at silicon and hydrogen atoms are omitted for clarity. Selected bond length [Å] and angles [deg]: Al(1)–O(1) 1.703(2), Al(1)–O(2) 1.700 (2), Al(1)–O(3) 1.706(2), Al(1)–N(1) 2.002(2), Si–O(Al) (av.) 1.597(3), Si–O(Si) (av.) 1.619(2), O(2)–Al(1)–O(1) 114.70(2), O(2)–Al(1)–O(3) 114.03(2), O(1)–Al(1)–O(3) 113.85(2), Si(1)–O(4)–Si(3)′ 173.09(2), Si(2)–O(5)–Si(2)′ 168.47(2), O–Si–O (av.) 111.28(1).
All donor stabilized compounds 3–5 have exact elemental analyses. Recorded CP-MAS NMR spectra show the expected 29Si NMR shifts at −47.4 (4), −48.1 (5) compared to −50.7 ppm for the diethylether coordinated bicyclic 3.
In Fig. 1, the molecular structure of the compound Al2[(OPh2Si)2O]3·2 NEt3 (4) is shown as a representative for the donor adducts of the bicyclic Al2[(OPh2Si)2O]3·2 D (D = Et2O (3),1 NEt3 (4)2 and py (5)3).
The skeleton of Al2[(OPh2Si)2O]3·2 NEt3 (4) consists of a cage which is deduced from a bicyclo[2.2.2]octane in which the two aluminium atoms take up the bridging positions and are connected by three disiloxane semicircles. A similar compound with the lighter homologous boron atoms in the place of the aluminium positions has already been described [11]. As expected, the most significant differences can be found in the coordination sphere of the homologous atoms boron and aluminium. Boron is able to form intramolecular back donations with the neighbouring oxygen atoms, resulting in a trigonal planar BO3 coordination which needs no further base stabilisation despite the trigonal coordination sphere. The aluminium atoms however compensate their electron deficiency by formation of an additional coordination with triethylamine leading to a distorted tetrahedral coordination environment. The O–Al–O angles have an average value of 114.2(1)° and the Al–O bonding lengths are well comparable to literature known values [5,12]. The O–Si–O–Si–O semicircles are strongly twisted. The shorter Si–O distances with an average value of 1.60(1) Å are found in the Al–O–Si groups (Si1–O1, Si2–O2, Si3–O3) whereas the longer ones are located in the Si–O–Si fragments (Si1–O4, Si2–O5, Si3–O4) with 1.619(2) Å on average.
The ethyl groups of the triethylamine donor molecules take a staggered confirmation. Although triethylamine (pKa = 10.8) presents a stronger Lewis base than pyridine (pKa = 5.2) [13], interestingly in Al2[(OPh2Si)2O]3·2 NEt3 (4) the Al1–N1 distances with 2.002(2) Å are longer than in Al2[(OPh2Si)2O]3·2 py (5) with 1.974(4) Å on average.3 This fact can be easily explained by the more sterical hindrance of triethylamine. In 4, the triethylamine groups show more sterical interactions with the phenyl groups at the silicon atoms compared to the plane pyridine molecule in 5, as may be seen from closer inspection of the structures.
An overall view of the reactions of compound Al2[(OPh2Si)2O]3·2 Et2O (3) with water is shown in Scheme 1 which summarizes our findings and proposes an intermediate [(Ph2SiO)2O]2[Al(OH)]2 (6) which could explain the different reaction products. The rearrangement reactions of Al2[(OPh2Si)2O]3·2 Et2O (3) leading to [(Ph2SiO)8(AlO1,5)4·2 THF (7) and [(Ph2SiO)8(AlO(OH))4]·4 Me2CO (8) can most likely be understood by the presence of water. First a molecule disiloxane-1,3-diol is cleaved from 3 and substituted by a hydroxyl group at each aluminium atom. Starting from this intermediate [(Ph2SiO)2O]2[Al(OH)]2 (6), the stoichiometries and structures of the subsequently formed products 7–10 can be easily rationalised. When the reaction of Al2[(OPh2Si)2O]3·2 Et2O (3) is carried out in acetone as solvent, two molecules of 6 dimerise to form the oligoalumosiloxane [(Ph2SiO)8(AlO(OH))4]·4 Me2CO (8) (yield: 33%) (Fig. 2),4 whereas in THF, the polycycle [(Ph2SiO)8(AlO1,5)4]·2 THF (7) (yield: 44%) (Fig. 3)5 is formed through condensation and rearrangement of two entities of 6.
In a recent publication [14] we presented the synthesis and the molecular structure of compound [Ph2SiO]8[AlO1,5]4·2 py showing the same isostructural skeleton as the molecule [(Ph2SiO)8(AlO1,5)4]·2 THF (7) (Fig. 3). With the exception of the different donor atoms, both molecules show comparable bond lengths and bond angles. As shown by single crystal X-ray analysis, 7 presents a polyalumosiloxane of point symmetry i , whose centre is formed of a planar (angle sum 180°) four-membered Al2O2 ring [14], which often can be found as bridging unit between silsesquisiloxanes [15,16]. From a formal point of view, [(Ph2SiO)8(AlO1,5)4]·2 THF (7) can be described as a polycycle, in which four Al2O4Si2 eight-membered rings are connect at two common aluminium corners with the peculiarity that always two rings are fused at a common Al–O edge. As expected, the highest amount of ring strain occurs in the central four-membered Al2O2 ring at the aluminium atoms. Whereas the O1–Al2–O1 angle is 85.59(7)°, the Al2–O1–Al2′ angle has a value of 94.40(1)°. The Al2–O1 and the Al2′–O1 distances of the central Al2O2 ring average to a bond length of 1.804(3) Å. Apparently, there is a strong dilatation in comparison to the Al1–O1 bond in 7 with 1.743(2) Å. The bond length of the donor–acceptor-interaction between aluminium and the oxygen atom of the THF molecule has a value of 1.847(2) Å corresponding to comparable bond lengths of other Al–O interactions [17–19]. The endocyclic oxygen atoms (O1 and O1′) are in the centres of the triangles formed by the aluminium atoms (Al1, Al2 and Al2′) and show an almost trigonal planar coordination sphere (sum of Al–O–Al angles: 359.2(1)°).
In the presence of water, the bicyclic Al2[(OPh2Si)2O]3·2 Et2O (3) surprisingly reacts in acetone as solvent to the pentacyclic molecule [(Ph2SiO)8(AlO(OH))4]·4 Me2CO (8) (Fig. 2). This molecular alumopolysiloxane [(Ph2SiO)8(AlO(OH))4], which forms the centre of the molecule has been synthesized long time ago by the reaction of diphenylsilanediol and tert-butoxyalane in diethylether [3]. The structure of [(Ph2SiO)8(AlO(OH))4] and its reactivity have already been described in detail in former publications [4,8,9,14,20,21]. The single crystal X-ray determination of [(Ph2SiO)8(AlO(OH))4]·4 Me2CO (8) shows that four acetone molecules interact with the hydrogen atoms of the central Al(OH)-groups by formation of hydrogen bridges (Fig. 2). To ethers, amine alcohols and other donors, we have thus added another representative in the form of a ketone [9].
Compound [(Ph2SiO)8(AlO(OH))4]·4 Me2CO (8) has S4 point symmetry in the crystal as has [(Ph2SiO)]8(AlO(OH))4]·4 py [4].
The description of acetone acting as a simple donor may be seen from the carbon–oxygen (C25–O5) distance of 1.214(2) Å which is typical for a CO double bond in the coordinating acetone molecules. As the C–C bonds vary quite remarkably from C25–C26 with 1.508(2) Å to C25–C27 value with 1.474(3) Å, we looked for further verification of our structural description. To find out if the acetone molecules interact through their keto form or through their enol form, IR- and CP-MAS spectra were recorded. Both spectra give evidence of the presence of the acetone molecule in its keto form: in the IR spectrum, the typical keto absorbance occurs at 1689 cm−1. In the recorded 13C-CP-MAS spectrum, the carbon resonances appear at 27.9 ppm for the carbon atoms of the methyl groups and only one signal occurs at 212.7 ppm for the sp2-hybridized C25(O) atom. In the case of a CC double bond, three 13C signals should occur: one in the range of 0–35 ppm for a methyl group and two signals in the range of 80–180 ppm for the methylene group and the quaternary carbon atom, respectively [22].
The CO⋯HO(Al) distances of 2.627(6) Å, which are comparable to CO⋯HO interactions between acetone and alcohols [23–27], indicate relatively strong oxygen–hydrogen bonds [28] leading to similar Al1–O1 and Al1–O1′ distances (1.792(9) and 1.796(9) Å) in the central Al4(OH)4 eight-membered ring of molecule [(Ph2SiO)8(AlO(OH))4]·4 Me2CO (8). According to the point symmetry in the crystal structure, only one 29Si NMR signal occurs in the CP-MAS spectrum at −45.6 ppm, consistent with 29Si shifts in similar alumosiloxane compounds [3,4].
Usually, in order to circumvent the ring strain energy, alumosiloxane chains form at least eight-membered cycles. So the strain energy of six-membered cyclosiloxanes is 16–21 kJ mol−1, while cyclotetrasiloxanes and larger ring systems have almost no strain energy [29,30].
Apart from some halogen substituted alumosiloxanes [31–34], highly strained five- and six-membered cycles with oxygen, aluminium and silicon are only known using di- and trisilane units [6,31,33]. We have now found that the starting compound Al2[(OPh2Si)2O]3·2 Et2O (3) may be transformed to two new and original condensation products in the presence of the base triethylamine. At low temperatures (50--60 °C) in toluene, the dispiro “alumosilicate” [(Ph2SiO)2O]2{Al[(Ph2SiO)2OH·NEt3]}2 (9) (yield: 57%)6 is formed (Fig. 4), whereas at higher temperatures under reflux in toluene, the product [(Ph2SiO)2O]Al[(Ph2SiO)2OH·NEt3] (10) (yield: 77%)7 (Fig. 5) is generated (see Scheme 1).
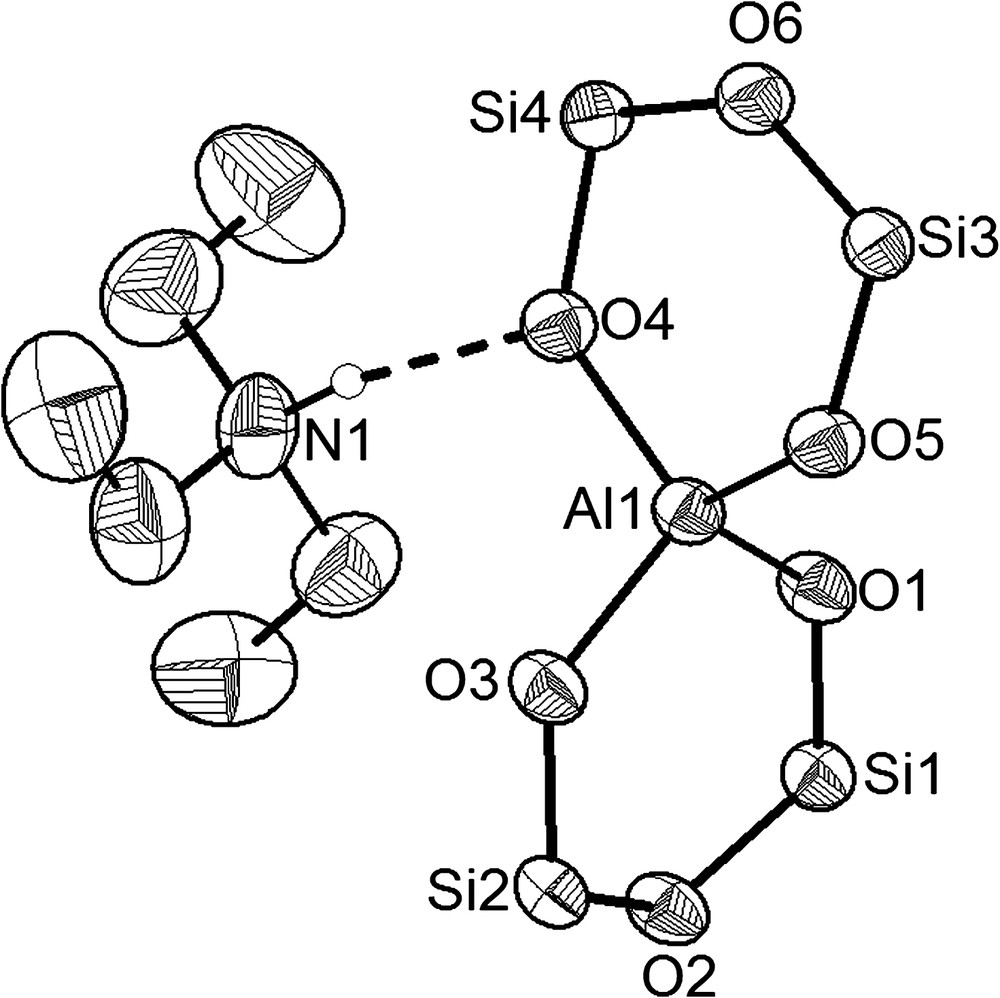
The molecule structure of compound 10, [(Ph2SiO)2O]Al[(Ph2SiO)2OH·NEt3], determined by X-ray diffraction techniques. Thermal ellipsoids are plotted at the 50% level. Phenyl substituents and hydrogen atoms are omitted for clarity. Selected bond length [Å] and angles [deg]: Al(1)–O(1) 1.744(2), Al(1)–O(3) 1.745(2), Al(1)–O(4) 1.773(2), Al(1)–O(5) 1.740(2), Si(4)–O(4) 1.628(2), Si–O(Al) (av.) 1.605(3), Si–O(Si) (av.) 1.641(4), O(1)–Al(1)–O(3) 105.89(2), O(3)–Al(1)–O(4) 109.61(2), O(5)–Al(1)–O(1) 110.89(2), O(5)–Al(1)–O(4) 106.55(2).
The compound [(Ph2SiO)2O]Al[(Ph2SiO)2OH·NEt3] (10) is a spirocyclic “alumosilicate” in which two (Ph2SiO)2O chains are connected by a central aluminium atom. The dihedral angle between the two six-membered rings is almost 90° (88.4(1)°). The O–Al–O angles range from 105.9(1)° (O1–Al1–O3) to 113.2(1)° (O3–Al1–O5) and are comparable to other alumo spiro silicate compounds like [PyH]{Al[OSiPh2(OSiPh2)2O]2} [5] or [HNEt3]{Al[(Cy7Si7O12(SiMe3))]2} [35]. The negative charge of the spirocyclic [(O–SiPh2–O–SiPh2–O)2Al] is compensated by a triethylammonium cation which is linked through a N–H⋯O hydrogen bridge to the oxygen atoms O(4) of the anion (N1⋯O4 = 2.842(1) Å) [5,6,35–37]. The coordination of the ammonium ion is accompanied by a slight elongation of the Al1–O4 (1.773(2) Å) and the Si4–O4 (1.628(2) Å) bonds, compared to the other three Al1–O(1,3,5) (av. 1.743(3) Å) and Si–O(Al) bonds (Si1–O1, Si2–O3, Si3–O5) (av. 1.605(3) Å) [35,36].
The formation of compound [(Ph2SiO)2O]2{Al[(Ph2SiO)2OH·NEt3]}2 (9) can be rationalised by a ring opening reaction of the bicyclic Al2[(OPh2Si)2O]3·2 Et2O (3) and the addition of a further molecule of 1,3-dihydroxy-1,1,3,3-tetra-phenyl-disiloxane in the presence of triethylamine (see Scheme 1). By linking a six-, a twelve- and a six-membered ring through spirocyclic aluminium atoms, a molecule is created as shown in Fig. 4. Whereas the six-membered O(SiO)2Al cycles almost form planar rings (sum of angles: 718.296°), the twelve-membered alumosiloxane ring is not planar (common to higher cyclic siloxane ring systems). As may be seen in Fig. 4, two Si1–O1–Si2 parts of the molecule are almost in a plane to which the O2Al(OSi)2O molecule fragments are arranged above and below. The molecule has a crystallographic inversion centre. The doubly charged anion is compensated by two triethylammonium cations. As found for 10, the formation of an O–H⋯N bridge leads to a significant change in the Al–O (Al–O5 1.788(2) Å, av. 1.731(2) Å) bonds and to an elongation for the Si4–O5(Al) bond length found at 1.626(2) Å, compared to the Si3–O3(Al) bond length with 1.604(2) Å in the six-membered alumosiloxane ring. The second conspicuous fact is the influence of the more electropositive aluminium atom in comparison to the silicon atoms concerning the bond lengths in the six- and the twelve-membered rings. In the six-membered rings, the Si–O(Al) bonds (Si3–O3, Si4–O5) have an average length of 1.615(1) Å while the Si–O bond lengths in the disiloxane chain (Si3–O4, Si4–O4) have 1.642(1) Å on average. The same effect can be observed in the larger ring fragment. Whereas the Si–O(Al) bonds (Si1–O6, Si2–O2) show very short average lengths of 1.596(1) Å, the Si–O bond lengths in the Si1–O1–Si2 groups are significant longer (1.629(1) Å on average) [38].
In both compounds [(Ph2SiO)2O]2{Al[(Ph2SiO)2OH·NEt3]}2 (9) (Fig. 4) and [(Ph2SiO)2O]Al[(Ph2SiO)2OH·NEt3] (10) (Fig. 5), elemental C-, H- and N analyses of which have found to be correct, the recorded 29Si-CP-MAS spectra clearly show differences due to the structural and electronic environments of the respective atoms. Chemical shifts for 29Si-NMR are dependent upon the ring size, as becomes apparent from a deshielding of around 14 ppm being found in the six-membered rings compared to the twelve-membered ring in 9 (−39.3 and −53.6 ppm). In compound 10, the coordination of triethylamine leads, as expected, to a splitting into three 29Si-CP-MAS signals at −35.7, −39.2 and −53.3 ppm.
Acknowledgements
The authors gratefully acknowledge support by the Fonds der Chemischen Industrie as well as by the “Europäisches Graduiertenkolleg” GRK 532 financed by the Deutsche Forschungsgemeinschaft (DFG).
The authors hereby state that there are no conflicts of interest.
1 Al2[(OPh2Si)2O]3·2 Et2O (3), C80H80Si6O11Al2, 1439.94 g/mol, triclinic, Space group P-1, a = 14.511(3) Å, b = 14.494(3) Å, c = 22.233(4) Å, α = 70.815(9)°, β = 71.463(9)°, γ = 60.000(8)°, V = 3756.6(11) Å3, Z = 2, 21 932 reflections, 896 parameters, R = 0.2750 (Rw = 0.5767) (due to the disorder of the molecule in the crystal).
2 Al2[(OPh2Si)2O]3·2 NEt3 (4), C84H90Al2N2O9Si6, 1494.08 g/mol, monoclinic, space group C2/c, a = 25.3630(7) Å, b = 14.5394(3) Å, c = 23.3481(7) Å, β = 114.818(2)°, V = 7814.7(4) Å3, Z = 4, 74 509 reflections, 480 parameters, R = 0.0568 (Rw = 0.1540).
3 Al2[(OPh2Si)2O]3·2 pyr (5), C82H70Al2N2O9Si6·½ C7H8, 1495.97 g/mol, monoclinic, space group C2/c, a = 24.7042(7) Å, b = 14.1960(3) Å, c = 45.1013(11) Å, β = 98.053(3)°, V = 15661.1(7) Å3, Z = 8, 119 128 reflections, 1077 parameters, R1 = 0.0824 (Rw = 0.1645).
4 (Ph2SiO)8(AlO(OH))4·4 Me2CO (8), C108H108Al4O20Si8, 2058.58 g/mol, tetragonal, space group I-4, a = 18.850(3) Å, b = 18.850(3) Å, c = 15.460(4) Å, α = β = γ = 90°, V = 5493.1(19) Å, Z = 2, 52 727 reflections, 332 parameters, R = 0.0265 (Rw = 0.0658).
5 [Ph2SiO]8[AlO1,5]4·4 THF (7), C112H120Al4O18Si8, 2086.72 g/mol, triclinic, space group P-1, a = 13.1656(15) Å, b = 15.1942(17) Å, c = 15.6210(18) Å, α = 69.616(4)°, β = 80.353(5)°, γ = 66.087(4)°, V = 2676.5(5) Å3, Z = 1, 39 619 reflections, 628 parameters, R1 = 0.0639 (Rw = 0.1751).
6 [(Ph2SiO)2O]2{Al[(Ph2SiO)2OH · NEt3]}2 (9), C108H112Si8O12Al2N2, 1908.68 g/mol, triclinic, space group P-1, a = 13.8375(3) Å, b = 16.3265(4) Å, c = 23.4351(5) Å, α = 91.1520(10)°, β = 91.5570(10)°, γ = 107.2190(10)°, V = 5053.0(2) Å, Z = 2, 98 220 reflections, 1203 parameters, R = 0.0446 (Rw = 0.0993).
7 [(Ph2SiO)2O]Al[(Ph2SiO)2OH · NEt3] (10), C54H55AlNO6Si4, 953.35 g/mol, triclinic, space group P-1, a = 14.4359(3) Å, b = 18.7978(4) Å, c = 18.9746(4) Å, α = 90.7510(10)°, β = 90.0050(10)°, γ = 94.9100(10)°, V = 5129.67(19) Å3, Z = 4, 93 905 reflections, 1206 parameters, R = 0.0542 (Rw = 0.1342).