1 Introduction
N-Heterocyclic carbenes (NHCs) were first reported by Wanzlick in the early 1960s [1–5]. During the following decade, Wanzlick, Öfele and Lappert independently reported transition-metal complexes bearing NHCs [6–14]. This new class of ligands rapidly attracted attention [15,16]; however, Wanzlick's quest for an isolated NHC remained unfulfilled. The search for a stable carbene culminated in Arduengo's 1991 disclosure where the isolation and characterisation of 1,3-di(1-adamantyl)imidazol-2-ylidene are described [17]. This revived interest in these ligands that, far from being unstable, were shown to be isolable, tuneable, and capable of stabilising transition-metal complexes. These attractive features prompted numerous groups to study NHCs in transition-metal mediated catalysis. The major advantage of NHCs, so-called “phosphine mimics”, in catalysis, is that an increased stability of the active catalyst is often observed, allowing for lower operational catalyst loadings, prolonged reaction times and tolerance of increased reaction temperature [18–44]. They thus appeared to be ideal candidates for immobilisation protocols in order to combine the advantages of homogeneous with those of heterogeneous catalysis: high activity and selectivity, in conjunction with recyclability. Supported N-heterocyclic carbenes in catalysis have been surveyed by Sommer and Weck, covering developments before 2006 [45]. The present contribution gives an overview on the recent advances reported on covalently immobilised NHC ligands for catalysis, from 2006 to September 2008.
2 NHC catalysts immobilised on polymers
Polymers are amongst the most commonly employed supports to immobilise NHCs. Early work on the subject was directed towards their use for Ru-based metathesis catalysts [45]. Interestingly, the most recent advances in this field have mainly focused on the development of such supported ligands for palladium-catalysed cross-coupling reactions. Luo and co-workers developed the polystyrene-supported NHC–palladium complex 1 shown in Scheme 1 [46].

Polystyrene-supported NHC.
This catalyst can promote the desulfitative Suzuki–Miyaura coupling of arylsulfonyl chlorides and boronic acids, at a Pd loading of 2.5 mol%. The supported catalytic system could be recycled up to 5 times without significant loss of activity. Studies on Pd leaching showed that the resin had released 0.21% of Pd during the first run, 89 ppm during the second and 53 ppm during the fifth run. More recently, the catalytic performance and recyclability of 1 in the Suzuki–Miyaura coupling of arenediazonium salts with boronic acids were investigated [47]. These studies showed that catalysis could be performed under milder reaction conditions (2 mol% Pd, r.t.), and that the catalyst could be reused 10 times. Another advantage of the system is that catalysis was conducted under aerobic conditions. Using a similar approach, Lee and co-workers synthesised the polymer-supported Pd complexes shown in Fig. 1. Synthesis of the polymer-supported imidazolium salts followed by reaction with palladium acetate led to supported Pd–NHC complexes. Complex 2 was used in the copper-free Sonogashira coupling of aryl iodides and bromides with alkynes [48]. High yields of coupling products were obtained with 1 mol% Pd loading. Under these conditions, the polymer-supported catalyst was reused successfully three times. Inductively coupled plasma-atomic emission spectroscopy (ICP–AES) showed no significant decrease in Pd-content on the polymer beads. Complex 3 is a macroporous polystyrene (MPS) supported palladium system that has been shown efficient in the Suzuki–Miyaura coupling of aryl chlorides [49]. Using a loading of 2 mol% Pd, aryl iodides and bromides could be coupled at room temperature, whilst higher temperature and longer reaction times are needed for the activation of aryl chlorides. However, it should be mentioned that this is a rare example of a heterogenised system able to promote the Suzuki–Miyaura coupling of deactivated aryl chlorides. Recycling of the catalyst was studied in the course of 5 runs, with Pd leaching between 1 and 3% observed in each run, and an essentially similar yield of biaryl formed (>89%) from one run to the next.
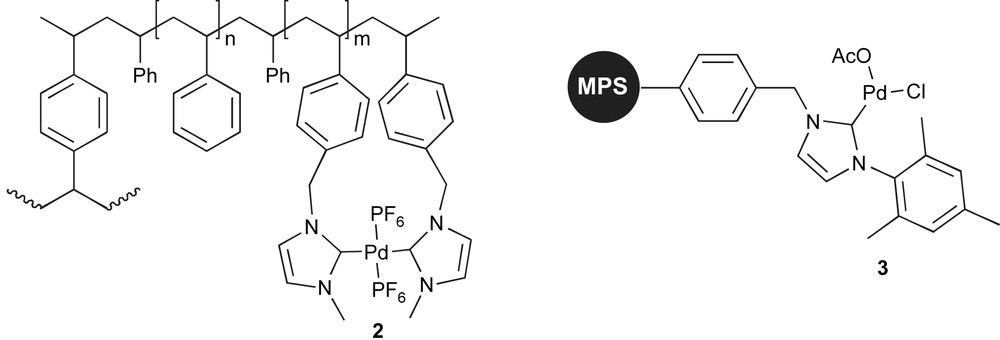
MPS supported palladium–NHC precatalysts.
A different approach for the development of NHC-immobilised complexes consists of using bisimidazoles as monomeric units for the synthesis of polyimidazolium salts. Such salts can then be reacted with palladium sources to form NHC complexes (Scheme 2). Ying and co-workers have claimed the use of these polymeric systems for the Suzuki–Miyaura reaction [50,51].
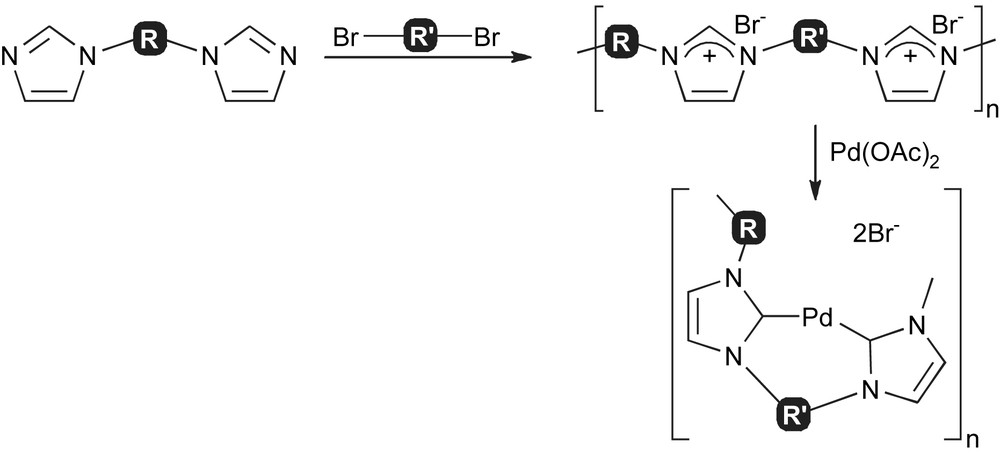
Synthesis of polyimidazolium salts and corresponding palladium complexes.
As mentioned above, there has been a large effort in the immobilisation of ruthenium precatalysts for metathesis reactions. Indeed, the major drawback of these systems is the difficult removal of toxic metallic residues from products, which are often pharmaceutical targets. Grubbs and co-workers reported the poly(ethylene glycol) (PEG) supported catalyst (Fig. 2, complex 4) for ring-opening metathesis polymerisation, ring-closing metathesis and cross-metathesis [52]. The advantage of this system is that the simple aqueous extraction of the PEG-supported catalyst from diethyl ether solutions of products reduces drastically the level of Ru contamination [53].
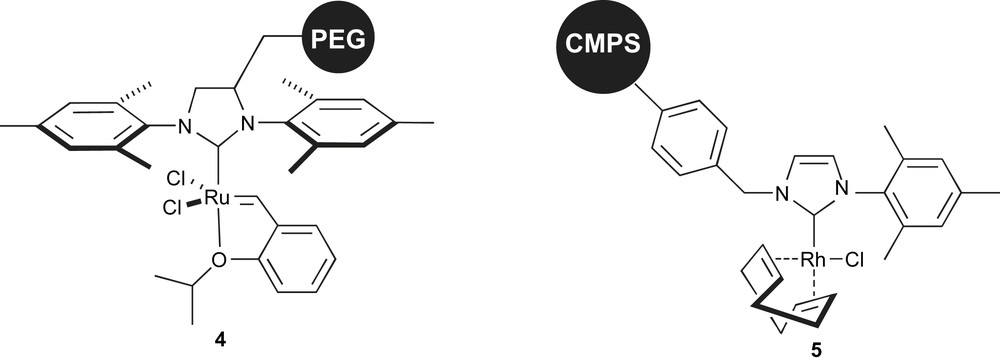
Olefin metathesis and arylation of aldehyde precatalysts.
Luo and co-workers recently disclosed one of the rare examples of a polymer-supported NHC–rhodium complex. This chloromethyl polystyrene (CMPS) immobilised system was used in the addition of arylboronic acids to aldehydes (Fig. 2, complex 5) [54]. This catalyst could be reused up to 5 times, provided that the correct solvent mixture was employed.
The recent reports discussed above show the viability of immobilising catalysts by bonding the N-heterocyclic carbene ligand to a polymeric support. Such an approach has also shown promise with silica-based supports. This is discussed in the following section.
3 NHC catalysts immobilised on silica
Silica has attracted significant attention as a support because covalent binding to its surface is enabled by the presence of OH groups. Indeed, the simple reaction of the ligand or catalyst bearing a trialkoxysilyl group leads to grafting onto the silica wall. Recent literature employing this immobilisation strategy has mainly focused on palladium systems. Artok and co-workers published the synthesis of Pd(II) complexes, shown in Fig. 3, obtained by the reaction of the imidazolinium salts with Ag2O, followed by NHC-transfer to the palladium centre [55]. These grafted complexes were studied in the Mizoroki–Heck reaction of aryl bromides and iodides with styrene and butyl acrylate. In the coupling of 4-iodotoluene, complex 6b was reused for 10 cycles, however, longer reaction times were required after the sixth run. With 4-bromoacetophenone as substrate, the drop in catalytic activity from one run to the next was even more dramatic. In this case, it was shown that catalytically active species had been released into solution, and also that the heterogenised complex decomposed gradually during each cycle. This might be due to the immobilisation of the Pd complexes that might restrict them from adopting stable geometries. High strain in intermediate species might thus result in decomposition. Furthermore, Artok and co-workers showed that the reaction was mainly catalysed by dissolved Pd and not, as one would have hoped, by “supported” Pd.
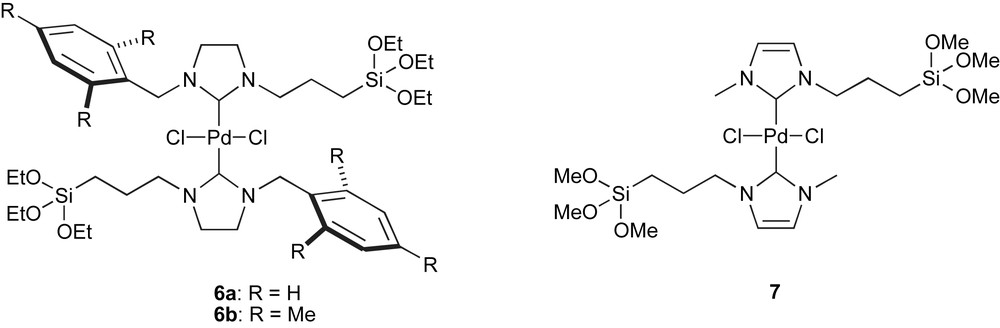
Alkoxysilyl-functionalised NHC–palladium complexes.
A similar complex was reported by Lee and co-workers for the Suzuki–Miyaura coupling (Fig. 3, complex 7) [56]. This complex was reused 4 times in the coupling of bromobenzene with phenylboronic acid at room temperature.
Sen and co-workers chose a different approach that consists of grafting the imidazolium to the silica prior to reaction with palladium (Scheme 3) [57].
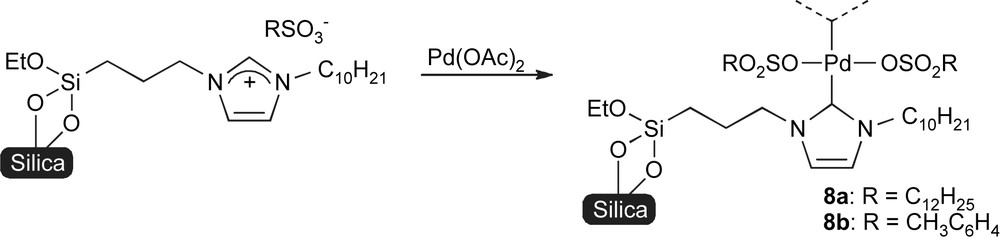
Palladation after NHC anchoring onto support.
These complexes were studied in both the Suzuki–Miyaura and in the Mizoroki–Heck reactions of aryl iodides and bromides. Whilst in the Suzuki–Miyaura coupling 8a and 8b gave similar results, 8b was found more active than 8a for Mizoroki–Heck reactions. Recycling of the catalyst system 8a was achieved over 5 runs for the coupling of 4-iodotoluene with phenylboronic acid.
Recently, Jin and co-workers described the immobilisation of complex 7, using both methods (Scheme 4) [58].
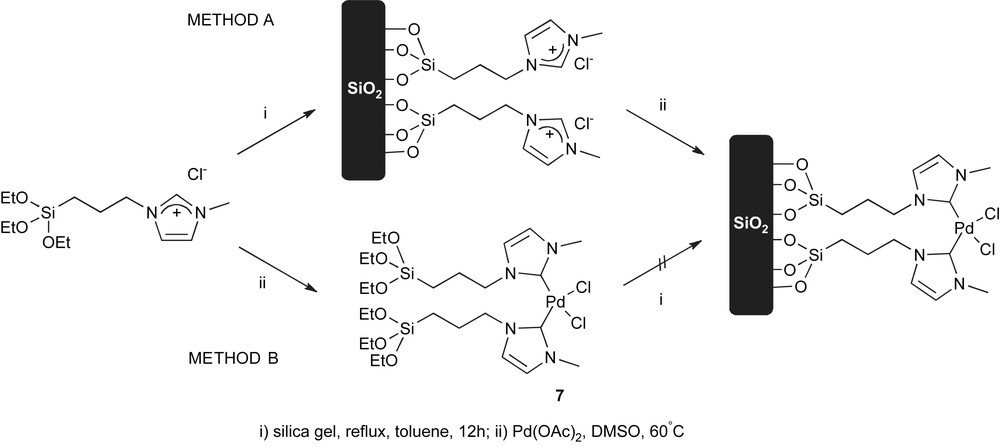
Two approaches leading to anchored NHC–Pd complexes.
Method A was found to be a better strategy because the alkoxysilyl-functionalised complex 7 has a very low solubility in most organic solvents. The immobilised system was investigated in the Suzuki–Miyaura coupling of aryl iodides, bromides and chlorides. The system was shown to be efficient for the coupling of iodides and bromides; and more interestingly, it can also activate aryl chloride substrates. Recycling of the catalyst was shown possible over six runs with both ArI and ArBr substrates, and atomic absorption studies indicated that no Pd leaching had occurred.
Another approach enabling immobilisation of Si(OR)3-functionalised molecules is to subject them to sol–gel processing in order to obtain a silica hybrid material. This was shown to be a viable protocol as the first silica hybrid material incorporating NHC–Pd species (10) could be obtained. Indeed, the hydrolysis polycondensation of complex 9 leads to the formation of amorphous silica hybrid material 10 (Scheme 5) [59,60].
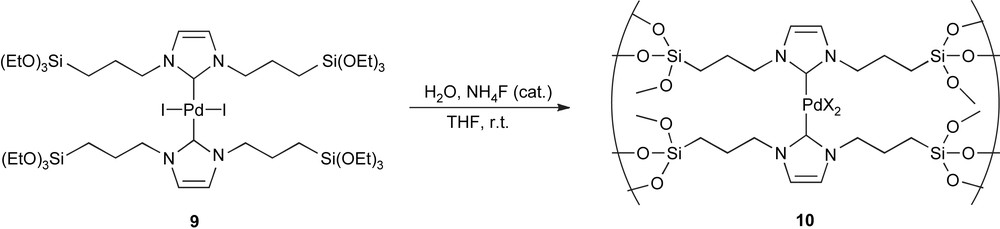
Sol–gel techniques leading to amorphous silica hybrid material.
This hybrid material was used to promote the Mizoroki–Heck and the Suzuki–Miyaura reactions of aryl iodides and bromides. Recyclability of the catalyst was confirmed over 5 runs, without observation of catalyst deterioration. However, catalyst leaching was measured with ICP–AES (22 ppm of palladium was found to be present in the organic fraction of the reaction involving iodobenzene with methyl acrylate) [59]. It was also shown that catalytically active species were released in solution during the Mizoroki–Heck reaction of iodotoluene with methyl acrylate [60]. However, as only a few ppm of Pd was measured in the organic phase, it is possible that the leached palladium, after completion of the reaction, gets redeposited on the support surface.
As mentioned above, another metal that has been intensively studied for heterogeneisation is ruthenium, in particular for its ability to promote metathesis reactions. Thus, Merck has studied SiO2-immobilisation strategies for Ru-metathesis catalysts (Fig. 4). Indeed, N-heterocyclic carbenes bearing a side chain in the 2-position with a terminal alkoxysilyl group were disclosed, together with corresponding second-generation Grubbs-type catalysts [61–64]. More recently, Merck also developed imidazolium and imidazolinium salts bearing the alkoxysilyl group on a chain attached to the 4-position of the heterocycle. This would thus allow for the synthesis of NHC complexes grafted onto supports through the backbone carbon atoms of the carbene ligand [65,66]. In this series of patents, broad claims are made as to the identity R substituents, including but not limited to alkyls, aryls and heterocycles.
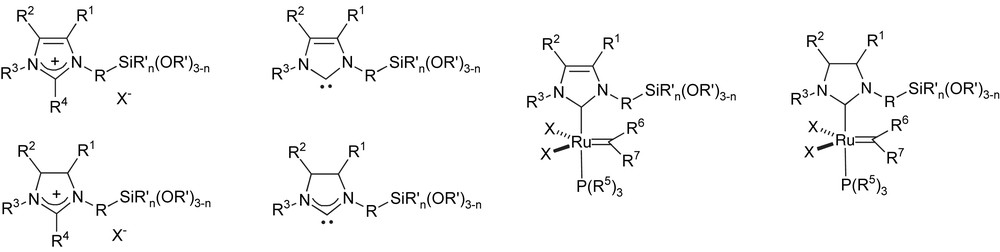
Merck alkoxysilyl-functionalised NHCs.
Gold complexes immobilised through the NHC moiety were recently reported [67]. As shown in Scheme 6, complex 11 was grafted to three different supports: Silica gel, MCM-41 (a mesoporous silica) and ITQ-2 (a delaminated zeolite). These systems were tested for the hydrogenation of alkenes. For the hydrogenation of diethyl citraconate and diethyl benzilydenesuccinate, the heterogenised catalysts of the type 11 had, in general, higher activity than the homogeneous relatives (12 and 13). This could be explained in the homogeneous case, by the poor solubility and self-degradation of the complexes that lead to a decreased reaction rate. In the heterogenised systems, the molecular dispersion can considerably reduce self-degradation. It is also clear that the support has a great influence on the catalyst performance, as the best results were obtained with the complex supported on the zeolite ITQ-2 (11c).
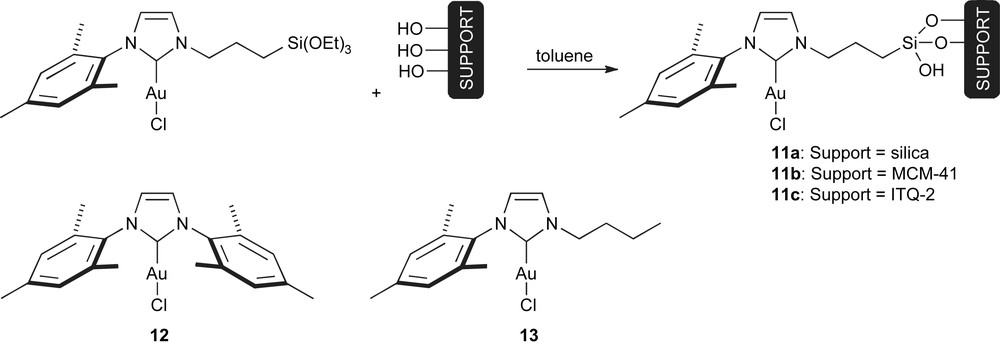
Heterogenised NHC–gold complexes and their homogeneous relatives.
Finally, Corriu, Thieuleux and co-workers recently developed a synthetic strategy leading to a mesostructured material containing Ir–NHC complexes [68]. Their methodology consists in synthesising a nanostructured hybrid material containing benzyl chloride functionalities by co-hydrolysis and co-polycondensation of p-(chloromethyl)phenyltrimethoxysilane with tetraethoxysilane under acidic conditions, and in the presence of a structure-directing agent (Pluronic P123). Reaction of this material with mesitylimidazole leads to the formation of the corresponding imidazolium salt which, after reaction with AgOC(CF3)3, generates complex 14. The latter was then reacted with [{Cp*IrCl2}2] in order to obtain the immobilised iridium complex 15 (Scheme 7).
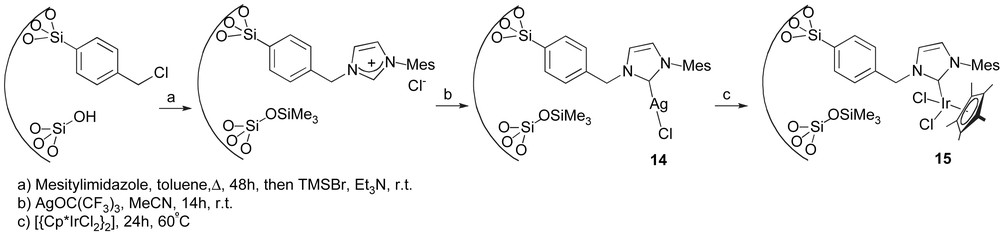
Mesostructured material containing Ir catalyst.
Complex 15 was tested for the deuteration of acetophenone with [D4] methanol. It was shown to be recyclable over three cycles, and that the heterogenised system has a catalytic activity comparable to the one of its homogeneous analogue.
4 Summary and conclusions
NHCs are attractive ligands for immobilisation protocols and thus recycling of catalysts. Indeed, they often increase catalyst longevity and are easily functionalised. Two types of supports are usually encountered: polymeric and inorganic. Latest advances in the use of such immobilised ligands for transition-metal catalysis have mainly focused on palladium. With respect to the supports, when it is polymeric, the most frequently encountered are polystyrene types, the NHC being bound to the polymer through the nitrogen atom. However, Grubbs showed interesting results with a ruthenium complex grafted through the carbon atom on the 4-position to poly(ethylene glycol). Finally, a different approach was shown with polyimidazolium salts leading to polymeric NHC–palladium complexes. With polymeric supports, interesting studies were disclosed mainly using SiO2-supports, the NHC being also covalently immobilised through a nitrogen atom. Merck also developed such ligands and their ruthenium complexes for olefin metathesis chemistry. However, a recent patent application describes the immobilisation of the NHC through its backbone (4-position). This is of great interest as it allows fine-tuning of the NHC sterics, when all systems grafted though nitrogen do not. A gold (I) system has been reported, grafted on silica and on a zeolite. Of great interest in this specific system is the fact that the heterogenised system is more catalytically active than its homogeneous analogue; the opposite is usually the case. Finally, a new strategy was recently developed to synthesise a hybrid mesostructured material containing Ir–NHC. This system showed promising results for H/D exchange.
There is thus a continued great interest in immobilising catalysts through their NHC ligand. Indeed, it has been shown that such systems could allow for catalyst recycling and easy purification of the reaction products. Furthermore, it can improve catalytic activity with respect to homogeneous analogues. However, there is still ample room for improvement: for instance in the areas of catalyst efficiency and longevity. In this respect, design of both new ligands and new materials is necessary.
Acknowledgements
The author is grateful to the DFG (Deutsche Forschungsgemeinschaft) for post-doctoral funding (2003–2004) within the framework of the GRK 532 (European Network for the formation of PhDs “Physical methods for the structural investigation of new materials”), and to Prof. Michael Veith and Dr. Pierre Braunstein for fruitful discussions.